DOI:
10.1039/D2RA00108J
(Paper)
RSC Adv., 2022,
12, 5275-5279
Iterative synthesis of nitrogen-containing polyketide via oxime intermediates†
Received
7th January 2022
, Accepted 6th February 2022
First published on 11th February 2022
Abstract
Typical polyketides consist of C, H, and O atoms, whereas several types of N-containing polyketides are known to show intriguing properties. Because conventional synthetic approaches for such compounds focus on only specific structures, a more general method is desirable. Here, we have developed an iterative synthesis of nitrogen-containing polyketide. Chain elongation of carboxylic acid via decarboxylative Claisen condensation with malonic acid half thioester was iteratively performed to construct carbon frameworks. β-Keto groups formed by the chain elongation were appropriately converted to O-methyl oximes for incorporation of nitrogen atoms. Cyclization of the resulting oxime intermediates followed by reductive N–O cleavage afforded structurally diverse nitrogen-containing polyketides such as 2-pyridone, 4-aminopyrone, and 4-aminosalicylate. This method was finally applied to the synthesis of (R)-6-aminomellein, which is a nitrogen-substituted derivative of bioactive compound, (R)-6-methoxymellein. The versatility of the present method would enable the synthesis of diverse polyketides with nitrogen functional groups, which can be potentially utilized for the development of novel bioactive compounds.
Introduction
Polyketides constitute an important class of secondary metabolites owing to their biological activities and have been utilized for potent pharmaceuticals.1 We have recently developed an iterative method for polyketide synthesis inspired by the biosynthetic mechanism.2 In this method, chain elongation of carboxylic acid via decarboxylative dehydration condensation with malonic acid half thioester (MAHT) is iteratively performed to form polyketide precursors. The advantage of the above strategy is that a variety of structures can be constructed by a tailored method, and chemical modifications such as reduction or alkylation can be easily made to the intended positions.
Similar to the typical natural polyketides, the constituent elements of the products obtained by our method are limited to carbon, hydrogen, and oxygen. Meanwhile, several types of nitrogen-containing polyketides are known in nature.3 For example, 2-pyridone is one of the most notable compounds because of their intriguing and valuable biological properties,4 and the studies on their chemical synthesis has attracted much attention5,6 (Fig. 1a). In addition, the replacement of oxygen functional group in polyketides with nitrogen counterparts can be an effective approach for drug design. Wang and co-workers reported the development of E6201, a novel compound exhibiting anti-inflammatory effect, by replacing methoxy group of natural polyketide with ethylamino group7 (Fig. 1b). From these points of view, the versatile method for the synthesis of nitrogen-containing polyketide is of great value, and we considered that our iterative method has potential to be utilized for such strategy on account of its flexibility.
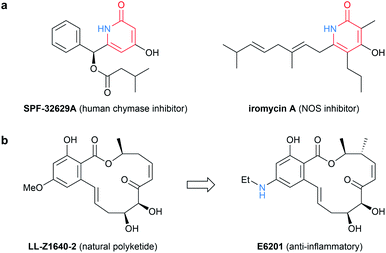 |
| Fig. 1 (a) Natural bioactive 2-pyridone compounds. (b) Replacement of methoxy group in natural polyketide with ethylamino group for the discovery of E6201. | |
The proposed method is shown in Scheme 1. The carbon frameworks are iteratively constructed by the condensation with MAHT 1. The β-keto group formed in each chain elongation is converted to O-methyl oxime (or protected as appropriate). Oxime intermediates thus obtained are cyclized, and the N–O bond is cleaved to afford nitrogen-containing polyketides.
 |
| Scheme 1 Proposed method for the synthesis of nitrogen-containing polyketide. R = alkyl group. | |
Results and discussion
We started from the synthesis of nitrogen-containing triketide compounds to confirm the viability of the proposed method (Scheme 2). Firstly, chain elongation of carboxylic acid 2 with MAHT 1 was carried out according to our previous reports.2a The keto group of the resulting β-ketothioester 3 was subjected to condensation with O-methyl hydroxylamine hydrochloride to give O-methyl oxime 4 in high yield. Hydrolysis of this compound followed by second chain elongation gave δ-methoxyimino-β-ketothioester 6. The second oxymation could be carried out on 6 under the same conditions as the first one to form β,δ-bis(methoxyimino)thioester 7. On the other hand, the β-keto group of 3 was protected as 1,3-dioxolane to afford thioester 8, which was converted to β-ketothioester 10 via carboxylic acid 9 by hydrolysis and chain elongation as reported.2a The oxime formation on 10 gave β-methoxyiminothioester 11. Thus, the series of linear triketides 6, 7, and 11 containing the oxime(s) in different positions were synthesized.
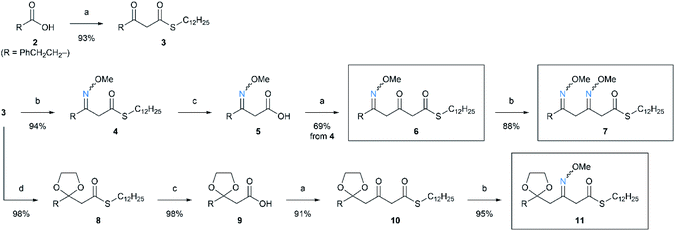 |
| Scheme 2 Synthesis of linear triketide compounds with O-methyl oxime(s): (a) COMU, DIEA, then 1, iPrMgBr, DME, rt; (b) MeONH2·HCl, CH2Cl2/pyridine, rt; (c) CsOH, H2O2, 1,4-dioxane/H2O, rt; (d) TMSO(CH2)2OTMS, TMSOTf, CH2Cl2, 0 °C to rt. | |
Having the linear oxime intermediates in hand, we sought the optimal conditions for cyclization and N–O scission. At first, we treated the precursor 6 with DBU to induce cyclization via acyl substitution of thioester with oxime nitrogen, and subsequent methylation afforded N-methoxy-2-pyridone 12 (Scheme 3). The N–O bond of the product 12 was successfully cleaved by the treatment with samarium(II) iodide8 under mild conditions to give the desired product, 2-pyridone 13.
 |
| Scheme 3 Formation of 2-pyridone structure through cyclization of 6 and reductive N–O bond cleavage: (a) DBU, CH2Cl2, rt, then (MeO)2SO2, DIEA, rt; (b) SmI2, THF, rt. | |
Next, we applied the above cyclization conditions to 7 but no conversion was observed (Table 1, entry 1). Use of stronger base also resulted in no reaction (entry 2). Hence, we tried the cyclization through activation of thioester using thiophilic reagents. When we used NBS,9 the starting material was consumed but complex mixture was obtained without the desired product (entry 3). While Cu(II)10a did not promote the intended reaction (entry 4), Ag(I)10 quickly converted the thioester and afforded the cyclized product with low yield (entry 5). This result seemed to be due to side reactions through ketene formation.10e Fortunately, we eventually found that use of ethyl 2-cyano-2-(hydroxyimino)acetate (oxyma), a recently developed additive for peptide synthesis,11 suppressed the side reactions and gave the desired product in good yield12 (entry 6). Both of the two N–O bonds in 14 were reductively cleaved by RANEY® Ni to generate 4-amino-2-pyridone 15.13 Then, we conducted deprotection of 1,3-dioxolane in 11 for subsequent cyclization. Notably, the oxime moiety was stable under the moderately acidic hydrolysis conditions, and β-methoxyimino-δ-ketothioester 16 was obtained. Subsequently, the same procedure was followed for 7. The cyclization proceeded via an intramolecular transesterification to give 4-methoxyamino-2-pyrone 17 (Scheme 4). Finally, the N–O bond cleavage was done by samarium(II) iodide, furnishing 18. The 4-amino-2-pyrone structure thus obtained has a few examples of existence in natural polyketide compounds.14 These results demonstrated that the structure of products can be diversified by simply changing the position of oxime(s) in linear intermediates.
Table 1 Cyclization of 7 and reductive N–O bond cleavage for the formation of 4-amino-2-pyridone
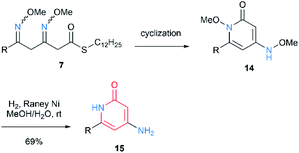
|
Entry |
Cyclization conditionsa |
Yieldb of 14 (%) |
All of the reactions were performed at room temperature. Isolated yield. |
1 |
DBU, CH2Cl2, 8 h |
n.r. |
2 |
KHMDS, CH2Cl2, 8 h |
n.r. |
3 |
NBS, CH2Cl2, 1.5 h |
0 |
4 |
Cu(OTf)2, THF, 2 h |
n.r. |
5 |
CF3CO2Ag, THF, 2 h |
23 |
6 |
CF3CO2Ag, oxyma, THF, 24 h |
74 |
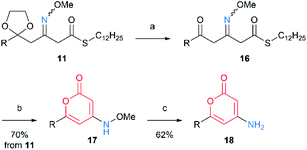 |
| Scheme 4 Formation of 4-amino-2-pyrone structure through cyclization of 11 and reductive N–O bond cleavage: (a) conc. HCl/1,4-dioxane, rt; (b) CF3CO2Ag, oxyma, THF, rt; (c) SmI2, THF, rt. | |
This method was further extended to nitrogen-containing tetraketide compound by performing three chain elongations (Scheme 5). Hydrolysis of thioester 11 and chain elongation of the resulting carboxylic acid 19 gave β-ketothioester 20. Deprotection of 1,3-dioxolane followed by cyclization resulted in the formation of salicylate 21. Remarkably, the cyclization proceeded via an intramolecular aldol condensation in priority to potentially competing 2-pyridone formation by employing the suitable reaction conditions. Samarium(II) iodide was also available in the cleavage of N–O bond in 21, and 4-aminosalicylate 22 was obtained.
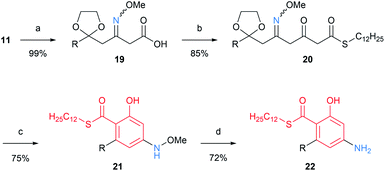 |
| Scheme 5 Chain elongation for the linear tetraketide compounds with O-methyl oxime and the formation of 4-aminosalicylate structure through cyclization of 20 and reductive N–O bond cleavage: (a) CsOH, H2O2, 1,4-dioxane/H2O, rt; (b) COMU, DIEA, then 1, iPrMgBr, DME, rt; (c) conc. HCl/1,4-dioxane, rt, then Et3N, CH2Cl2, rt; (d) SmI2, THF, rt. | |
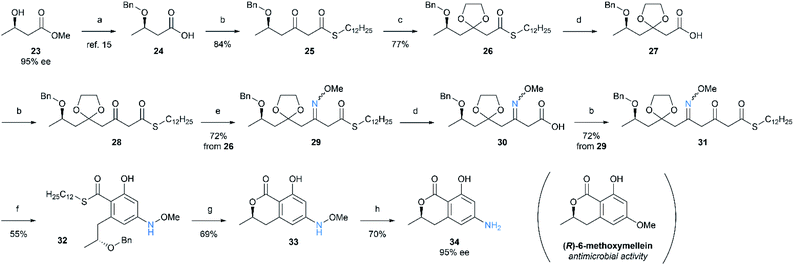 |
| Scheme 6 Synthesis of (R)-6-aminomellein: (a) (i) benzyl 2,2,2-trichloroacetimidate, TfOH, 4 Å molecular sieves, cyclohexane/CH2Cl2, 0 °C to rt; (ii) LiOH, THF/H2O, rt; (b) COMU, DIEA, then 1, iPrMgBr, DME, rt; (c) TMSO(CH2)2OTMS, TBSOTf, CH2Cl2, 0 °C; (d) CsOH, H2O2, 1,4-dioxane/H2O, rt; (e) MeONH2·HCl, CH2Cl2/pyridine, rt; (f) TsOH·H2O, acetone, rt, then Et3N, CH2Cl2, rt; (g) BBr3, CH2Cl2, −78 °C; (h) SmI2, THF, rt. | |
Finally, we applied the present method to the synthesis of amino analogue of a natural polyketide with oxyfunctionality. (R)-6-Methoxymellein is a dihydroisocoumarin compound isolated from carrot cells, and it is known as a phytoalexin which inhibits the growth of several fungi and bacteria.15 We chose this compound as a target molecule for our purpose, and carried out the synthesis of 6-demethoxy-6-amino analogue of the compound (Scheme 6). The synthesis was launched from carboxylic acid 24 prepared from commercially available chiral alcohol 23.16 After chain elongation, the β-keto group of 25 was protected as 1,3-dioxolane. The thioester 26 was hydrolyzed to generate carboxylic acid 27, which was followed by second chain elongation to give 28. Here, the resulting β-keto group was converted to oxime for the O to N replacement giving 29. Carboxylic acid 30 was obtained by hydrolysis of the thioester 29, and subsequent third chain elongation gave a linear precursor 31. Removal of 1,3-dioxolane followed by cyclization yielded salicylate 32. Deprotection of benzyl ether and lactonization proceeded simultaneously by the action of BBr3 to afford lactone 33. Finally, N–O bond cleavage by samarium(II) iodide was carried out to give (R)-6-aminomellein (34). Remarkably, no stereochemical erosion occurred along the synthesis, and the product was obtained with high optical purity.
Conclusions
We have developed the iterative strategy for the synthesis of nitrogen-containing polyketides. Because the chain elongation was performed in the iterative manner inspired by polyketide biosynthesis, the length of carbon chain and the position of O-methyl oxime could be easily changed. Therefore, the products with a variety of structures were obtained by the uniformed protocol. The synthesis of nitrogen-substituted natural compound demonstrated the feasibility of present method for the development of novel bioactive compounds. We believe that this method can be further extended to more complicated compounds derived from longer intermediates by combining with the synthetic method such as convergent strategy.
Conflicts of interest
There are no conflicts to declare.
Acknowledgements
This work was partially supported by a Grant-in-Aid for Scientific Research (C) from Japan Society for the Promotion of Science (17K05854 for K. A.). We thank Dr Abeda Sultana Touchy for carefully proofreading the manuscript.
Notes and references
- Comprehensive Natural Products III, ed. H.-W. Liu and T. P. Begley, Elsevier, Amsterdam, 2020, vol. 1 Search PubMed.
-
(a) K. Akagawa and K. Kudo, Chem. Commun., 2017, 53, 8645 RSC;
(b) K. Akagawa and K. Kudo, J. Org. Chem., 2018, 83, 4279 CrossRef CAS PubMed;
(c) Y. Takeuchi, K. Akagawa and K. Kudo, J. Org. Chem., 2021, 86, 17307 CrossRef CAS PubMed.
-
(a) M. A. Fischbach and C. T. Walsh, Chem. Rev., 2006, 106, 3468 CrossRef CAS PubMed;
(b) D. Boettger and C. Hertweck, ChemBioChem, 2013, 14, 28 CrossRef CAS PubMed;
(c) F. Hemmerling and F. Hahn, Beilstein J. Org. Chem., 2016, 12, 1512 CrossRef CAS PubMed;
(d) A. Miyanaga, F. Kudo and T. Eguchi, Nat. Prod. Rep., 2018, 35, 1185 RSC.
-
(a) T. Shimatani, N. Hosotani, M. Ohnishi, K. Kumagai and I. Saji, J. Antibiot., 2006, 59, 29 CrossRef CAS PubMed;
(b) F. Surup, O. Wagner, J. Von Frieling, M. Schleicher, S. Oess, P. Müller and S. Grond, J. Org. Chem., 2007, 72, 5085 CrossRef CAS PubMed;
(c) M. N. Thaker, M. García, K. Koteva, N. Waglechner, D. Sorensen, R. Medina and G. D. Wright, MedChemComm, 2012, 3, 1020 RSC;
(d) A. Haga, H. Tamoto, M. Ishino, E. Kimura, T. Sugita, K. Kinoshita, K. Takahashi, M. Shiro and K. Koyama, J. Nat. Prod., 2013, 76, 750 CrossRef CAS PubMed;
(e) J. Han, C. Liu, L. Li, H. Zhou, L. Liu, L. Bao, Q. Chen, F. Song, L. Zhang, E. Li, L. Liu, Y. Pei, C. Jin, Y. Xue, W. Yin, Y. Ma and H. Liu, J. Org. Chem., 2017, 82, 11474 CrossRef CAS PubMed;
(f) A. Chicca, R. Berg, H. J. Jessen, N. Marck, F. Schmid, P. Burch, J. Gertsch and K. Gademann, Bioorg. Med. Chem., 2017, 25, 6102 CrossRef CAS PubMed;
(g) W. Y. Zhang, Y. Zhong, Y. Yu, D. F. Shi, H. Y. Huang, X. L. Tang, Y. H. Wang, G. D. Chen, H. P. Zhang, C. L. Liu, D. Hu, H. Gao and X. S. Yao, J. Nat. Prod., 2020, 83, 3338 CrossRef CAS PubMed.
- For the examples shown in Fig. 1, see:
(a) S. R. Vegi, S. K. Boovanahalli, A. P. Sharma and K. Mukkanti, Tetrahedron Lett., 2008, 49, 6297 CrossRef CAS;
(b) H. Shojaei, Z. Li-Böhmer and P. Von Zezschwitz, J. Org. Chem., 2007, 72, 5091 CrossRef CAS PubMed;
(c) S. R. Vegi, S. K. Boovanahalli, B. Patro and K. Mukkanti, Eur. J. Med. Chem., 2011, 46, 1803 CrossRef CAS PubMed.
- Synthetic methods for 2-pyridone structure were reported. For review, see:
(a) H. J. Jessen and K. Gademann, Nat. Prod. Rep., 2010, 27, 1168 RSC;
(b) W. S. Hamama, M. Waly, I. El-Hawary and H. H. Zoorob, Synth. Commun., 2014, 44, 1730 CrossRef CAS;
(c) M. M. K. Amer, M. A. Aziz, W. S. Shehab, M. H. Abdellattif and S. M. Mouneir, J. Saudi Chem. Soc., 2021, 25, 101259 CrossRef CASFor selected papers, see:
(d) S. C. Yu, Y. R. Ka, O. K. Young, Y. H. Joo, J. Hong, H. Shin and S. G. Lee, J. Org. Chem., 2009, 74, 7556 CrossRef PubMed;
(e) B. H. Patel, A. M. Mason and A. G. M. Barrett, Org. Lett., 2011, 13, 5156 CrossRef CAS PubMed;
(f) H. S. P. Rao, N. Muthanna and A. H. Padder, Synlett, 2018, 29, 1649 CrossRef CAS.
- Y. Shen, R. Boivin, N. Yoneda, H. Du, S. Schiller, T. Matsushima, M. Goto, H. Shirota, F. Gusovsky, C. Lemelin, Y. Jiang, Z. Zhang, R. Pelletier, M. Ikemori-Kawada, Y. Kawakami, A. Inoue, M. Schnaderbeck and Y. Wang, Bioorg. Med. Chem. Lett., 2010, 20, 3155 CrossRef CAS PubMed.
- Samarium(II) iodide-mediated reductive cleavage of N–O bonds in O-alkylhydroxylamine and its N-acylated derivatives were reported:
(a) G. E. Keck, S. F. McHardy and T. T. Wager, Tetrahedron Lett., 1995, 36, 7419 CrossRef CAS;
(b) J. L. Chiara, C. Destabel, P. Gallego and J. Marco-Contelles, J. Org. Chem., 1996, 61, 359 CrossRef CAS;
(c) G. E. Keck, T. T. Wager and S. F. McHardy, Tetrahedron, 1999, 55, 11755 CrossRef CAS.
-
(a) T. Kumamoto and T. Mukaiyama, Bull. Chem. Soc. Jpn., 1968, 41, 2111 CrossRef CAS;
(b) H. Minato, H. Kodama, T. Miura and M. Kobayashi, Chem. Lett., 1977, 6, 413 CrossRef.
-
(a) S. Masamune, Y. Hayase, W. Schilling, W. K. Chan and G. S. Bates, J. Am. Chem. Soc., 1977, 99, 6756 CrossRef CAS PubMed;
(b) P. M. Booth, C. M. J. Fox and S. V. Ley, J. Chem. Soc., Perkin Trans. 1, 1987, 121 RSC;
(c) S. V. Ley, S. C. Smith and P. R. Woodward, Tetrahedron, 1992, 48, 1145 CrossRef CAS;
(d) G. Zhou, D. Lim and D. M. Coltart, Org. Lett., 2008, 10, 3809 CrossRef CAS PubMed;
(e) A. E. May and T. R. Hoye, J. Org. Chem., 2010, 75, 6054 CrossRef CAS PubMed.
- R. Subirós-Funosas, R. Prohens, R. Barbas, A. El-Faham and F. Albericio, Chem.–Eur. J., 2009, 15, 9394 CrossRef PubMed.
- We speculate that nucleophilic oxyma effectively traps the ketene species generated from 7 by the action of Ag(I), and the resulting ester is reactive enough to be attacked by the oxime nitrogen, affording the cyclized product 14.
- When samarium(II) iodide was used for this reaction, only one N–O bond was cleaved to give 4-methoxyamino-2-pyridone, and the desired product 15 was not obtained.
- K. M. Jenkins, S. G. Toske, P. R. Jensen and W. Fenical, Phytochemistry, 1998, 49, 2299 CrossRef CAS.
- F. Kurosaki and A. Nishi, Phytochemistry, 1983, 22, 669 CrossRef CAS.
- H. Liu, Y. Zhang, R. Wei, G. Andolina and X. Li, J. Am. Chem. Soc., 2017, 139, 13420 CrossRef CAS PubMed.
Footnote |
† Electronic supplementary information (ESI) available: Experimental procedures, spectral data, HPLC trace and copies of NMR spectra. See DOI: 10.1039/d2ra00108j |
|
This journal is © The Royal Society of Chemistry 2022 |
Click here to see how this site uses Cookies. View our privacy policy here.