DOI:
10.1039/D2RA00077F
(Paper)
RSC Adv., 2022,
12, 8435-8442
Fluorescence detection of malachite green and cations (Cr3+, Fe3+ and Cu2+) by a europium-based coordination polymer†
Received
6th January 2022
, Accepted 9th March 2022
First published on 17th March 2022
Abstract
Due to remarkable fluorescence characteristics, lanthanide coordination polymers (CP) have been widely employed in fluorescence detection, but it is rarely reported that they act as multifunctional luminescent probes dedicated to detecting malachite green (MG) and various metal ions. A europium-based CP fluorescent probe, Eu(PDCA)2(H2O)6 (PDCA = 2,6-pyridinedicarboxylic acid), has been synthesized and exhibited excellent recognition ability for malachite green and metal cations (Cr3+, Fe3+ and Cu2+) among 11 metal cations, 13 anions and six other compounds. The recognition was achieved by fluorescence quenching when MG, Cr3+, Fe3+ and Cu2+ were added to a suspension of Eu(PDCA)2(H2O)6 respectively. Eu(PDCA)2(H2O)6 is a multifunctional luminescent probe, and displayed high quenching efficiencies Ksv (2.10 × 106 M−1 for MG; 1.46 × 105 M−1 for Cr3+; 7.26 × 105 M−1 for Fe3+; 3.64 × 105 M−1 for Cu2+), and low detection limits (MG: 0.039 μM; Cr3+: 0.539 μM; Fe3+: 0.490 μM; Cu2+: 0.654 μM), presenting excellent selectivity and sensitivity, especially for MG. In addition, Eu(PDCA)2(H2O)6 was also made into fluorescent test strips, which can rapidly and effectively examine trace amounts of MG, Cr3+, Fe3+ and Cu2+ in aqueous solutions. This work provides a new perspective for detecting malachite green in fish ponds and heavy metal ions in waste water.
1. Introduction
Malachite green (MG) is a triphenylmethane compound, exists as green crystals and is easily soluble in water. It is an industrial dye and used for dyeing wool, paper and other materials. In the past, malachite green has been utilized as a fungicide in the aquaculture field to control parasites, fungi and bacterial infections in aquatic products.1,2 However, later studies showed that malachite green and its metabolites have obvious residues and toxicity in aquatic animals, which can cause cancer, teratogenicity and mutation.3,4 In the field of pollution-free aquaculture, it has been forbidden in some countries. However, malachite green is still illegally employed in fish farming because of its remarkable antibacterial property and low price.
In order to detect whether MG remains in water of aquaculture, it is necessary to develop a rapid and effective method in detecting MG. The most common method is surface-enhanced Raman scattering (SERS),5–14 but substrate unreliability and impurity interference are major inhibited factors for practical application.15 In recent years, several publications have reported fluorescence detection method for MG, and some results have been achieved.16–19 However, only a few lanthanide CP were used to detect MG.20–22 It is still necessary to develop Ln-CP fluorescent sensors for MG detection with higher selectivity and sensitivity.
Much industrial wastewater discharged from mining, metallurgy, chemical and battery manufacture involves a large amount of toxic heavy metal ions. Heavy metal ion pollution was regarded as severe danger to aquatic organisms, plants, animals, human health and the environment, so it is still a severe task to develop an effective method for detecting heavy metal ions in industrial wastewater. Traditional detection methods for heavy metal ions include ultraviolet spectrometry (UV),23–29 atomic absorption spectrometry (AAS),30,31 fluorescence spectrometry (FS),32–37 and inductively coupled plasma mass spectrometry (ICP-MS).38–41 Among these methods, fluorescence spectrometry has been used widely because it is simple, fast, precise and cost-effective.42,43 It is necessary to design new chemical sensors for the efficient detection of trace metal ions in environmental chemistry.
Due to multiple coordination modes and remarkable fluorescence characteristics,44–53 lanthanide CP (such as Tb-CP, Eu-CP) have been widely employed in fluorescent detection,54–65 such as detecting metal cations,66–75 inorganic anions,69–75 small organic molecules76–80 and explosives.81–83 However, it is rarely recorded that lanthanide CP as multifunctional luminescent probe dedicated to detecting MG and various metal ions.22 In this work, we synthesized an Eu-based CP, Eu(PDCA)2(H2O)6 (referred to as Eu-PDCA, PDCA = 2,6-pyridinedicarboxylic acid), and surveyed its application in fluorescence detection. We detected 11 metal cations, 13 anions, six other compounds, and the results showed that Eu-PDCA can rapidly recognize malachite green and cations (Cr3+, Fe3+ and Cu2+) by fluorescence quenching.
2. Experimental
2.1 Synthesis of Eu(PDCA)2(H2O)6
A solution of distilled water (3 mL) containing Eu(NO3)3·6H2O (0.0267 g, 0.06 mmol) and 2,6-pyridinedicarboxylic acid (0.0201 g, 0.12 mmol) was placed in a Teflon vessel under autogenous pressure and heated at 140 °C for 96 hours, cooled to room temperature over 48 hours. Yellow block crystals were obtained and washed with distilled water and ethanol, dried in air and collected in 61% yield.
2.2 Luminescence sensing experiment
Ethanol solution (50 mL) was added into the ground Eu-PDCA powder (25 mg), performing ultrasonic treatment for four hours. After standing for 12 hours, the colloidal suspension of upper layer was used for fluorescence detection. Malachite green (5 × 10−4 mol L−1 in water solution) and other substances or ions (5 × 10−3 mol L−1 in water solution) were dropped into Eu-PDCA suspension, respectively, then the photoluminescence (PL) spectroscopy was monitored.
3. Results and discussion
3.1 Crystal structure
X-ray crystallographic analysis reveals that Eu(PDCA)2(H2O)6 (PDCA = 2,6-pyridinedicarboxylic acid) crystallizes in monoclinic system, P21/c space group. Eu(III) cation lay in nine coordinated environment, where four oxygen atoms in two carboxylate groups from two 2,6-pyridinedicarboxylic acid ligands, two nitrogen atoms from the same two 2,6-pyridinedicarboxylic acid ligands, one oxygen atom from other 2,6-pyridinedicarboxylic acid ligand, and two oxygen atoms from two water molecules coordinated to same Eu(III) center (Fig. 1a). The four free water molecules lay in the lattice, and they have been omitted for clarity in Fig. 1a. The average Eu–O distance was 2.454 Å, and average Eu–N distance was 2.563 Å. Moreover, Each Eu(III) coordinated unit connected to each other by the bidentate PDCA ligands, resulting a linear chain (Fig. 1b). A three-dimensional structure is achieved by π–π stacking interactions between pyridine rings, as shown in Fig. 1c. The two pyridine rings are stacked in a parallel-displaced geometry with lateral offset. The interplanar distance between the two pyridine rings is 3.231 Å, respectively. The Eu–O bond lengths and bond angles listed in Table S1,† and the crystal data and structural refinement parameters are summarized in Table S2.†
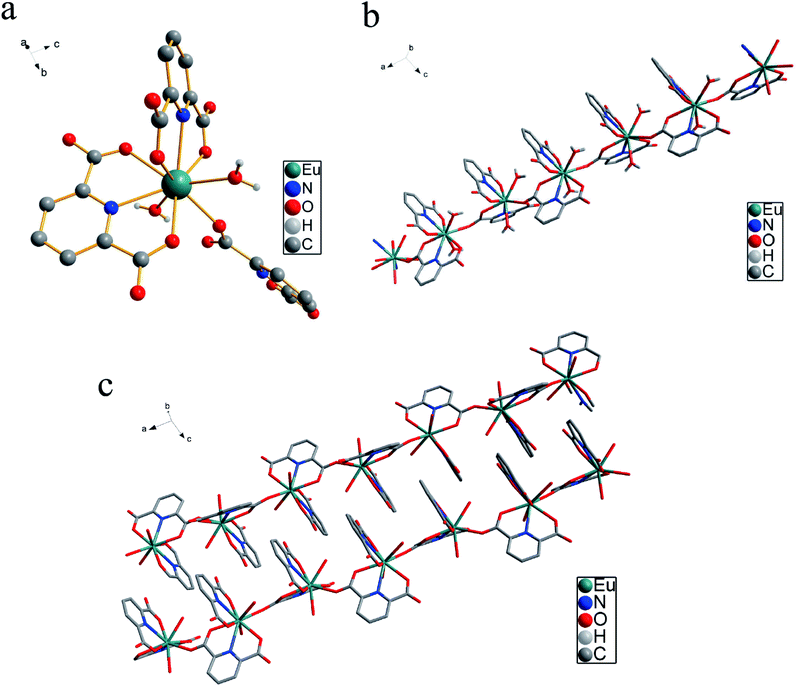 |
| Fig. 1 (a) Coordination mode of Eu(III) cation for Eu-PDCA (four free water molecules were omitted for clarity). (b) A linear chain formed by Eu–O coordinated bonds. (c) 3D structure formed by π–π stacking interactions between pyridine rings. | |
3.2 Thermal stability and PXRD
Thermogravimetric analysis (TGA) and powder X-ray diffraction (PXRD) were measured for the thermal and chemical stability analysis. Thermal gravimetric analysis of Eu-PDCA was shown in Fig. S1.† The weight loss rate of first stage was 18.5% (calcd 18.3%) from room temperature to 118 °C, which was responsible for losing all water molecules. The weight loss rate of 52.1% (calcd 55.9%) happened in second stage after 118 °C, belonging to the loss of coordinated 2,6-pyridinedicarboxylic acid. The powder X-ray diffraction displayed that crystal peaks of Eu-PDCA were consistent with simulated patterns, suggesting the pure crystals of Eu-PDCA (Fig. S2†).
3.3 Luminescence properties of Eu-PDCA
3.3.1 Fluorescent detecting MG by Eu-PDCA. In order to investigate the fluorescence sensing quality of Eu-PDCA, we detected six substances, 11 metal cations and 13 inorganic anions, and the test results of six substances were displayed in Fig. 2.
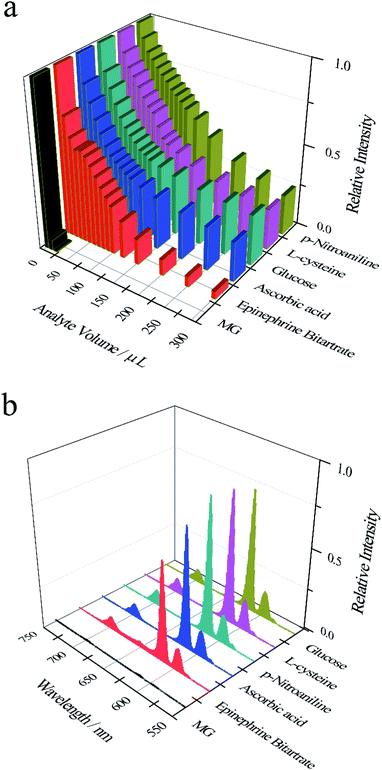 |
| Fig. 2 (a) Bar graph representing the change of the relative emission intensity at 615 nm in the presence of six various analytes. (b) Fluorescence spectra of Eu-PDCA (ethanol suspension, 1.0 mL) added various analytes (20 μL) (excited at 265 nm). | |
As shown in Fig. 2a and S3, among the six substances (malachite green, epinephrine bitartrate, ascorbic acid, glucose, L-cysteine, p-nitroaniline), only adding 20 μL malachite green (5 × 10−4 mol L−1) to Eu-PDCA suspension, the fluorescence intensity of Eu-PDCA was almost reduced to zero, representing complete quenching. However, when 300 μL other substances (5 × 10−3 mol L−1) were added to Eu-PDCA suspension respectively, the fluorescence intensity of Eu-PDCA was not completely quenched. Fig. 2b demonstrating the fluorescence spectra of Eu-PDCA (ethanol suspension, 1.0 mL) added various analytes (20 μL), the result also revealed the 20 μL MG had the best quenching ability with small volume and quick response (in seconds), so Eu-PDCA can effectively detect MG by fluorescence quenching.
The detection of MG is performed by measuring fluorescence spectroscopy of Eu-PDCA before and after adding MG. With the dropping of MG, the fluorescence intensity of Eu-PDCA decreased gradually. When adding 20 μL MG, the fluorescence intensity of Eu-PDCA at 615 nm almost decreased to 0. The titration curves for Eu-PDCA by MG are shown in Fig. 3a. The detection was immediately completed when MG was dropped, only spending several seconds. The result revealed malachite green had evident fluorescent quenching ability for Eu-PDCA. The quenching efficiency Ksv was calculated by the Stern–Volmer (SV) equation. Fig. 3b presenting the Stern–Volmer plots, the Io/I is linearly proportional to MG concentration, and the slope is the Ksv. The Ksv for MG was quantified to be 2.10 × 106 M−1, exhibiting excellent selectivity of Eu-PDCA toward MG. Moreover, the calculated detection limit was 0.039 μM. In addition, in order to detect more conveniently and quickly, fluorescence test strip was used for detecting MG. The test strip was prepared by dipping a filter paper (1 × 3 cm2) in Eu-PDCA ethanol suspension and dried at room temperature. As shown in the left of Fig. 3c, the test strip was white under natural light. For the detection of MG, the test strip was dipped in MG aqueous solution for 1 min and exposed to air for drying. Seeing the right of Fig. 3c, the test strip was greenblack after immersed in MG observed under natural light. In the left of Fig. 3d, under the irradiation of UV light of 365 nm, the test strip without MG was pink. While the pink fluorescence of the test paper disappeared after soaked in MG aqueous solution (in the right of Fig. 3d). To sum up, Eu-PDCA presented fast-responsive luminescent recognition for MG with excellent selectivity and sensitivity.
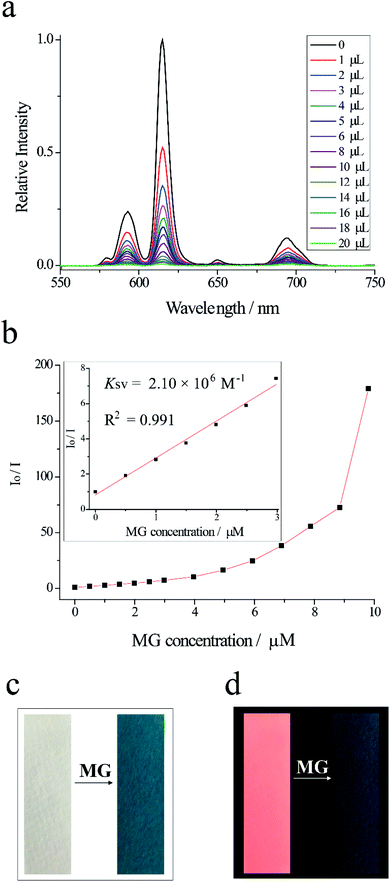 |
| Fig. 3 (a) Fluorescence spectra of Eu-PDCA (ethanol suspension, 1.0 mL) added MG solution (5 × 10−4 mol L−1) (excited at 265 nm). (b) SV curve for MG. (c) Optical image of Eu-PDCA test paper after immersed in MG solution under natural light. (d) Optical image of the Eu-PDCA test paper after immersed in MG solution under 365 nm ultraviolet light. | |
3.3.2 Fluorescent detecting cations by Eu-PDCA. In Fig. 4a and S4, among 11 metal cations, Cr3+ (30 μL), Fe3+ (40 μL) and Cu2+(50 μL) can completely quench the fluorescence of Eu-PDCA. While quench ability for Eu-PDCA by other cations (Cd2+, Zn2+, Al3+, Ag+, K+, Ca2+) was weak, and their quenching ability gradually decreased. Cd2+ (200 μL) can quench the 97.2% fluorescence intensity of Eu-PDCA, and Zn2+ (300 μL) can quench 96.9% fluorescence intensity of Eu-PDCA. In addition, when Ba2+ or Mg2+ ions are added dropwise, fluorescence enhancement occurs. Ba2+ (130 μL) can enhance the relative fluorescence intensity of Eu-PDCA from 1 to 1.973, and Mg2+ (100 μL) enhance the relative fluorescence intensity from 1 to 1.957. But beyond this volume, the fluorescence intensity will decrease slightly. Fig. 4b presenting the fluorescence spectra of Eu-PDCA (ethanol suspension, 1.0 mL) added 40 μL metal cations, when 40 μL Cr3+, Fe3+ and Cu2+ was added, the fluorescence intensity at 615 nm of Eu-PDCA almost reduced to 0, indicating Cr3+, Fe3+ and Cu2+ having excellent quench ability for Eu-PDCA.
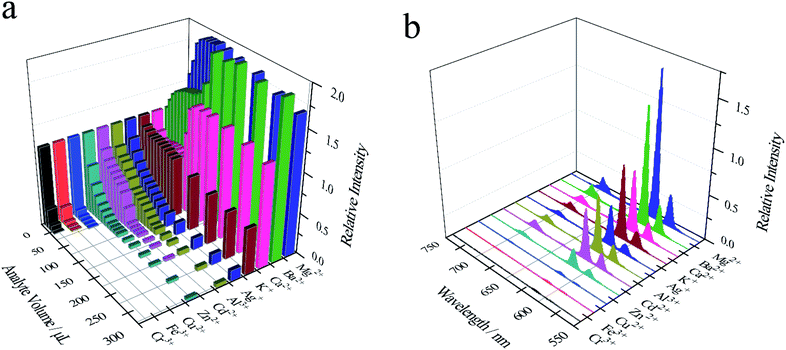 |
| Fig. 4 (a) Bar graph representing the change of the relative emission intensity at 615 nm in the presence of 11 cations. (b) Fluorescence spectra of Eu-PDCA (ethanol suspension, 1.0 mL) added various cations (40 μL) (excited at 265 nm). | |
As shown in Fig. 5a, d and g, Cr3+ (30 μL), Fe3+ (40 μL) and Cu2+(50 μL) can decrease the relative fluorescence intensity of Eu-PDCA nearly to 0. And calculated Ksv for Cr3+, Fe3+ and Cu2+ was 1.46 × 105 M−1, 7.26 × 105 M−1, 3.64 × 105 M−1, respectively. Moreover, the detection limit was about 0.539 μM for Cr3+, 0.490 μM for Fe3+ and 0.654 μM for Cu2+. In addition, fluorescence test paper was also prepared for detecting Cr3+, Fe3+ and Cu2+ cations. As shown in Fig. 5b, e and h, the pictures of the test strips before and after soaking in cations solution show almost no change, except that the test strips soaked in iron ions are slightly yellow observed in natural light. Under 365 nm ultraviolet lamp, it was found that the pink fluorescence of the test strips after soaked in solution disappeared (Fig. 5c, f and i). Eu-PDCA can detect Cr3+, Fe3+ and Cu2+ by fluorescence quenching, and fluorescence test strips can help detect Cr3+, Fe3+ and Cu2+ quickly.
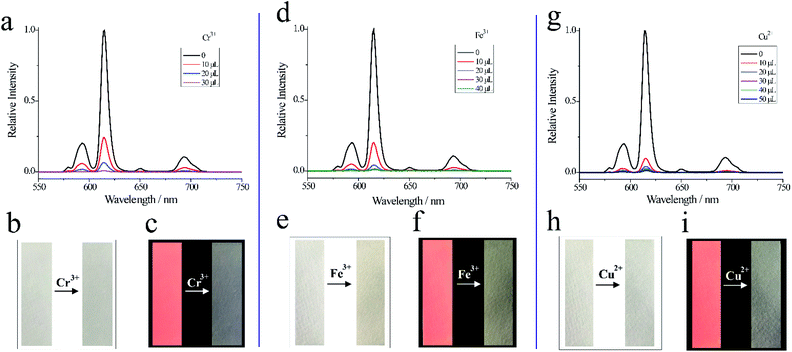 |
| Fig. 5 (a), (d) and (g) Fluorescence spectra of Eu-PDCA (ethanol suspension, 1.0 mL) added metal ions (5 × 10−3 mol L−1) (excited at 265 nm). (a) Cr3+, (d) Fe3+, (g) Cu2+. Optical image of Eu-PDCA test paper after immersed in solutions under natural light, (b) Cr3+, (e) Fe3+, (h) Cu2+. Optical image of Eu-PDCA test paper after immersed in solutions under 365 nm ultraviolet light, (c) Cr3+, (f) Fe3+, (i) Cu2+. | |
3.3.3 Fluorescent detecting anions by Eu-PDCA. In Fig. 6a, 6c and S5, among 13 anions, MnO4− (30 μL) can completely quench fluorescence of Eu-PDCA, CrO42− (300 μL) can quench the 90.6% fluorescence intensity of Eu-PDCA, and ClO4− (300 μL) can quench the 51.3% fluorescence intensity of Eu-PDCA. While 300 μL other anions (BrO3−, Br−, Cl−, SO42−, H2PO4−, NO2−, F−, HCO3−, CH3COO−) processed weaker quench ability for Eu-PDCA. In addition, added PO43− (50 μL), the relative fluorescence intensity of Eu-PDCA was increased from 1 to 3.557, belong to fluorescence enhancement. However, when the volume of PO43− continued to increase, the fluorescence intensity of suspension decreased. Fig. 6b representing the fluorescence spectra of Eu-PDCA (ethanol suspension, 1.0 mL) added 30 μL anions, the result revealed that MnO4− (30 μL) has best fluorescence quench effect for Eu-PDCA, and PO43− has obvious fluorescence enhancement effect with low concentration.
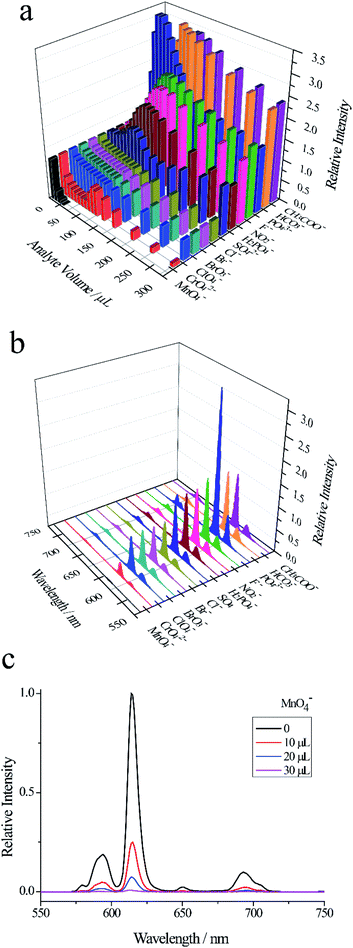 |
| Fig. 6 (a) Bar graph representing the change of the relative emission intensity at 615 nm in the presence of 13 anions. (b) Fluorescence spectra of Eu-PDCA (ethanol suspension, 1.0 mL) added various anions (30 μL) (excited at 265 nm). (c) Fluorescence spectra of Eu-PDCA (ethanol suspension, 1.0 mL) added MnO4− (5 × 10−3 mol L−1) (excited at 265 nm). | |
3.4 Detection mechanism
The spectral overlap, energy competition and transfer32,66,84 were responsible for fluorescent quenching of Eu-PDCA. As can be seen in Fig. S6† of the liquid UV-vis spectra, MG, Cr3+, Fe3+ and Cu2+ in water have wide absorption bands from 200 to 700 nm, which covered the majority absorption band of Eu-PDCA suspension. Under the excitation of light source, there was a competition of absorbing light source energy.85,86 Therefore, MG, Cr3+, Fe3+ and Cu2+ almost filtered all the light adsorption of Eu-PDCA, leading to the fluorescent quenching.
4. Conclusions
We synthesized a europium-based coordination polymer (Eu-PDCA) and surveyed its application in luminescent sensing. Eu-PDCA was capable of quick and effective optical detection of malachite green, cations (Cr3+, Fe3+ and Cu2+) and anion MnO4− via a luminescence quenching mechanism. Among 11 metal cations, 13 anions, six other substances, Eu-PDCA has high quenching efficiency of 2.10 × 106 M−1 and low detection limit of 0.039 μM for MG. It is hoped that our results can provide new inspiration to design fluorescent sensors for MG and ions.
Conflicts of interest
There are no conflicts to declare.
Acknowledgements
This work was financial supported by the National Natural Science Foundation of China (No. 21701048), and the Natural Science Foundation of Shandong Province (ZR2017MB047).
Notes and references
- L. Wu, Z. Z. Lin, H. P. Zhong, A. H. Peng, X. M. Chen and Z. Y. Huang, Food Chem., 2017, 229, 847 CrossRef CAS PubMed.
- R. Sahraei, A. Farmany, S. S. Mortazavi and H. Noorizadeh, Environ. Monit. Assess., 2013, 185, 5817 CrossRef CAS PubMed.
- S. Srivastava, R. Sinha and D. Roy, Aquat. Toxicol., 2004, 66, 319 CrossRef CAS.
- S. L. Stead, H. Ashwin, B. H. Johnston, A. Dallas, S. A. Kazakov, J. A. Tarbin, M. Sharman, J. Kay and B. J. Keely, Anal. Chem., 2010, 82, 2652 CrossRef CAS PubMed.
- T. Xu, X. Wang, Y. Huang, K. Lai and Y. Fan, Food Control, 2019, 106, 106720 CrossRef CAS.
- D. Zhang, H. You, L. Yuan, R. Hao, T. Li and J. Fang, Anal. Chem., 2019, 91, 4687 CrossRef CAS PubMed.
- D. Deng, Q. Lin, H. Li, Z. Huang, Y. Kuang, H. Chen and J. Kong, Talanta, 2019, 200, 272 CrossRef CAS PubMed.
- X. Luo, W. Liu, C. Chen, G. Jiang, X. Hu, H. Zhang and M. Zhong, Opt. Laser Technol., 2021, 139, 106969 CrossRef CAS.
- H. Xie, P. Li, J. Shao, H. Huang, Y. Chen, Z. Jiang, P. K. Chu and X.-F. Yu, ACS Sens., 2019, 4, 2303 CrossRef CAS PubMed.
- X. Zhao, J. Yu, C. Zhang, C. Chen, S. Xu, C. Li, Z. Li, S. Zhang, A. Liu and B. Man, Appl. Surf. Sci., 2018, 455, 1171 CrossRef CAS.
- Y. Quan, R. Su, S. Yang, L. Chen, M. Wei, H. Liu, J. Yang, M. Gao and B. Li, J. Hazard. Mater., 2021, 412, 125209 CrossRef CAS PubMed.
- S. A. Ogundare and W. E. van Zyl, Colloids Surf. A, 2019, 570, 156 CrossRef CAS.
- W. Fang, X. Zhang, Y. Chen, L. Wan, W. Huang, A. Shen and J. Hu, Anal. Chem., 2015, 87, 9217 CrossRef CAS.
- P. Kumar, R. Khosla, M. Soni, D. Deva and S. K. Sharma, Sens. Actuators, B, 2017, 246, 477 CrossRef CAS.
- D. Chen, X. Zhu, J. Huang, G. Wang, Y. Zhao, F. Chen, J. Wei, Z. Song and Y. Zhao, Anal. Chem., 2018, 90, 9048 CrossRef CAS PubMed.
- H. Ran, Z. Lin, C. Hong, J. Zeng, Q.-H. Yao and Z.-Y. Huang, J. Photochem. Photobiol., A, 2019, 372, 260 CrossRef CAS.
- Y. J. Ju, N. Li, S. G. Liu, L. Han, N. Xiao, H. Q. Luo and N. B. Li, Sens. Actuators, B, 2018, 275, 244 CrossRef CAS.
- W. Gui, H. Wang, Y. Liu and Q. Ma, Sens. Actuators, B, 2018, 266, 685 CrossRef CAS.
- L. Wu, Z. Lin, H. Zhong, X. Chen and Z. Huang, Sens. Actuators, B, 2017, 239, 69 CrossRef CAS.
- X. Zhang, Y. Li and L. Zhang, Spectrochim. Acta A, 2021, 251, 119464 CrossRef CAS.
- K. Yi and L. Zhang, Food Chem., 2021, 354, 129584 CrossRef CAS.
- L.-J. Han, Y.-J. Kong, G.-Z. Hou, H.-C. Chen, X.-M. Zhang and H.-G. Zheng, Inorg. Chem., 2020, 59, 7181 CrossRef CAS PubMed.
- F. Amourizi, K. Dashtian, M. Ghaedi and B. Hosseinzadeh, Anal. Methods, 2021, 13, 2603 RSC.
- J. Wang, J. Wu, Y. Zhang, X. Zhou, Z. Hu, X. Liao, B. Sheng, K. Yuan, X. Wu, H. Cai, H. Zhou and P. Sun, Sens. Actuators, B, 2021, 330, 129364 CrossRef CAS.
- B. Unnikrishnan, C.-W. Lien, H.-W. Chu and C.-C. Huang, J. Hazard. Mater., 2021, 401, 123397 CrossRef CAS PubMed.
- Y. Guo, C. Liu, R. Ye and Q. Duan, Appl. Sci., 2020, 10, 6874 CrossRef CAS.
- J. Yang, Y. Zhang, J. Guo, Y. Fang, Z. Pang and J. He, ACS Appl. Mater. Interfaces, 2020, 12, 39118 CrossRef CAS PubMed.
- X. She, H. Xu, Y. Xu, J. Yan, J. Xia, L. Xu, Y. Song, Y. Jiang, Q. Zhang and H. Li, J. Mater. Chem. A, 2014, 2, 2563 RSC.
- J. Du, Y. Sun, L. Jiang, X. Cao, D. Qi, S. Yin, J. Ma, F. Y. C. Boey and X. Chen, small, 2011, 7, 1407 CrossRef CAS PubMed.
- M. Ghaedi, F. Ahmadi and A. Shokrollahi, J. Hazard. Mater., 2007, 142, 272 CrossRef CAS PubMed.
- J. A. Baig, T. G. Kazi, M. B. Arain, H. I. Afridi, G. A. Kandhro, R. A. Sarfraz, M. K. Jamal and A. Q. Shah, J. Hazard. Mater., 2009, 166, 662 CrossRef CAS PubMed.
- L.-J. Han, W. Yan, S.-G. Chen, Z.-Z. Shi and H.-G. Zheng, Inorg. Chem., 2017, 56, 2936 CrossRef CAS PubMed.
- H. N. Kim, W. X. Ren, J. S. Kim and J. Yoon, Chem. Soc. Rev., 2012, 41, 3210 RSC.
- L. N. Neupane, E.-T. Oh, H. J. Park and K.-H. Lee, Anal. Chem., 2016, 88, 3333–3340 CrossRef CAS PubMed.
- G. Yang, X. Wan, Y. Su, X. Zeng and J. Tang, J. Mater. Chem. A, 2016, 4, 12841 RSC.
- A. K. Saini, M. Srivastava, V. Sharma, V. Mishra and S. M. Mobin, Dalton Trans., 2016, 45, 3927 RSC.
- X. Guo, D. Xu, H. Yuan, Q. Luo, S. Tang, L. Liu and Y. Wu, J. Mater. Chem. A, 2019, 7, 27081 RSC.
- M. Ghaedi, F. Ahmadi and A. Shokrollahi, J. Hazard. Mater., 2007, 142, 272 CrossRef CAS PubMed.
- J. Qin, Z. Su, Y. Mao, C. Liu, B. Qi, G. Fang and S. Wang, Ecotoxicol.
Environ. Saf., 2021, 208, 111729 CrossRef CAS.
- X. Fu, E. Chen, B. Ma, Y. Xu, P. Hao, M. Zhang, Z. Ye, X. Yu, C. Li and Q. Ji, Foods, 2021, 10, 413 CrossRef CAS.
- E. M. Krupp, B. F. Milne, A. Mestrot, A. A. Meharg and J. Feldmann, Anal. Bioanal. Chem., 2008, 390, 1753 CrossRef CAS.
- Y.-W. Li, J. Li, X.-Y. Wan, D.-F. Sheng, H. Yan, S.-S. Zhang, H.-Y. Ma, S.-N. Wang, D.-C. Li, Z.-Y. Gao, J.-M. Dou and D. Sun, Inorg. Chem., 2021, 60, 671 CrossRef CAS PubMed.
- Y. Lu, Y. Liang, Y. Zhao, M. Xia, X. Liu, T. Shen, L. Feng, N. Yuan and Q. Chen, ACS Appl. Mater. Interfaces, 2021, 13, 1644 CrossRef CAS.
- Y. Cui, H. Xu, Y. Yue, Z. Guo, J. Yu, Z. Chen, J. Gao, Y. Yang, G. Qian and B. Chen, J. Am. Chem. Soc., 2012, 134, 3979 CrossRef CAS PubMed.
- X. Rao, T. Song, J. Gao, Y. Cui, Y. Yang, C. Wu, B. Chen and G. Qian, J. Am. Chem. Soc., 2013, 135, 15559 CrossRef CAS PubMed.
- Y. Wan, J. Wang, H. Shu, B. Cheng, Z. He, P. Wang and T. Xia, Inorg. Chem., 2021, 60, 7345 CrossRef CAS PubMed.
- E. H. Otal, H. Tanaka, M. L. Kim, J. P. Hinestroza and M. Kimura, Chem. - Eur. J., 2021, 27, 7376 CrossRef CAS PubMed.
- M. Hu, Y. Shu, A. Kirillov, W. Liu, L. Yang and W. Dou, ACS Appl. Mater. Interfaces, 2021, 13, 7625 CrossRef CAS.
- D. A. Gálico, A. A. Kitos, J. S. Ovens, F. A. Sigoli and M. Murugesu, Angew. Chem., Int. Ed., 2021, 60, 6130 CrossRef PubMed.
- T. Gorai, W. Schmitt and T. Gunnlaugsson, Dalton Trans., 2021, 50, 770 RSC.
- J.-M. Wang, P.-F. Zhang, J.-G. Cheng, Y. Wang, L.-L. Ma, G.-P. Yang and Y.-Y. Wang, CrystEngComm, 2021, 23, 411 RSC.
- V. Trannoy, A. N. Carneiro Neto, C. D. S. Brites, L. Carlos and H. Serier-Brault, Adv. Opt. Mater., 2021, 9, 2001938 CrossRef CAS.
- F. Saraci, V. Quezada-Novoa, P. Rafael Donnarumma and A. J. Howarth, Chem. Soc. Rev., 2020, 49, 7949 RSC.
- Y. Cui, Y. Yue, G. Qian and B. Chen, Chem. Rev., 2012, 112, 1126 CrossRef CAS PubMed.
- Y. Cui, B. Chen and G. Qian, Coord. Chem. Rev., 2014, 273, 76 CrossRef.
- M. D. Allendorf, C. A. Bauer, R. K. Bhakta and R. J. T. Houk, Chem. Soc. Rev., 2009, 38, 1330 RSC.
- F.-Y. Yi, D. Chen, M.-K. Wu, L. Han and H.-L. Jiang, ChemPlusChem, 2016, 81, 675 CrossRef CAS PubMed.
- K. A. White, D. A. Chengelis, K. A. Gogick, J. Stehman, N. L. Rosi and S. Petoud, J. Am. Chem. Soc., 2009, 131, 18069 CrossRef CAS PubMed.
- B. Yan, Acc. Chem. Res., 2017, 50, 2789 CrossRef CAS PubMed.
- P. Zhang, N. Song, S. Liu, Q. Li, Y. Wang and B. Zhou, J. Mater. Chem. C, 2021, 9, 6208 RSC.
- J. Liu, X. Han, Y. Lu, S. Wang, D. Zhao and C. Li, Inorg. Chem., 2021, 60, 4133 CrossRef CAS PubMed.
- T. Xia, Y. Wan, X. Yan, L. Hu, Z. Wu and J. Zhang, Dalton Trans., 2021, 50, 2792 RSC.
- Y.-Q. Sun, Y. Cheng and X.-B. Yin, Anal. Chem., 2021, 93, 3559 CrossRef CAS PubMed.
- K. Yin, S. Wu, H. Zheng, L. Gao, J. Liu, C. Yang, L.-W. Qi and J. Peng, Langmuir, 2021, 37, 5321 CrossRef CAS PubMed.
- Y.-J. Tong, L.-D. Yu, J. Zheng, G. Liu, Y. Ye, S. Huang, G. Chen, H. Yang, C. Wen, S. Wei, J. Xu, F. Zhu, J. Pawliszyn and G. Ouyang, Anal. Chem., 2020, 92, 15550 CrossRef CAS PubMed.
- L. J. Han, Y.-J. Kong, X.-M. Zhang, G.-Z. Hou, H.-C. Chen and H.-G. Zheng, J. Mater. Chem. C, 2021, 9, 6051 RSC.
- Q. Lin, W. Xie, Z. Zong, Z. Liu, Y. Sun and L. Liang, New J. Chem., 2021, 45, 7382 RSC.
- Z.-G. Lin, F.-Q. Song, H. Wang, X.-Q. Song, X.-X. Yu and W. Liu, Dalton Trans., 2021, 50, 1874 RSC.
- Y. Shu, Q. Ye, T. Dai, Q. Xu and X. Hu, ACS Sens., 2021, 6, 641 CrossRef CAS PubMed.
- L. Yu, L. Feng, L. Xiong, S. Li, Q. Xu, X. Pan and Y. Xiao, ACS Appl. Mater. Interfaces, 2021, 13, 11646 CrossRef CAS PubMed.
- B. Li, J.-P. Dong, Z. Zhou, R. Wang, L.-Y. Wang and S.-Q. Zang, J. Mater. Chem. C, 2021, 9, 3429 RSC.
- J.-P. Dong, B. Li, Y.-J. Jin and L.-Y. Wang, CrystEngComm, 2021, 23, 1677 RSC.
- J. Jin, J. Xue, Y. Liu, G. Yang and Y.-Y. Wang, Dalton Trans., 2021, 50, 1950 RSC.
- M. Wang, Z. Liu, X. Zhou, H. Xiao, Y. You and W. Huang, Inorg. Chem., 2020, 59, 18027 CrossRef CAS PubMed.
- L.-J. Liu, M.-Y. Zhang, Q.-Z. Guo, Z.-H. Zhang and J.-F. Guo, Dalton Trans., 2021, 50, 1697 RSC.
- W.-P. Ma and B. Yan, Dalton Trans., 2020, 49, 15663 RSC.
- X.-J. Che, S.-L. Hou, Y. Shi, G.-L. Yang, Y.-L. Hou and B. Zhao, Dalton Trans., 2019, 48, 3453 RSC.
- H.-Y. Zheng, X. Lian, S.-j. Qin and B. Yan, Dalton Trans., 2018, 47, 6210 RSC.
- W. Yan, C. Zhang, S. Chen, L. Han and H. Zheng, ACS Appl. Mater. Interfaces, 2017, 9, 1629 CrossRef CAS PubMed.
- H. Wang, J. Qin, C. Huang, Y. Han, W. Xu and H. Hou, Dalton Trans., 2016, 45, 12710 RSC.
- D. Tian, Y. Li, R.-Y. Chen, Z. Chang, G.-Y. Wang and X.-H. Bu, J. Mater. Chem. A, 2014, 2, 1465 RSC.
- F. G. Moscoso, J. Almeida, A. Sousaraei, T. Lopes-Costa, A. G. Silva, J. Cabanillas-Gonzalez, L. Cunha-Silva and J. Pedrosa, J. Mater. Chem. C, 2020, 8, 3626 RSC.
- Z. Zhan, X. Liang, X. Zhang, Y. Jia and M. Hu, Dalton Trans., 2019, 48, 1786 RSC.
- Z. Hu, W. P. Lustig, J. Zhang, C. Zheng, H. Wang, S. J. Teat, Q. Gong, N. D. Rudd and J. Li, J. Am. Chem. Soc., 2015, 137, 16209 CrossRef CAS PubMed.
- S. Nagarkar, B. Joarder, A. K. Chaudhari, S. Mukherjee and S. K. Ghosh, Angew. Chem., Int. Ed., 2013, 52, 2881 CrossRef CAS PubMed.
- Z. Hu, K. Tan, W. P. Lustig, H. Wang, Y. Zhao, C. Zheng, D. Banerjee, T. J. Emge, Y. J. Chabal and J. Li, Chem. Sci., 2014, 5, 4873 RSC.
Footnote |
† Electronic supplementary information (ESI) available: The supporting figures are provided. CCDC 2082443. For ESI and crystallographic data in CIF or other electronic format see DOI: 10.1039/d2ra00077f |
|
This journal is © The Royal Society of Chemistry 2022 |
Click here to see how this site uses Cookies. View our privacy policy here.