DOI:
10.1039/D1RA08978A
(Paper)
RSC Adv., 2022,
12, 4191-4198
Dual inhibition of the renin and angiotensin converting enzyme activities of aqueous extracts of 22 edible flower petals
Received
13th December 2021
, Accepted 12th January 2022
First published on 2nd February 2022
Abstract
In order to explore novel blood pressure-regulating substances and fulfill the high-value utilization of various edible flowers, the inhibitory activities of aqueous solutions of 22 edible flower petals extracts on renin and angiotensin converting enzyme (ACE) were investigated. The results showed that almost all the aqueous sample extracts demonstrated an inhibition of renin and/or ACE. The Rosa rugosa Thunb. (IC50 = 25.13 and 60.00 μg mL−1) and Paeonia suffruticosa Andr. (IC50 = 50.54 and 292.47 μg mL−1) extracts showed prominent dual inhibitory activity against renin and ACE. The antioxidant activities and content of total phenols and flavonoids of the aqueous sample extracts were tested, because the oxidative damage of blood vessels is closely related to the occurrence and development of hypertension. The correlation between the contents of total phenolic substances and flavonoids, and the functional activities was analyzed. Renin and ACE inhibitory activities, DPPH and ABTS free radical scavenging capacity, and iron reduction ability of different sample extracts were significantly positively correlated with the total phenolic content (p <0.01), whereby the correlation coefficients were 0.87, 0.83, 0.93, 0.95, and 0.93 respectively. It was indicated that the aqueous phenolic compounds in Rosa rugosa Thunb and Paeonia suffruticosa Andr extracts tended to show stronger renin and ACE inhibitory activities, and exhibited a potential prospect for auxiliary blood pressure control.
1 Introduction
Hypertension is a global public health problem with increasing prevalence.1 The renin-angiotensin system (RAS) plays a crucial role in modulating blood pressure in the human body. Renin (EC 3.4.23.15), a rate limiting enzyme, can hydrolyze the N-terminus of angiotensinogen to yield angiotensin I, which is then further hydrolyzed to angiotensin II with strong vasoconstriction under the catalysis of angiotensin converting enzyme (ACE; EC 3.4.15.1), resulting in an increase in blood pressure. Hence, the control of the RAS has been well established as an effective therapy for the treatment of hypertension. Currently, synthetic renin inhibitors, like aliskiren, and synthetic ACE inhibitors, like captopril and enalapril, are widely applied clinically as antihypertensive drugs. However, some side effects, such as dry cough, allergic reaction, and taste disturbance, are associated with the usage of these inhibitors and thought to be unavoidable.2 The research into renin inhibitors and an ACE inhibitor is focused on food sources, which are considered to be easily absorbed, and to have less or no side effects.3
Recently, many renin and/or ACE inhibitory peptides have been reported from plant or foodstuff sources, such as flaxseed protein,4,5 pea protein,6 Bovine Fibrinogen,7 amaranth proteins,8 Peptide from Red Seaweed palmaria palata,9 and hemp seed proteins.10 Although these new identified peptides have provided new leading molecules that can inhibit renin and/or ACE, clear limitations still exist with these peptides. Even though several peptides have shown good activity in vitro, their efficacy cannot meet therapeutic requirements due to low bioavailability. For example, some peptides have fast metabolisms in vivo and are broken down to inactive metabolites.11 Therefore, the search for safer and more effective natural inhibitors is still ongoing.
Some clinical experiments have shown that the intake of some polyphenol-rich substances have a beneficial effect in reducing blood pressure,12,13 attributed to possibly reducing oxidative damage to blood vessels by polyphenols. It is noticeable that polyphenolic compounds were found to potentially contribute to renin inhibition and ACE inhibition; which could be supported by the renin inhibitory activities of tea,14 saponin from soybean,15 polyphenolic extracts of two green leafy vegetables vernonia amygdalina, and gongronema latifolium,16 and by the ACE inhibitory activities of polyphenols from tomato,17 flavonoids from the buds of Rosa damascene,18 and polyphenols from Indian gooseberry.19 All these studies suggested that polyphenolic ingredients are promising as candidates for renin inhibitors and ACE inhibitors.
Edible flowers are rich in polyphenols and are non-toxic and many have been used since ancient times for their nutritional and health benefits. Also, the biological activities of edible flowers have been widely studied in recent years. While most articles focus on the antioxidant activity of edible flowers,20–22 little information has been reported on the several biological properties associated with edible flowers, such as the improvement of ulcerative colitis,23 anti-hyperglycemic and anticholinergic activity,24 protection against oxidative effects in erythrocytes,25 and even anticancer activity.26 These health benefits are attributed to their phytochemicals, such as phenolic acids and flavonoids. Nonetheless, to date, little attention has been paid to their potential renin and ACE. inhibitory effects. The present study aimed to investigate in vitro the renin and ACE inhibitory activities of 22 normal edible flowers. Further, the antioxidant activity of the samples was investigated, and the correlation between the contents of polyphenols and flavonoids and their functional activities was analyzed, which provided a new idea for exploring new blood pressure-regulatory factors, and provided a richer theoretical basis for further improving the functional value system of edible flowers, thereby allowing realizing their high-value utilization and promoting the development of the related edible flower food industry.
2 Material and methods
2.1 Materials
The human recombinant renin inhibitor screening assay kit, including human recombinant renin and substrate (Ar-gGlu(EDANS)-Ile-His-Pro-Phe-His-Leu-Val-Ile-His-Thr-Lys(Dabcyl)-Arg), was purchased from Cayman Chemical Co. (Ann Arbor, MI, USA). Angiotensin I-converting enzyme (ACE, from rabbit lung), N-Hippuryl-His-Leu tetrahydrate (HHL), o-phthaldialdehyde (OPA), Folin–Ciocalteu phenol reagent, 1,1′-diphenyl-2-picrylhydrazyl (DPPH), 2,2′-azinobis(3-ethylbenzothiazoline-6-sulfonic acid) diammonium salt (ABTS), 6-hydroxy-2,5,7,8-tetramethylchroman-2-carboxylic acid (Trolox), 2,4,6-tri(2-pyridyl)-s-triazine (TPTZ), gallic acid, and catechin were sourced from Sigma-Aldrich (St. Louis, MO, USA).
The edible flowers and their manufacturers are listed in Table 1.
Table 1 The number and manufacturer of the experimental materials
Number |
Name |
Manufacturer |
1 |
Rosa rugosa Thunb. |
Fujian Zhifu Ecological Agriculture Development Co., Ltd. (sub packaging) |
2 |
Jasminum sambac (L.) Ait. |
Fujian Zhifu Ecological Agriculture Development Co., Ltd. (sub packaging) |
3 |
Dendranthema morifolium Ramat. |
Wuhu Dongfang Tea Co., Ltd |
4 |
Dendranthema morifolium Tzvel. |
Fujian Zhifu Ecological Agriculture Development Co., Ltd. (sub packaging) |
5 |
Lonicera japonica Thunb. |
Wuhu Dongfang Tea Co., Ltd |
6 |
Osmanthus fragrans (Thunb.) Lour. |
Wuhu Dongfang Tea Co., Ltd |
7 |
Coreopsis tinctoria Nutt. |
Hangzhou yifutang Tea Co., Ltd |
8 |
Gomphrena globosa Linn. |
Mingyuan bee Technology Co., Ltd |
9 |
Magnolia liliflora Desr. |
Mingyuan bee Technology Co., Ltd |
10 |
Armeniaca mume Sieb. |
Mingyuan bee Technology Co., Ltd |
11 |
Paeonia suffruticosa Andr. |
Mingyuan bee Technology Co., Ltd |
12 |
Lavandula angustifolia Mill. |
Mingyuan bee Technology Co., Ltd |
13 |
Lilium brownii var. viridulum |
Mingyuan bee Technology Co., Ltd |
14 |
Magnolia denudata Desr. |
Mingyuan bee Technology Co., Ltd |
15 |
Prunus persica (L.) Batsch. |
Mingyuan bee Technology Co., Ltd |
16 |
Celastrus orbiculatus Thunb. |
Mingyuan bee Technology Co., Ltd |
17 |
Myosotis silvatica Ehrh. ex Hoffm. |
Mingyuan bee Technology Co., Ltd |
18 |
Flower of Siraitia grosvenorii (Swingle) C. Jeffrey ex A. M. Lu et Z. Y. Zhang |
Mingyuan bee Technology Co., Ltd |
19 |
Camellia japonica L. |
Mingyuan bee Technology Co., Ltd |
20 |
Citrus aurantium L. var. amara Engl. |
Mingyuan bee Technology Co., Ltd |
21 |
Sophora japonica L. |
Fujian Anxi YIZUN Tea Co., Ltd |
22 |
Flower of Eucommia ulmoides male |
Fujian Anxi YIZUN Tea Co., Ltd |
2.2 Preparation of sample extracts
All samples were powdered by a pulverizer. Each sample powder (0.5 g) was dispersed in 10 mL distilled water. After mixing sufficiently, the suspension was centrifuged at 5000 rpm for 10 min. The resulted supernatant was collected, followed by filtration through a 0.45 μm membrane. An original concentration of 50.0 mg mL−1 for each dried flower powder was prepared prior to the later experiments.
2.3 Assay for renin inhibition
The determination of renin inhibition was based on the evaluation method of Li et al.14 and used the human recombinant renin inhibitor screening kit. The specific steps are as follows, starting with preparing the test solutions: (1) blank: 20 μL substrate, 160 μL buffer, 10 μL distilled water; (2) Control: 20 μL substrate, 160 μL buffer, 10 μL distilled water; (3) Sample: 20 μL substrate, 160 μL buffer, 10 μL sample solution. Then add 10 renin enzyme solution to the control and sample wells to start the reaction, and add 10 μL buffer to the blank wells, letting it stand at 37 °C for 15 min. The synthetic fluorescence resonance energy transfer peptide utilized in this assay was the normal substrate for renin and was linked to a fluorophore at one end and to a nonfluorescent chromophore at the other end. After the peptide was cleaved by renin, the product obtained was highly fluorescent and could easily be analyzed by recording the fluorescence intensity (FI) on a fluorescence plate reader (Powerscan HT; BioTek Instruments, Inc., Winooski, VT, U.S.), with an excitation wavelength of 360 nm and an emission wavelength of 528 nm. The renin inhibitory activity was calculated as follows:
The analyses were performed in triplicate. The dose-dependent suppression of renin activity was evaluated with at least four different concentrations of the inhibitor. The IC50 value was defined as the concentration of the inhibitor required to inhibit 50% of the renin activity, and was calculated using the linear function of percentage renin inhibition versus the logarithm of the inhibitor concentration.
2.4 Assay for ACE inhibition
The determination of the ACE inhibitory activity was based on the evaluation method of Li et al.27 The aqueous extract samples were appropriately diluted, and a 96-well microtiter plate was used as a reaction container. Next, 15 μL of the sample solution (replaced with distilled water in the control reaction solution) was mixed with 30 μL of 4.66 mmol L−1 of HHL (dissolved in 0.6 mol L−1 NaCl to 0.4 mol L−1 phosphate buffer, pH 8.5). Then, 30 μL of 12.5 mU mL−1 ACE enzyme solution (the blank of the sample reaction solution and the control reaction solution was replaced by distilled water) was added and mixed on a microplate mixer, and reacted at 37 °C for 1 h. Next, 120 μL of 1.2 mol L−1 NaOH solution was added to stop the enzyme reaction, and then 30 μL of 2% OPA solution (dissolved in methanol) added, mixed, and the mixture allowed to stand at room temperature for 20 min, followed by adding add 30 μL of 6 mol L−1 HCl solution to terminate the derivatization reaction. The fluorescence absorption intensity was measured under the following conditions: excitation wavelength, 340 nm; emission wavelength, 455 nm; slit width, 5 nm. The ACE inhibition rate of the sample is calculated as follows:
where I1 indicates the fluorescence absorption intensity of the sample reaction solution in the presence of the ACE inhibitor; I2 indicates that the control reaction solution has no ACE inhibition, and shows the fluorescence absorption intensity of the agent.
2.5 Determination of the total antioxidant capacity
2.5.1 DPPH free radical scavenging assay. The DPPH assay was conducted according to the method described by Brand-Williams,28 with some modifications. Samples with a concentration of 5 mg mL−1 at 5 μL were mixed with 200 μL of 0.08 mg mL−1 DPPH ethanol solution. After incubation for 30 min in darkness, each absorbance was measured at 517 nm. In the blank control, the same volume of distilled water was used instead of the sample, and the final result was expressed as the antioxidant capacity by mg TE per g DW. The scavenging rate of DPPH was calculated according to the following formula:
where I is the DPPH scavenging rate; Ac is the absorbance of the blank; Ai is the absorbance in the presence of the sample; and Aj is the absorbance without DPPH.
2.5.2 Determination of the ABTS + scavenging capacity. The ABTS radical scavenging activity assay was performed by following the method described by van den Berg et al.29 ABTS reagent was formed by 5 mL of 7 mmol L−1 ABTS stock solution and 88 μL of 140 mmol L−1 potassium persulfate in the dark for 12 h. This was diluted with ethanol before use for absorbance at 0.70 ± 0.02 at 732 nm. Next, 25 μL of the sample extract was added to 2 mL of ABTS solution for 6 min in the dark for determination of the absorbance. Trolox solution (final concentration 0.03125–1 mg mL−1) was used as a reference standard. The results were expressed in mg Trolox per g DW (mg TE per g DW). The scavenging rate of ABTS was calculated on the basis of the following formula:
where I is the ABTS scavenging rate; A0 is the absorbance of the blank; and A1 is the absorbance of the test sample.
2.5.3 Ferric reducing activity power (FRAP) assay. The FRAP assay was performed according to the Benzie and Strain method.30 Here, 10 μL of sample extract was sequentially added with 1 mL of distilled water, and 1.8 mL of TPTZ solution (preparation of the TPTZ solution consisted of 0.3 mol L−1 acetate buffer 25 mL, 10 mmol L−1 TPTZ working solution 2.5 mL, and 20 mmol L−1 FeCl3 solution 2.5 mL) was added, with mixing and reacting at 37 °C for 10 min. Finally, the absorbance was determined at 593 nm. The standard curve was constructed using Trolox solution (0.03125–1 mg mL−1). The results were expressed as mg Trolox per g dry weight of plant material (mg TE per g DW).
2.6 Determination of the content of the total phenolics
The total phenolics content (TPC) was determined by the Folin–Ciocalteu method according to the method of McDonald et al.31 with minor modification. Each 100 μL of sample solution was added to 100 μL of 10% Folin–Ciocalteu solution and incubated at 37 °C. After 10 min, 100 μL of 10% Na2CO3 was added and the mixture was allowed to stand at 37 °C for 60 min in the dark. The absorbance was measured at 750 nm. The standard curve was established using various concentrations of gallic acid in distilled water. The TPC was expressed as mg gallic acid equivalent (GAE) per g dried weight (DW).
2.7 Determination of the content of flavonoids
The total flavonoids (TF) was analyzed by the method described by Chang et al.,32 in which 100 μL of extract was mixed with 625 μL of distilled water and 375 μL of 5% NaNO2 solution. After 6 min, 75 μL of a 10% AlCl3·6H2O solution was added and the mixture was left for another 5 min before the addition of 250 μL of 1 M NaOH. The absorbance was measured at 510 nm using a spectrophotometer. The results were expressed as mg of catechin equivalents per g of dry weight (mg CE per g DW).
2.8 Statistical analysis
Data are the means of triplicate analyses and expressed as the mean ± standard error. Data were analyzed using IBM SPSS Statistics version 19.0. Duncan's multiple range test was used to evaluate differences among the samples. Differences at p <0.05 were considered statistically significant.
3 Results and discussion
3.1 Renin and ACE inhibitory activities of 22 edible flowers
The concentration of water extracts from the edible flowers used in the experiment for assessing the renin and ACE inhibitory activity was 5 mg mL−1. The inhibition rates of renin and ACE in the 22 edible flowers are shown in Fig. 1. For the renin inhibitory activities assay, Paeonia suffruticosa Andr., Rosa rugosa Thunb., and Camellia japonica L. showed better inhibition activity, reaching 71.25%, 42.19%, and 38.48% respectively. Meanwhile, the ACE activity of the 22 edible flowers was determined. The ACE inhibitory activity of Sophora japonica L. was the highest, reaching 81.61%, followed by Rosa rugosa Thunb. (61.50%) and Citrus aurantium (49.81%). The inhibition rate of ACE in Paeonia suffruticosa Andr. was 44.89%.
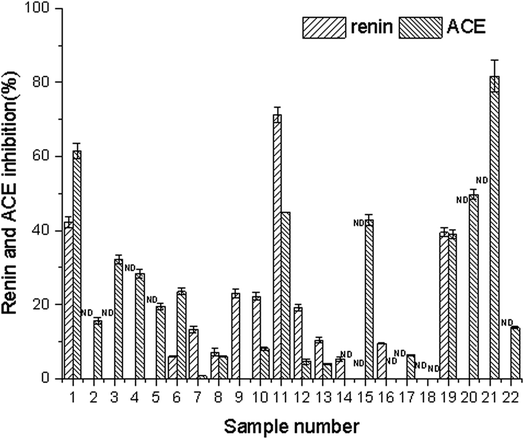 |
| Fig. 1 Inhibition of renin and ACE by different aqueous sample extracts (the flowers represented by numbers are shown in Table 1). | |
In this study, aqueous solutions of 22 edible flowers extracts were tested for their inhibitory activity against renin and ACE for the first time in a comparative study. The results showed that almost all the aqueous sample extracts inhibited renin and/or ACE. Rosa rugosa Thunb. and Paeonia suffruticosa Andr. had the most prominent double inhibitory activity on renin and ACE. Therefore, the inhibitory activities against renin and ACE of Rosa rugosa Thunb. and Paeonia suffruticosa Andr. water extracts were determined at different concentrations. The inhibition rate and calculated IC50 value are indicated in Fig. 2. The IC50 values of renin and ACE inhibited by sample 1 (Rosa rugosa Thunb.) were 25.13 μg mL−1 and 60.00 μg mL−1, while the IC50 values of renin and ACE inhibited by sample 11 (Paeonia suffruticosa Andr.) were 50.54 μg mL−1 and 292.47 μg mL−1. Compared with other reported natural renin and ACE inhibitors, like peptides from wheat gluten hydrolysate with an inhibition ACE IC50 value of 36 μg mL−1,33 the different components of protease hydrolysates of Phascolosoma esculenta with an inhibition ACE IC50 value of 430–1410 μg mL−1,34 peptides from amaranth proteins with an inhibition renin IC50 value of 600 μg mL−1,8 the extracts from legume (except soybean and peanut) with an inhibition renin IC50 value of 270–1700 μg mL−1, soyasaponin from soybean with an inhibition renin IC50 value of 30 μg mL−1,15 in this study, the aqueous extracts of rose and peony flowers had strong renin and ACE inhibitory activities, which can thus be regarded as an important source of natural renin and ACE inhibitory substances.
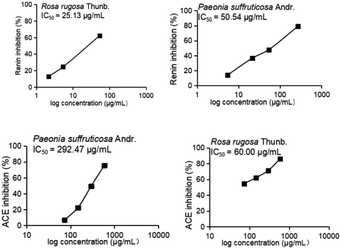 |
| Fig. 2 Inhibition of renin and ACE by aqueous sample extracts at different concentrations. | |
3.2 Total antioxidant capacity of the 22 edible flowers
The antioxidant power of edible flowers has been found to be very high, compared to that of common fruits and vegetables because of the highly bioactive components.35 The composition of the extract and the conditions of the test system have a large effect on the antioxidant capacity of the plant extract. Many factors influence the antioxidant capacity, and these factors cannot be fully described by one single method. Therefore, it is necessary to perform more than one type of antioxidant capacity measurement to take into account the various mechanisms of antioxidant action. In this paper, the extracts of 22 edible flowers were evaluated for the antioxidant capacities using the DPPH, ABTS, and FRAP assays. The antioxidant activities of the water extracts of various edible flowers are listed in Fig. 3.
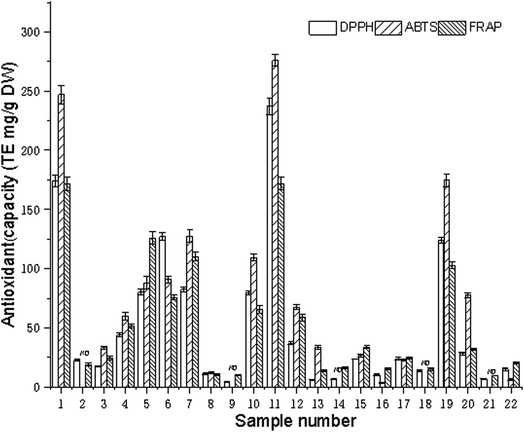 |
| Fig. 3 Antioxidant capacity of different aqueous sample extracts (the flowers represented by numbers are shown in Table 1). | |
For the DPPH assay, the antioxidant capacity ranged from 4.33 to 237.24 mg TE per g DW, and the difference in antioxidant capacities was up to 54-fold. Sample 11 (Paeonia suffruticosa Andr.) was found to have the highest antioxidant capacity (237.24 mg TE per g DW), followed by Rosa rugosa Thunb. (174.18 mg TE per g DW), Osmanthus fragrans (Thunb.) Lour. (127.10 mg TE per g DW), Camellia japonica L. (123.59 mg TE per g DW), and Coreopsis drummondii Torr. et Gray (82.50 mg TE per gDW). Magnolia biondii Pamp. possessed the lowest antioxidant capacity (4.33 mg TE per g DW).
The ABTS radical scavenging activity varied from 0 to 276.02 mg TE per g DW with a 1380-fold difference for the 22 edible flowers. Paeonia suffruticosa Andr. (276.02 mg TE per g DW), Rosa rugosa Thunb. (246.95 mg TE per g DW), and Camellia japonica L. (174.60 mg TE per g DW) had the highest free radical scavenging capacities, while Jasminum sambac (L.) Ait., Magnolia biondii Pamp., Magnolia denudata Desr., Flower of Siraitia grosvenorii (Swingle) C. Jeffrey ex A.M.Lu et Z.Y.Zhang, and Sophora japonica L. did not show significant ABTS radical scavenging activity in this assay.
The FRAP assay is a simple and widely used method for the evaluation of the antioxidant capacity. The ferric reducing activity power ranged from 9.49 to 172.00 mg TE per g DW. The difference in antioxidant capacities was up to 18 = fold. Rosa rugosa Thunb. (172.00 mg TE per g DW) was found to have the highest reducing power, followed by Paeonia suffruticosa Andr. (171.95 mg TE per g DW), Lonicera japonica Thunb. (125.94 mg TE per g DW), and Coreopsis drummondii Torr. et Gray (110.27 mg TE per g DW).
The antioxidant capacity of the 22 kinds of edible flowers was quite different; among them, sample 1 (Rosa rugosa Thunb), sample 11 (Paeonia suffruticosa Andr). and sample 19 (Camellia japonica L.) had strong antioxidant capacities. Some articles have reported that Rosa rugosa Thunb. and Paeonia suffruticosa Andr. extracts have excellent antioxidant capacity,36,37 as well as an inhibitory effect on some cancer cells,20,38 and have good preventive effects on cardiovascular and cerebrovascular diseases due to them being rich in flavonoids and polyphenols.25,39 It is worth noting that flowers with strong antioxidant activity also have a strong inhibitory effect on renin and ACE activity. In Table 2, it can be seen that the renin and ACE inhibitory activities were significantly positively correlated with the total antioxidant capacity (p <0.01), and the correlation coefficients of renin and ACE inhibition with DPPH, ABTS, and FRAP were 0.85, 0.90, 0.72 and 0.76, 0.79, 0.64.
Table 2 Correlation analysis between the activities and contents of total phenols and flavonoids of different aqueous samples extracts
Pearson correlation |
Renin |
ACE |
DPPH |
ABTS |
FRAP |
TPC |
TF |
Renin |
1 |
|
|
|
|
|
|
ACE |
0.78(**) |
1 |
|
|
|
|
|
DPPH |
0.85(**) |
0.76(**) |
1 |
|
|
|
|
ABTS |
0.90(**) |
0.79(**) |
0.99(**) |
1 |
|
|
|
FRAP |
0.72(**) |
0.64 |
0.93(**) |
0.94(**) |
1 |
|
|
TPC |
0.87(**) |
0.83(**) |
0.93(**) |
0.95(**) |
0.93(**) |
1 |
|
TF |
0.000 |
0.016 |
0.43(*) |
0.35 |
0.57(**) |
0.391 |
1 |
3.3 TPC and TF
The determination of the total amount of plant phenolic compounds as the main antioxidant or free radical terminator is reasonable. Flavonoids, as one of the most diverse and widely distributed natural compounds, may be the most important natural phenolic compounds The total phenolic contents of 22 edible flowers were measured using the Folin–Ciocalteu method, and are also shown in Fig. 4. These compounds have a wide range of chemical and biological activities, including radical scavenging properties.
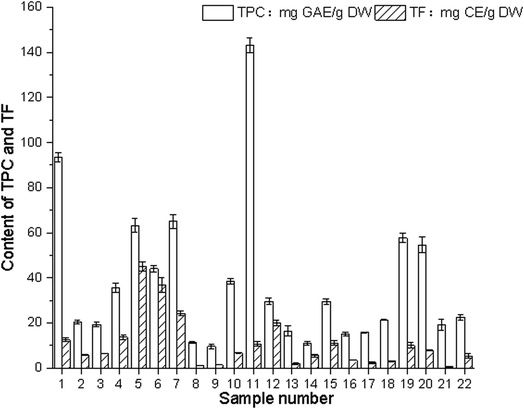 |
| Fig. 4 Contents of total phenols and flavonoids in different aqueous sample extracts (The flowers represented by numbers are shown in Table 1). | |
The content of phenolic compounds (mg g−1) in water extracts expressed in gallic acid equivalents (GAE) varied between 9.60 and 143.17 mg GAE per g DW. The difference in total phenolic content was up to 46-fold. Paeonia suffruticosa Andr. had the highest phenolic content (143.17 mg GAE per g DW), followed by Rosa rugosa Thunb. (103.57 mg GAE per g DW).
The concentration of flavonoids was expressed in catechin equivalents in mg g−1 of plant extract. The total flavonoid contents of the materials varied from 0.58 mg CE per g DW (Sophora japonica L.) to 45.18 mg CE per g DW (Lonicera japonica Thunb.). The difference in total flavonoid content was significant, up to 78-fold, varying from 0.58 mg CE per g DW (Sophora japonica L.) to 45.18 mg CE per g DW (Lonicera japonica Thunb.). The difference in total flavonoid content was significant, up to 78-fold.
In some recent studies, it was observed that the treatment of CVDs and many other diseases can be made possible by the polyphenols that are present in some medicinal plants. In vivo, in vitro, and numerous clinical trials have reported that the consumption of a polyphenol-rich diet may be significantly beneficial in the prevention and treatment of CVDs due to their antioxidant, anti-inflammatory, antiplatelets and other pleiotropic actions.40,41 It is worth noting that polyphenols have been found to have renin and ACE inhibitory activities, such as tea polyphenols in black tea and oolong tea,14 flavonoids in rose buds,18 polyphenol extracts from vernonia amygdalina and gongronema latifolium leaves,16 and soyasaponin in soybeans.15 In this study, we found that there were significant differences in the renin and ACE inhibitory and antioxidant activities of water extracts from different edible flowers. While all these activities were significantly correlated with the polyphenol content, as can be seen in Table 2 the correlation coefficients of the total phenol content with renin and ACE inhibition were 0.871 and 0.826, respectively. The correlation coefficients of total phenol content with DPPH, ABTS free radical scavenging capacity, and iron ion reduction ability were 0.93, 0.95, and 0.93, respectively. These studies show that polyphenols can be regarded as important renin and ACE inhibitory activity substances.
4 Conclusions
In this study, aqueous solutions of 22 edible flowers extracts and their inhibitory activity against renin and ACE were studied for the first time in a comparative study. The results showed that almost all the aqueous sample extracts could inhibit renin and/or ACE. Rosa rugosa Thunb. and Paeonia suffruticosa Andr. had the most prominent double inhibitory activity against renin and ACE, Camellia japonica L, Sophora japonica L, Prunus persica (L.) Batsch, and Citrus aurantium L. var. amara Engl. showed moderate renin and/or ACE inhibitory activity. Compared with other samples, they also had higher ABTS, DPPH free radical scavenging ability, and iron ion reduction ability. At the same time, the renin and ACE inhibitory activities and antioxidant capacity of water extracts from different samples were significantly positively correlated with the polyphenol content (p <0.01). The purification, identification, and mechanism of renin and ACE inhibitory active components in aqueous Rosa rugosa Thunb. and Paeonia suffruticosa Andr. extracts will be done in the next work, so as to provide theoretical support for the excavation of new blood pressure-regulation functional factors and for the high-value utilization of peony flowers and the development of edible-flower-related industries.
Conflicts of interest
The authors declare that they have no conflict of interest.
Acknowledgements
This work was funded by Tianjin Natural Science Foundation Project (16JCYBJC23200).
References
- C. Bavishi, S. Bangalore and F. H. Messerli, Prog. Cardiovasc. Dis., 2016, 59, 253–261 CrossRef PubMed.
- Z. D. Bekassy, A. C. Kristoffersson, J. Rebetz, R. Tati, A. I. Olin and D. Karpman, Kidney Int., 2018, 94, 689–700 CrossRef CAS PubMed.
- L. Du, M. Fang, H. Wu, J. Xie, Y. Wu, P. Li, D. Zhang, Z. Huang, Y. Xia, L. Zhou and D. A. Wei, J. Funct. Foods, 2013, 5, 475–483 CrossRef CAS.
- C. C. Udenigwe, Y. S. Lin, W. C. Hou and R. E. Aluko, J. Funct. Foods, 2009, 1, 199–207 CrossRef.
- I. Nwachukwu, A. Girgih, S. Malomo, J. Onuh and R. Aluko, Int. J. Mol. Sci., 2014, 15, 18131–18147 CrossRef CAS PubMed.
- H. Li and R. E. Aluko, J. Agric. Food Chem., 2010, 58, 11471–11476 CrossRef CAS PubMed.
- T. Lafarga, D. K. Rai, P. O'Connor and M. Hayes, J. Agric. Food Chem., 2015, 63, 8676–8684 CrossRef CAS PubMed.
- A. E. Nardo, M. C. Añón and A. V. Quiroga, J. Funct. Foods, 2019, 104683 Search PubMed.
- C. Fitzgerald, R. E. Aluko, M. Hossain, D. K. Rai and M. Hayes, J. Agric. Food Chem., 2014, 62, 8352–8356 CrossRef CAS PubMed.
- A. Montoya-Rodríguez, M. A. Gómez-Favela, C. Reyes-Moreno, J. Milán-Carrillo and E. González de Mejía, Compr. Rev. Food Sci. Food Saf., 2015, 14, 139–158 CrossRef PubMed.
- T. Tavares, M. D. Contreras, M. Amorim, M. Pintado, I. Recio and F. X. Malcata, Peptides, 2011, 32, 1013–1019 CrossRef CAS PubMed.
- T. E. Tjelle, L. Holtung, S. K. Hn and K. Aaby, Br. J. Nutr., 2015, 114, 1054–1063 CrossRef CAS PubMed.
- R. L. Noad, C. Rooney, D. Mccall and I. S. Young, Heart, 2016, 64, 1172–1174 Search PubMed.
- F. Li, M. Ohnishi-Kameyama, Y. Takahashi and K. Yamaki, J. Agric. Food Chem., 2013, 61, 9697–9704 CAS.
- S. Takahashi, K. Hori, M. Shinbo, K. Hiwatashi, T. Gotoh and S. Yamada, Biosci Biotechnol Biochem, 2008, 72, 3232–3236 CrossRef CAS PubMed.
- C. F. Ajibola, A. F. Eleyinmi and R. E. Aluko, Biosci., Biotechnol., Biochem., 2011, 66, 320–327 CAS.
- W. Blaszczak, M. Jez and A. Szwengiel, Food Chem., 2020, 314, 126–128 CrossRef PubMed.
- E. K. Kwon, D. Y. Lee, H. Lee, D. O. Kim, I. Baek, Y. E. Kim and H. Y. Kim, J. Agric. Food Chem., 2010, 58, 882–886 CrossRef CAS PubMed.
- N. Chaudhary, L. Sabikhi, S. A. Hussain and S. A. Kumar M H, J. Herb. Med., 2020, 100343 CrossRef.
- G. L. Chen, S. G. Chen, Y. Xiao and N. L. Fu, Ind. Crops Prod., 2018, 111, 430–445 CrossRef CAS.
- Y. J. Xie and W. Zhang, J. Ethnopharmacol., 2012, 144, 562–566 CrossRef CAS PubMed.
- G. L. Chen, S. G. Chen, Y. Q. Xie, F. Chen and Y. Q. Gao, J. Funct. Foods, 2015, 17, 243–259 CrossRef CAS.
- M. C. Meurer, M. Mees, L. N. B. Mariano, T. Boeing, L. B. Somensi, M. Mariott, R. Da Silva, A. C. Dos Santos, B. Longo, T. C. Santos Franca, L. C. Klein-Junior, P. De Souza and L. M. Da Silva, Nutr. Res., 2019, 66, 95–106 CrossRef CAS PubMed.
- P. Nowicka and A. Wojdylo, Antioxidants, 2019, 8, 308, DOI:10.3390/antiox8080308.
- P. F. Yang, Y. N. Yang, Z. M. Feng, J. S. Jiang and P. C. Zhang, Bioorg. Chem., 2019, 82, 139–144 CrossRef CAS PubMed.
- C. Nguyen, K. Baskaran, A. Pupulin, I. Ruvinov, O. Zaitoon, S. Grewal, B. Scaria, A. Mehaidli, C. Vegh and S. Pandey, BMC Complementary Altern. Med., 2019, 19, 98 CrossRef PubMed.
- F. J. Li, L. J. Yin, X. Lu and L. T. Li, Int. J. Food Prop., 2010, 13, 512–524 CrossRef CAS.
- W. M. Brand-Williams, M. E. Cuvelier and C. L. Berset, LWT–Food Sci. Technol., 1995, 28, 25–30 CrossRef CAS.
- R. V. D. Berg, G. R Haenen, M. M. Berg and A. Bast, Food Chem., 1999, 66, 511–517 CrossRef.
- I. F. Benzie and J. J. Strain, Anal. Biochem., 1996, 239, 70 CrossRef CAS PubMed.
- S. Mcdonald, P. D. Prenzler, M. Antolovich and K. Robards, Food Chem., 2001, 73, 73–84 CrossRef CAS.
- F. Pourmorad, S. J. Hosseinimehr and N. Shahabimajd, Afr. J. Biotechnol., 2006, 5, 1142–1145 CAS.
- P. Zhang, C. Chang, H. Liu, B. Li, Q. Yan and Z. Jiang, J. Funct. Foods, 2020, 65, 103751 CrossRef CAS.
- M. Guo, X. Chen, Y. Wu, L. Zhang, W. Huang, Y. Yuan, M. Fang, J. Xie and D. Wei, Process Biochem., 2017, 63, 84–95 CrossRef CAS.
- B. Chitrakar, M. Zhang and B. Bhandari, Trends Food Sci. Technol., 2019, 89, 76–87 CrossRef CAS.
- M. Tabaszewska and D. Najgebauer-Lejko, NFS Journal, 2020, 21, 50–56 CrossRef.
- S. Wang, J. Xue, S. Zhang, S. Zheng, Y. Xue, D. Xu and X. Zhang, Plant Physiol. Biochem., 2020, 155, 1–12 CrossRef CAS PubMed.
- N. Sato, W. Li, M. Tsubaki, K. Higai, M. Takemoto, T. Sasaki, T. Onoda and K. Suzuki, J. Funct. Foods, 2017, 35, 159–165 CrossRef CAS.
- T. Pires, M. I. Dias, L. Barros, R. C. Calhelha, M. J. Alves, M. Oliveira and C. Santos-Buelga, Food Res. Int., 2018, 105, 580–588 CrossRef CAS PubMed.
- T. Behl, S. Bungau, K. Kumar, G. Zengin, F. Kha, A. Kumar, R. Kaur, T. Venkatachalam, D. M. Tit, C. M. Vesa, G. Barsan and D. E. Mosteanu, Biomed. Pharmacother., 2020, 130, 110714 CrossRef CAS PubMed.
- A. I. Gostin and V. Y. Waisundara, Trends Food Sci.
Technol., 2019, 86, 381–391 CrossRef CAS.
|
This journal is © The Royal Society of Chemistry 2022 |