DOI:
10.1039/D1RA08134A
(Paper)
RSC Adv., 2022,
12, 3394-3401
Polystyrene nanofibers as an effective sorbent for the adsorption of clonazepam: kinetic and thermodynamic studies
Received
6th November 2021
, Accepted 16th January 2022
First published on 26th January 2022
Abstract
Polystyrene (PS) electrospun nanofibers were prepared via electrospinning for the adsorption of clonazepam from aqueous solution. The adsorption conditions such as adsorption time, solution pH and the amount of adsorbent were optimized. The adsorption kinetics and thermodynamic properties of clonazepam on PS nanofibers were studied under optimized conditions. The pseudo-second-order kinetic model can fit well the adsorption process of clonazepam on polystyrene nanofibers, indicating that the diffusion process in the fiber is the rate-limiting step of the adsorption process. The adsorption equilibrium data are in accordance with the Freundlich isotherm model, and the maximum adsorption capacity is 3.2 mg g−1. Thermodynamic studies revealed that the adsorption process is endothermic and spontaneous in nature. It was suggested that PS electrospun nanofibers have good potential for the separation and purification of clonazepam from a water-soluble matrix as a novel effective adsorbent material.
1. Introduction
Benzodiazepines (BZDs) are a kind of psychoactive substance that produce sedative, hypnotic, anxiolytic, and anticonvulsant activities by enhancing the effect of gamma-aminobutyric acid (γ-GABA) on GABA receptors.1 Clonazepam is a classic BZD used to treat epileptic seizures, insomnia, and anxiety disorders. However, due to its low price and easy availability, clonazepam has become a more commonly abused drug, whether in medical or recreational settings.2 Moreover, criminals have illegally added it to beverages for recreational use to lure young people into drug addiction. Clonazepam alone or in combination with other psychoactive substances may have adverse health effects, such as cognitive and motor disorders, increased anxiety, and sleep disorders. Long-term use of clonazepam may lead to physical dependence and tolerance.2 There is, therefore, a need to develop an effective analysis method for guiding the rational use of clonazepam, treating poisoned patients, or assisting the criminal investigation of crimes.
The matrix of biological samples, such as blood or urine, is complex, and the target substances are often in trace amounts. Thus, an efficient pretreatment method is required to separate and purify them from the matrix before instrumental analysis. The importance of sample pretreatment is reflected in its significant influence on the sensitivity, accuracy, and precision of the qualitative or quantitative analysis.3 It is the key factor that determines the reliability of the analysis results and even determines the feasibility of subsequent analyses. Among various sample pretreatment methods, solid-phase extraction (SPE) is the most used technique. The concept of SPE is based on the distribution of analytes between liquid and solid adsorbents. SPE enriches the analyte from the solution by adsorbing the target compound on the solid adsorbent. The core of solid-phase extraction technology is the adsorption medium.4,5 The proper selection of adsorbents can improve the selectivity, adsorption capacity, and efficiency of the SPE program. The most common classical adsorbents in the SPE process include chemically bonded silica gel with different functional groups, organic polymer adsorbents, and carbon-based materials.6,7 In recent years, nanofibers have gradually become a new SPE adsorption material.8–12 The high specific surface area of nanofibers can provide a large number of active sites, which allows for efficient separation, extraction, and enrichment. Polystyrene nanofibers have been widely used in food detection and the enrichment of environmental pollutants13–15 because of their excellent adsorption capacity.
In this paper, PS nanofibers were prepared by electrospinning, and the adsorption behavior of the PS nanofibers to clonazepam was studied, including its kinetic and thermodynamic properties. Clonazepam was selected as the representative BZD drug in this work. The results of this study will be used to evaluate the feasibility of the efficient extraction and separation of BZDs from biological samples by using PS electrospun nanofibers as an adsorption medium for SPE. It was suggested that PS electrospun nanofibers had good potential for the separation and purification of BZDs from the water-soluble matrix as a novel effective sorbent material.
2. Material and methods
2.1. Materials
The standard of clonazepam (purity 100%) was provided by National Institutes for Food and Drug Control (Beijing, China). Methanol (HPLC-grade) was purchased from Fisher Scientific (Fair Lawn, NJ, USA). PS raw material (MW 230000) was acquired from Shijiazhuang Chemical Fiber Co., Ltd, China. And N,N-dimethylformamide (DMF), tetrahydrofuran (THF), hydrochloric acid, and sodium hydroxide were analytical-grade from Sinopharm Chemical Reagent Co.,Ltd, China.
2.2. Spinning of PS nanofibers
PS nanofibers were prepared by SS-2535 electrospinning apparatus (Beijing Ucalery, China) at 25 °C and 35% humidit. The 15wt% PS solution was prepared by stirring 1.5 g of PS raw material with 10 mL THF–DMF at room temperature for 4 h. The spinning solution was put into the syringe, and the spinneret is connected to the positive pole of the power supply. The aluminum foil is used as the fiber collection screen, which is connected to the negative electrode. The distance between the needle and the collecting screen is 300 mm, the voltage of the spray needle and the collecting screen were set to +16 kV and −7 kV respectively, the spinning speed is 0.08 mm min−1, and the electrospinning time is 8–12 h. The prepared PS nanofibers are washed sequentially with methanol, water, and methanol, and dried at 40 °C. PS nanofibers were characterized by scanning electron microscope (SEM, Hitachi S-4800 I, Tokyo, Japan). A surface area and porosity analyzer (ASAP 2460, Micromeritics Instrument Corporation, USA) was used to determine the Brunauer–Emmett–Teller (BET) of the PS nanofibers.
2.3. Instrument and analytical conditions ions
Waters ACQuity UPLC® H-Class ultra high performance liquid chromatography systems (Waters, Milford, MA, USA) were used for the determination of clonazepam. The LC separation of the compounds was performed on an ODS column (150 mm × 4.6 mm, 5 μm) at 40 °C, with a mobile phase of methanol and water (70
:
30, v/v) at a flow rate of 0.5 mL min−1. The injection volume was 10 μL. The compound was detected by PDA at 254 nm.
2.4. Adsorption experiments
The adsorption test was carried out to quantify the properties of the PS nanofibers. In this experimental study conducted under batch conditions, the effects of different parameters, such as time, pH, initial concentration of adsorbate, and amount of adsorbent in the adsorption of clonazepam, were examined.
2.4.1 Effect of sample solution pH. First, 20 mL aliquots of clonazepam aqueous solution with a concentration of 20 mg L−1 were added to five 50 mL Erlenmeyer flasks. The pH of each solution was adjusted to 3, 5, 7, 9, and 11, respectively. Then, 20 mg of PS nanofibers was added to each Erlenmeyer flask and oscillated at 120 rpm at 25 °C until the adsorption equilibrium was reached. Afterward, 0.1 mL was sampled, and the concentration of clonazepam was determined. The adsorption capacity was calculated, and the effect of pH on the adsorption of clonazepam was investigated.
2.4.2 Effect of amount of adsorbent. To eight 50 mL Erlenmeyer flasks, 20 mL aliquots of clonazepam aqueous solution with a concentration of 20 mg L−1 were added and adjusted to the appropriate pH value. Then, 2.4, 5.2, 8.9, 9.2, 20, 31, 45, and 54 mg of PS nanofibers were added to each flask, respectively, and oscillated at a rate of 120 rpm at 25 °C until the adsorption equilibrium was reached. Afterward, 0.1 mL was sampled, and the concentration of clonazepam was determined. The adsorption capacity was calculated, and the influence of the amount of adsorbent on the adsorption efficiency of clonazepam was investigated.
2.4.3 Effect of the adsorption time and initial concentration on the adsorption of clonazepam. First, 20 mL aliquots of clonazepam aqueous solution with initial concentrations of 4, 8, 12, 16, and 20 mg L−1 were prepared in separate Erlenmeyer flasks. After adjusting to the appropriate pH value, 20 mg of PS nanofibers was added to each flask, and the oscillation rate was 120 rpm at 25 °C. At 0, 5, 10, 20, 30, 40, 50, 60, 90, and 120 min, 0.1 mL was sampled to measure the concentration of clonazepam and calculate the adsorption capacity at different times to determine the effect of adsorption time and initial concentration on the adsorption ability of clonazepam. Vegetable oil, fruit, and tea samples were purchased from local supermarkets of Shijiazhuang. For fruit, the edible part of sample was uniformly crushed using a proofer and placed in a closed container, then it was stored in a refrigerator at 4 °C away from light. The tea sample was ground in a mortar and placed in a clean self-sealing bag until use. Blank samples were used for validation studies and matrix-matched standard calibrations. Samples for recovery studies were spiked with a known amount of fortification standard and left for 10 min before the extraction.
2.5. Determining the kinetics of adsorption
First, 20 mL aliquots of clonazepam aqueous solution with initial concentrations of 4, 8, 12, 16, and 20 mg L−1 were prepared separately and adjusted to the suitable pH value. Then, 20 mg of PS nanofibers were added to each solution and oscillated at 120 rpm at 25 °C until the adsorption equilibrium was achieved, and the adsorption capacity was calculated. Pseudo-first-order and pseudo-second-order kinetic models were used to fit the kinetic adsorption process.
The expressions of the pseudo-first-order kinetic eqn (1) and the pseudo-second-order kinetic eqn (2) are as follows:16–18
|
log(qe − qt) = log qe − k1t/2.303
| (1) |
|
t/qt = t/qe + 1/k2qe2
| (2) |
where
qt and
qe (mg g
−1) are the clonazepam uptake at time
t and at equilibrium, respectively;
qt and
qe were respectively determined using
eqn (3) and
(4).
k1 (min
−1) and
k2 (g mg
−1 min
−1) are the equilibrium rate constants of pseudo-first-order and pseudo-second-order equations, respectively.
where
C0 is the initial concentration of clonazepam in solution (mg L
−1),
Ce is the equilibrium concentration (mg L
−1),
Ct is the concentration at time
t (mg L
−1),
m is the mass of adsorbent (g), and
V is the volume of solution (L).
2.6. Determining the isotherms of the adsorption of clonazepam
Five 20 mL clonazepam aqueous solutions with initial concentrations of 2, 4, 6, 8, and 10 mg L−1 were prepared simultaneously and adjusted to the suitable pH value. Then, 20 mg of PS nanofibers was added and oscillated at 120 rpm at 10 °C and 25 °C until adsorption equilibrium was reached. The samples were determined by high-performance liquid chromatography, and the adsorption capacity was calculated. The data obtained from thermodynamic adsorption experiments were fitted by the Langmuir and Freundlich isothermal adsorption models. Freundlich and Langmuir isotherm model is shown as eqn (5) and (6):16,19 |
ln qe = ln KF + 1/n × ln Ce
| (5) |
|
Ce/qe = Ce/qmax + 1/b × qmax
| (6) |
where qe is the adsorption capacity of clonazepam per unit mass of PS nanofibers (mg g−1), qmax is the maximum monolayer adsorption capacity (mg g−1), b is the Langmuir constant related to binding energy (L mg−1), and KF and n are the Freundlich constants related to adsorption capacity and adsorption intensity, respectively.
2.7. Determining the thermodynamics of adsorption
The enthalpy (ΔH), entropy (ΔS), and Gibbs free energy change (ΔG) of the thermodynamic parameters were calculated using eqn (7)–(9):20 |
log(1/Ce) = log k0 + (−ΔH/2.303RT)
| (7) |
where R is the universal gas constant (8.314 J mol−1 K−1), T is the absolute solution temperature (K), and k0 is a constant.
3. Results and discussion
3.1. Characterization of the PS nanofibers
A scanning electron microscope (SEM, Hitachi S-4800 I, Tokyo, Japan) was used to characterize the PS nanofibers. Fig. 1 shows the diameter of the fibers was about 400 nm, and the fibers showed uniform thickness and a smooth surface. The Brunauer–Emmett–Teller surface area of PS nanofibers was 2.65 m2 g−1, the pore diameter was 7.93 nm, and the pore volume was 0.0053 cm3 g−1.
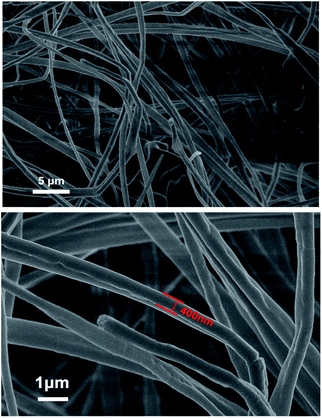 |
| Fig. 1 Typical SEM images of electrospun PS nanofibers. | |
3.2. Adsorption study
3.2.1 Effect of pH. The effect of pH on the adsorption efficiency of clonazepam by PS nanofibers at a pH range of 3.0–11.0 was explored. To adjust the pH, 1 mol L−1 of hydrochloric acid solution or sodium hydroxide solution was used. It was found that clonazepam is unstable under alkaline conditions. When sodium hydroxide solution was added to adjust the pH to 9–11, the clonazepam aqueous solution changed from colorless and transparent to yellow instantly, which indicated that clonazepam had degraded to smaller compounds. When the solutions were adjusted to pH 3–5, the concentration of clonazepam decreased by about 30% and reached 53% after 8 h. Some impurity peaks also appeared on the chromatogram, which indicated that clonazepam had degraded under acidic conditions. Therefore, the final experiment was carried out under neutral conditions.
3.2.2 Effect of initial concentration of clonazepam on its removal by PS nanofibers at various time intervals. The effect of adsorption time on the adsorption capacity at different initial solution concentrations is shown in Fig. 2. It can be seen that the adsorption capacity of clonazepam increased with the adsorption time until equilibrium was reached between the adsorbent and clonazepam solution. In the first 20 minutes, the adsorption capacity of the PS nanofibers for clonazepam increased rapidly, and then gradually slowed down to reach an adsorption equilibrium after 60 min. This may be because, in the early stages of adsorption, the surface of the adsorbent provides a large number of adsorption sites, and the difference in the concentration of clonazepam between the two phases produces a large mass transfer motive force, which causes the rapid complexation of clonazepam during the adsorption process. As the reaction progresses, clonazepam diffuses into the adsorbent until the adsorption equilibrium is reached. The equilibrium adsorption capacity of clonazepam increased from 0.42 to 1.88 mg g−1 as the initial concentration of clonazepam solution increased from 4 to 20 mg L−1. As the initial concentration of clonazepam increased, the adsorption capacity of PS nanofibers to clonazepam also increased, which may be due to increased contact between clonazepam and the adsorbent.
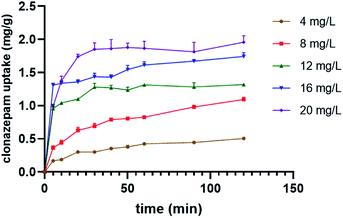 |
| Fig. 2 Time and concentration to the adsorption of clonazepam. | |
3.2.3 Effect of adsorbent dosage. It can be seen from Fig. 3 that as the adsorbent dosage increased, the adsorption capacity per unit mass of clonazepam gradually decreased. This might be because while the total amount of clonazepam in the solution remained unchanged, the number of active sites involved in the adsorption process increases with increasing adsorbent dosage, resulting in a decreasing trend in the adsorption capacity of clonazepam per unit mass of adsorbent. However, as the adsorbent dosage increased, the absolute amount of clonazepam adsorbed also increased. To balance the relationship between the two aspects, the adsorbent mass of 20 mg (1 mg L−1) was chosen for the following experiment.
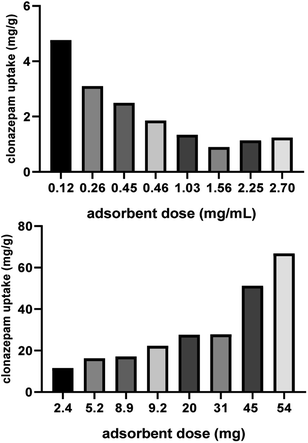 |
| Fig. 3 Effect of adsorbent dosage on clonazepam adsorption on PS nanofibers (T = 293 K, contact time = 60 min, C = 15 mg L−1). | |
3.3. Studying the kinetics of the adsorption process
Adsorption kinetics were utilized to elucidate the controlling mechanism of the adsorption process (e.g., surface adsorption, chemical adsorption, etc.) or the permeation mechanism.21 The study of adsorption kinetics is very useful for predicting the adsorption rate and designing the process model. The adsorption kinetic curves of clonazepam are shown in Fig. 4, and the adsorption kinetic parameters of clonazepam are shown in Table 1. The suitability of the model can be judged by the determination coefficient (R2) of the fitting equation. Compared with the R2 obtained from the two models listed in Table 1, it was found that the adsorption of clonazepam by PS nanofibers was more consistent with the pseudo-second-order kinetic equation. It can be seen from Table 1 that there was good agreement between the theoretical and experimental values of qe. The results show that the rate control step of the adsorption process is the diffusion process in the membrane.22
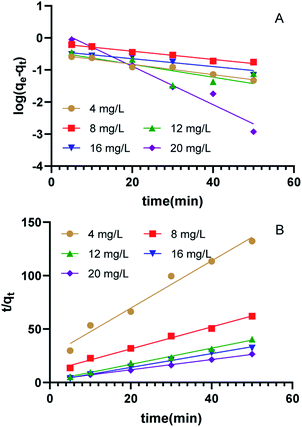 |
| Fig. 4 The linearized plots of pseudo-first order kinetics (A), and pseudo-second order kinetics(B) model for clonazepam adsorption on PS nanofibers. | |
Table 1 Kinetic parameters for adsorption of clonazepam by PS nanofibers
C0 (mg L−1) |
Qexperiment (mg g−1) |
Pseudo-first-order model |
Pseudo-second-order model |
qe (mg g−1) |
k1 (h−1) |
R2 |
qe (mg g−1) |
k2 (g mg−1 h−1) |
R2 |
4 |
0.43 |
0.32 |
0.037 |
0.9637 |
0.45 |
2.50 |
0.9786 |
8 |
0.98 |
0.69 |
0.028 |
0.9758 |
0.97 |
1.01 |
0.9920 |
12 |
1.31 |
0.38 |
0.046 |
0.6321 |
1.32 |
0.76 |
0.9957 |
16 |
1.61 |
0.40 |
0.028 |
0.8058 |
1.55 |
0.60 |
0.9952 |
20 |
1.88 |
2.06 |
0.136 |
0.9657 |
2.09 |
0.60 |
0.9981 |
3.4. The isotherm model of adsorption
The adsorption isotherm was to define the amount of adsorption to the target per unit mass of adsorbent. The Langmuir model assumes homogeneous adsorption, which is used to describe homogeneous monolayer adsorption, and the Freundlich model was used to describe heterogeneous multilayer adsorption. The adsorption isotherms of clonazepam removal by PS nanofibers at 283 K are shown in Table 2 and Fig. 5. The higher determination coefficient (R2) value indicated that the Freundlich model fits the isotherm data better than the Langmuir model. This showed that the adsorption process of clonazepam by PS was multi-layer adsorption, which consists of both surface physical adsorption and internal chemical adsorption. It can be seen that n was greater than 1, indicating that the adsorption process occurred easily.
Table 2 Isotherm parameters for the adsorption of clonazepam by PS nanofibers (T = 283.15 K)
T (K) |
Langmuir |
Freundlich |
Qmax (mg g−1) |
b (L mg−1) |
R2 |
KF [(mg g−1)(L mg−1)] |
n |
R2 |
283.15K |
3.225 |
0.054 |
0.9406 |
0.1827 |
1.238 |
0.9995 |
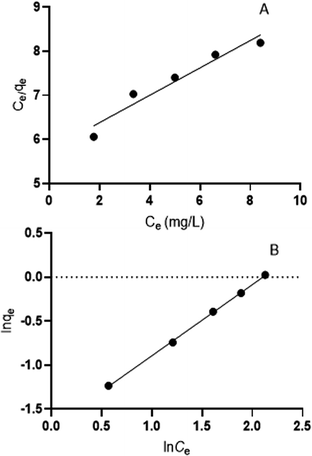 |
| Fig. 5 The Langmuir isotherm model of clonazepam (A), and Freundlich isotherm model of clonazepam adsorption (B). | |
3.5. Effect of temperature on adsorption and thermodynamic parameters
The adsorption behavior of clonazepam on PS nanofibers was studied in the temperature range of 283 to 298 K, from which the thermodynamic parameters such as changes in enthalpy (ΔH, kJ mol−1), entropy (ΔS, kJ mol−1 K−1), and free energy (ΔG, kJ mol−1) could be obtained.
A plot of log(1/Ce) vs. 1/T was created and linearly fitted. According to the slope of the straight line, the change of isometric adsorption enthalpy at a certain adsorption level could be calculated, with its magnitude directly reflecting the nature of the adsorption force.
From Fig. 6 and Table 3, it can be seen that ΔH is positive, indicating that the adsorption process of clonazepam by the PS nanofiber adsorbent was endothermic. ΔG was negative and decreased with increasing temperature, meaning that the adsorption process was spontaneous. Generally speaking, the free energy of physical adsorption is less than that of chemical adsorption, the former in the range of −20–0 kJ mol−1 and the latter in the range of −400 to −80 kJ mol−1.20,23 The value of ΔG in Table 3 shows that the adsorption of clonazepam by PS nanofibers is physical adsorption. ΔS was greater than 0, which showed that the adsorption process led to an increase in entropy.
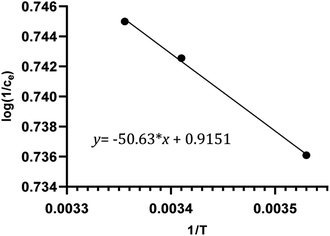 |
| Fig. 6 Plot of log(1/Ce) versus 1/T for the estimation of thermodynamic parameters. | |
Table 3 Thermodynamic parameters for adsorption of clonazepam by PS nanofibers
T (K) |
ΔH (kJ mol−1) |
ΔG (kJ mol−1) |
ΔS (J mol−1 K−1) |
283.15 |
|
−2.91 |
19.6 |
293.15 |
2.64 |
−3.02 |
19.3 |
298.15 |
|
−3.07 |
19.2 |
3.6. Comparison to other reported adsorbents
Commercial SPE cartridges24–26 have been used to extract clonazepam from water or biological samples, and good extraction recoveries have been obtained. However, no adsorption experiment has been done by using commercial SPE cartridges, most of the commercial SPE adsorbents are carbon-based materials with the particle size of the packing is generally micron level, the specific surface area of the material is small, and the adsorption efficiency is generally not high. Three literature27–29 on the adsorption of clonazepam by molecularly imprinted materials were found. The adsorption material is nanometer, and the adsorption capacity is shown in Table 4. It can be seen that the adsorption effect of materials used in this work is the best.
Table 4 Comparison of adsorption capacity by adsorbents in this work and other literaturea
Methods |
Adsorbents |
Adsorption capacity |
Reference |
MIP-SPE: molecularly imprinted polymer solid-phase extraction; MHSPE: mixed hemimicelles-based solid-phase extraction; MIPs-MSB: molecularly imprinted polymers-magnetic stir bar; PS-NFs-based SPE: polystyrene nanofibers-based solid-phase extraction; AGE: allyl glycidyl ether; IDA: iminodiacetic acid; DMAA: N,N-dimethylacrylamide; CTAB: cationic surfactant cetyltrimethylammonium bromide; gf-Fe3O4 NPs: graft-functional Fe3O4 nanoparticles. |
MIP-SPE |
Poly(AGE/IDA-co-DMAA) |
0.18 mg g−1 |
27 |
MHSPE |
CTAB modified Fe3O4/SiO2 nanocomposites |
0.8 mg g−1 |
28 |
MIPs-MSB |
gf-Fe3O4 NPs |
0.27 mg g−1 |
29 |
PS-NF-based SPE |
PS NFs |
3.2 mg g−1 |
This work |
3.7. Adsorption mechanism
Physical adsorption is characterized by low adsorption heat, fast adsorption rate, non-selective, reversible and generally multi-layer adsorption. When the absolute value of adsorption free energy (ΔG) was greater than 40 kJ mol−1, chemical adsorption played an important role; otherwise, physical adsorption is the main process.30,31 In this paper, the value of ΔG were about −3 kJ mol−1, indicating that the adsorption of clonazepam by PS nanofibers may be physical adsorption. The changes of ΔG value (−2.91 to −3.07) with various temperature level is very small (Table 3), which further confirms the physical adsorption characteristics of the adsorption process. The adsorption of clonazepam by PS is multi-layer adsorption and accords with the characteristics of physical adsorption. In addition, we did the adsorption elution experiment by loading clonazepam standard (1 mL) at the concentration of 10 μg mL−1 to 60 mg PS nanofibers, clonazepam was completely eluted by 200 μL methanol, showing that the adsorption–desorption process is reversible. The experimental results support that the adsorption of clonazepam by PS is physical adsorption. Based on the results presented in this paper, we conclude the conclusion that van der Waals forces control the adsorption.
Based on the principle that similar substances are easy to adsorb each other, in general, non-polar adsorbents are suitable for the adsorption of non-polar substances in polar solutions. PS, also known as aromatic adsorbent, is a typical non-polar adsorbent. Fig. 7 shows clonazepam is rich in benzene ring and aromatic ring. The two compounds share the similar structures. This may be the adsorption mechanism for PS adsorbing clonazepam.
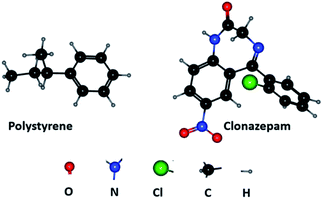 |
| Fig. 7 Structure of polystyrene and clonazepam. | |
Besides, the π–π interaction may also occur when the benzene ring structure of PS and clonazepam interacts with each other. π–π interaction is a general term for the interaction between aromatic molecules with planar structure or aromatic functional groups in organic compounds. The π–π interaction is a kind of non-bonding interaction. PS system is the most common and representative polymer aromatic system. Clonazepam is rich in benzene ring and aromatic ring, and there may be π–π interaction between the two substances.
4. Conclusion
In this paper, the study aimed to determine the detailed adsorption characteristics of novel adsorbents, the adsorption kinetics and thermodynamic properties of clonazepam on PS electrospun nanofibers were studied for the first time, and the feasibility of PS nanofibers for pretreatment of clonazepam and other BZDs was explored. PS nanofibers adsorbed clonazepam quickly in the initial 20 min, and reached the adsorption equilibrium around 60 min. The theoretical adsorption capacity reached 3.2 mg g−1, showing that PS nanofiber is a good adsorbent for clonazepam. The adsorption capacity of clonazepam increased with the increase of adsorbent concentration, and the adsorption of clonazepam by PS nanofibers was more in line with the second-order kinetic model and Freundlich isotherm model. The adsorption processes were found to be naturally feasible, spontaneous, and endothermic based on the thermodynamic parameters. The results showed that PS nanofibers were an efficient adsorbent for the extraction of clonazepam from aqueous solutions and had good potential for separating and purifying BZDs from water-soluble matrices.
Author contributions
Jing An: data curation, formal analysis, methodology, writing – original draft, funding acquisition. Xin Wang: formal analysis, methodology, writing – original draft. Ying Li: data curation. Weijun Kang and Kaoqi Lian: conceptualization, funding acquisition, project administration, resources, Writing – review & editing. All authors read and approved the final manuscript.
Conflicts of interest
The authors declare no conflict of interest.
Acknowledgements
This work was supported by the National Natural Science Foundation of China (Project No. 81872628), and the Hebei Province Science and Technology Support Program (No. 14273002D).
References
- A. N. Bateson, The benzodiazepine site of the GABAA receptor: an old target with new potential?, Sleep Med., 2004, 5, S9–S15 CrossRef PubMed.
- V. Dokkedal-Silva, L. F. Berro, J. Galduróz, S. Tufik and M. L. Andersen, Clonazepam: Indications, Side Effects, and Potential for Nonmedical Use, Harv. Rev. Psychiatr., 2019, 27, 279–289 CrossRef PubMed.
- Z. Qriouet, Z. Qmichou, N. Bouchoutrouch, H. Mahi, Y. Cherrah and H. Sefrioui, Analytical Methods Used for the Detection and Quantification of Benzodiazepines, J. Anal. Methods Chem., 2019, 5, 2035492 Search PubMed.
- R. A. Souza-Silva, R. Jiang, A. Rodríguez-Lafuente, E. Gionfriddo and J. Pawliszyn, A critical review of the state of the art of solid-phase microextraction of complex matrices I. Environmental analysis, Trends Anal. Chem., 2015, 71, 224–235 CrossRef.
- B. Hashemi, P. Zohrabi and M. Shamsipur, Recent developments and applications of different sorbents for SPE and SPME from biological samples, Talanta, 2018, 187, 337–347 CrossRef CAS PubMed.
- K. C. Saunders, A. Ghanem, W. Boon Hon, E. F. Hilder and P. R. Haddad, Separation and sample pre-treatment in bioanalysis using monolithic phases: A review, Anal. Chim. Acta, 2009, 652, 22–31 CrossRef CAS PubMed.
- J. Płotka-Wasylka, N. Szczepańska, M. de la Guardia and J. Namienik, Modern trends in solid phase extraction: New sorbent media, TrAC, Trends Anal. Chem., 2016, 77, 23–43 CrossRef.
- N. G. Jian, S. H. Liang, J. K. Cao, Q. N. Di, K. Kang and Q. Xu, A nanofiber mat prepared from sulfonated polyaniline for solid-phase extraction of fluoroquinolones from water and biological fluids prior to their quantitation by UPLC-MS/MS, Mikrochim. Acta, 2019, 186, 857 CrossRef CAS PubMed.
- O. C. Ifegwu, C. Anyakora, S. Chigome and N. Torto, Application of Nanofiber-packed SPE for Determination of Urinary 1-Hydroxypyrene Level Using HPLC, Anal. Chem. Insights, 2014, 9, 17–25 Search PubMed.
- M. Háková, L. C. Havlíková, F. Švec, P. Solich, J. Erben, J. Chvojka and D. Šatínský, Novel nanofibrous sorbents for the extraction and determination of resveratrol in wine, Talanta, 2020, 206, 120181 CrossRef PubMed.
- M. Háková, L. C. Havlíková, F. Švec, P. Solich and D. Šatínský, Nanofibers as advanced sorbents for on-line solid phase extraction in liquid chromatography: A tutorial. Anal, Chim. Acta, 2020, 1121, 83–96 CrossRef PubMed.
- R. Wang, C. Li, Q. Li, S. Zhang, F. Lv and Z. Yan, Electrospinning fabrication of covalent organic framework composite nanofibers for pipette tip solid phase extraction of tetracycline antibiotics in grass carp and duck, J. Chromatogr. A, 2020, 1622, 461098 CrossRef CAS PubMed.
- D. Qi, X. Kang, L. Chen, Y. Zhang, H. Wei and Z. Gu, Electrospun polymer nanofibers as a solid-phase extraction sorbent for the determination of trace pollutants in environmental water, Anal. Bioanal. Chem., 2008, 390, 929–938 CrossRef CAS PubMed.
- W. Y. Hu, X. J. Kang, C. Zhang, J. Yang, R. Ling, E. H. Liu and P. Li, Packed-fiber solid-phase extraction coupled with high performance liquid chromatography-tandem mass spectrometry for determination of diethylstilbestrol, hexestrol, and dienestrol residues in milk products, J. Chromatogr. B, 2014, 957, 7–13 CrossRef CAS PubMed.
- X. J. Kang, L. Q. Chen, Y. Y. Zhang, Y. W. Liu and Z. Z. Gu, Performance of electrospun nanofibers for SPE of drugs from aqueous solutions, J. Sep. Sci., 2008, 31, 3272–3278 CrossRef CAS PubMed.
- V. K. Gupta, A. Fakhri, S. Agarwal, A. K. Bharti, M. Naji and A. G. Tkachev, Preparation and characterization of TiO2 nanofibers by hydrothermal method for removal of Benzodiazepines (Diazepam) from liquids as catalytic ozonation and adsorption processes, J. Mol. Liq., 2018, 249, 1033–1038 CrossRef CAS.
- F. F. Qi, Y. Cao, M. Wang, F. Rong and Q. Xu, Nylon 6 electrospun nanofibers mat as effective sorbent for the removal of estrogens: kinetic and thermodynamic studies, Nanoscale Res. Lett., 2014, 9, 353 CrossRef PubMed.
- M. Ghaedi, A. Daneshyar, A. Asfarama and K. M. Purkait, Adsorption of naphthalene onto high-surface-area nanoparticle loaded activated carbon by high performance liquid chromatography: response surface methodology, isotherm and kinetic study, RSC Adv., 2016, 6, 54322–54330 RSC.
- A. Fakhri, Investigation of mercury (II) adsorption from aqueous solution onto copper oxide nanoparticles: Optimization using response surface methodology, Process Saf. Environ. Prot., 2015, 93, 1–8 CrossRef CAS.
- G. Luo, Q. X. Zhang, A. M. Li, F. Q. Liu, L. Shao and J. L. Chen, Adsorption and thermodynamic properties of sorbic acid on the polymeric adsorption resins, Chin. J. Appl. Chem., 2003, 20, 1139–1142 CAS.
- A. Fakhri, S. Rashidi, M. Asif, I. Tyagid, S. Agarwalde and V. K. Gupta, Dynamic adsorption behavior and mechanism of Cefotaxime, Cefradine and Cefazolin antibiotics on CdS-MWCNT nanocomposites, J. Mol. Liq., 2016, 215, 269–275 CrossRef CAS.
- H. K. Boparai, M. Joseph and D. M. O'Carroll, Kinetics and thermodynamics of cadmium ion removal by adsorption onto nano zerovalent iron particles, J. Hazard. Mater., 2011, 186, 458–465 CrossRef CAS PubMed.
- C. C. Liu, M. Kuang-Wang and Y. S. Li, Removal of nickel from aqueous solution using wine processing waste sludge, Ind. Eng. Chem. Res., 2005, 44, 1438–1445 CrossRef CAS.
- F. G. de Araujo, G. FBauerfeldt, M. Marques and E. M. Martins, Development and Validation of an Analytical Method for the Detection and Quantification of Bromazepam, Clonazepam and Diazepam by UPLC-MS/MS in Surface Water, Bull. Environ. Contam. Toxicol., 2019, 103, 362–366 CrossRef CAS PubMed.
- I. Álvarez-Freire, P. Brunetti, P. Cabarcos-Fernández, A. Fernández-Liste, M. J. Tabernero-Duque and A. M. Bermejo-Barrera, Determination of benzodiazepines in pericardial fluid by gas chromatography-mass spectrometry, J. Pharm. Biomed. Anal., 2018, 159, 45–52 CrossRef PubMed.
- J. An, H. J. Song, X. Wei and Z. J. Dong, Simultaneous determination of nine kinds of sedativehypnotics in the plasma using solid phase extraction coupled with UPLC-MS/MS, West China J. Pharm. Sci., 2018, 33, 72–76 Search PubMed.
- H. A. Panahi, A. Mehramizi, S. Ghassemi and E. Moniri, Selective extraction of clonazepam from human plasma and urine samples by molecularly imprinted polymeric beads, J. Sep. Sci., 2014, 37, 691–695 CrossRef CAS PubMed.
- E. Esmaeili-Shahri and Z. Es'haghi, Superparamagnetic Fe3 O4 @SiO2 core-shell composite nanoparticles for the mixed hemimicelle solid-phase extraction of benzodiazepines from hair and wastewater samples before high-performance liquid chromatography analysis, J. Sep. Sci., 2015, 38, 4095–4104 CrossRef CAS PubMed.
- X. Li, X. Mei, L. Xu, X. Shen, W. Zhu, J. Hong and X. Zhou, Development and application of novel clonazepam molecularly imprinted coatings for stir bar sorptive extraction, J. Colloid Interface Sci., 2016, 468, 183–191 CrossRef CAS PubMed.
- Z. Zhang, Q. Gao, J. Yang, Y. Li, J. Liu, Y. Wang, H. Su, Y. Wang, S. Wang and G. Feng, The adsorption and mechanism of the nitrification inhibitor nitrapyrin in different types of soils, R. Soc. Open. Sci., 2020, 7, 200259 CrossRef CAS PubMed.
- J. Zhang, J. Wang, C. Zhang, Z. Li, J. Zhu and B. Lu, Molecular simulation of gases competitive adsorption in lignite and analysis of original CO desorption, Sci. Rep., 2021, 11, 11706 CrossRef CAS PubMed.
|
This journal is © The Royal Society of Chemistry 2022 |
Click here to see how this site uses Cookies. View our privacy policy here.