DOI:
10.1039/D1RA06276J
(Paper)
RSC Adv., 2022,
12, 3630-3634
Synthesis of functional dicationic ionic liquids for the treatment of lignocellulosic biomass
Received
19th August 2021
, Accepted 13th December 2021
First published on 27th January 2022
Abstract
Mono-cationic ionic liquids (MILs) have been widely utilized for the treatment of lignocelluloses. However, the hydrophilic property of MILs increases the difficulty of isolating sugars and their reuse, which limits their industrial application. In the present study, a series of dicationic ionic liquids (DILs) were synthesized with totally different di-cations and di-anions. An alternating miscibility of DILs with water was observed depending on the dealing temperature. The functional DILs exhibiting hydrophobic and acidic properties were investigated for the treatment of corn stalk. High yields of sugars were achieved as 27.8% and 31.6% with DIL3 and DIL4, respectively. Cellulosic materials were obtained as 74.3% and 77.8% with DIL1 and DIL2 and 61.2% and 65.7% with DIL3 and DIL4, respectively. Enzymatic reaction was successfully performed with cellulosic materials after the DIL treatment. DILs were recycled and reused with no significant reduction in sugar yields. Hydrophobic DILs are a potentially effective approach to enable the successful decomposition and degradation of lignocellulosic biomass.
Introduction
Lignocellulosic biomass has been identified as a renewable source for generating biofuels and bio-products. Lignocelluloses comprise cellulose, hemicelluloses and lignin, forming a recalcitrant network.1,2 In general, pretreatment is required to destroy the compact structure of lignocellulosic biomass to make it available to enzymatic hydrolysis to achieve complete conversion into valuable chemicals.
Ionic liquids (ILs) are considered as an effective approach in the pretreatment of lignocelluloses.3 ILs are environmentally friendly due to a variety of favourable properties including high thermal stability, low vapour pressure, non-flammable and reusable.4 In addition, ILs are capable of destroying the cross-linking among the lignocellulosic polymers and reducing the cellulose crystalline structure by forming hydrogen bonds. Herein, the IL pretreatment could improve enzymatic saccharification for the complete degradation of lignocelluloses.5,6
Mono-cationic ILs (MILs) are reported widely for the treatment of lignocelluloses. In 2002, Rogers et al. found the dissolution of cellulose in ILs with the cation of 1-butyl-3-methylimidazolium ([BMIM]) and the anions Cl−, Br−, or SCN− at 100 °C.7 Consequently, a variety of MILs have been reported, such as imidazolium, pyridinium, ammonium, phosphonium, and cholin-based cations, coupled to a number of anions, such as chloride, bromide, acetate, or phosphonate. It has been reported that 1-allyl-3-methylimidazolium chloride ([AMIM]Cl), 1-ethyl-3-methylimidazolum acetate ([EMIM]CH3COO) and [BMIM]Cl are extensively used as effective pretreated solvents for lignocelluloses.8,14 Moreover, functional acidic MILs including hydroxyalkylimidazolium hydrogen sulphate and 1-(sulfobutyl)-3-methylimidazolium chloride have been employed as both catalysts and solvents to degrade lignocelluloses.9,10 However, these MILs with Cl− or CH3COO− as the anions are completely water-miscible.21
Dicationic ionic liquids (DILs) are classified into symmetrical DILs, with two identical cations and unsymmetrical DILs, with two different cations. In general, the miscibility of ionic liquids with water depends much on their anions. MILs are water-immiscible with the anions including PF6−or NTf2−, whereas MILs are water-miscible with the anions involving Cl− or Br−. Our previous study showed that the miscibility of DILs in water could be altered by the combination of a hydrophobic anion (PF6−) and a hydrophilic anion (Cl−).11 However, in current studies, there is very limited information concerning the application of DILs. It is therefore necessary to investigate DILs for the treatment of lignocellulosic biomass.
In this study, a series of novel functional DILs were synthesized. The miscibility of DILs with water or various organic solvents was measured. DILs were investigated for the treatment of corn stalk. Reducing sugar and cellulosic materials were measured during DIL treatments. Enzymatic saccharification was performed for the cellulosic materials after DIL treatment.
Results
Preparation of dicationic ionic liquids
A series of dicationic ionic liquids (DILs) were synthesized with totally different dications and dianions; for instance, the cation involving imidazolium and pyridinium, coupled to a hydrophobic anion (PF6−) and a hydrophilic anion (Br−) (Fig. 1). The PF6− group improves the immiscibility of DILs with water. The Br− group promotes the intrinsic interaction of ILs with lignocelluloses. The carboxyl group assists the hydrolysis of lignocelluloses. In addition, the imidazolium cation was introduced into the dications of DILs because the imidazolium ring could particularly promote lignocellulose dissolution.12 The pyridinium cation was introduced into the dications of DILs because pyridinium-based ILs were observed to generate poor solubility with water.19 In this study, these DILs exhibiting hydrophobic or acidic properties could be potentially utilized in various applications.
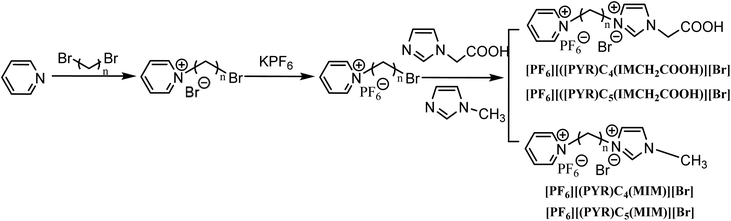 |
| Fig. 1 Synthesis of dicationic ionic liquids. | |
Miscibility of DILs with organic solvents
The solubilities of the DILs were determined in several representative solvents at room temperature. Good solubility of DILs was achieved in polar solvents such as dimethyl sulfoxide and acetonitrile. Poor solubility of DILs was observed in non-polar solvents such as ethyl acetate, dichloromethane and diethyl ether. Particularly, DILs showed poor solubility in methanol and ethanol (Table 1).
Table 1 Solubility of DILs with organic solventsa
|
DMSO |
MeOH |
EtOH |
CH3CN |
EtOAc |
CH2Cl2 |
Et2O |
DIL1: [PF6][(PYR)C4(MIM)][Br]; DIL2: [PF6][(PYR)C5(MIM)][Br]; DIL3: [PF6][(PYR)C4(IMCH2COOH)][Br]; DIL4: [PF6][(PYR)C5(IMCH2COOH)][Br]; “++”: 0.1 g ionic liquid was easily dissolved in one of the organic solvents (0.1–1 mL). “+”: 0.1 g ionic liquid was dissolved in one of the organic solvents (1–10 mL). “−”: 0.1 g ionic liquid was not dissolved in one of the organic solvents (10 mL). |
DIL1 |
+ |
− |
− |
+ |
− |
− |
− |
DIL2 |
+ |
− |
− |
+ |
− |
− |
− |
DIL3 |
+ |
− |
− |
+ |
− |
− |
− |
DIL4 |
+ |
− |
− |
+ |
− |
− |
− |
Miscibility of DILs with water
Commonly, MILs with the anions including PF6− or NTf2− are water-immiscible, whereas MILs with the anions involving Cl− or Br− are water-miscible. Remarkably, the miscibility of DILs with water was dependent on the dealing temperature. At high temperatures, the miscibility of DILs with water was better. A small amount of water was necessary at 80–100 °C. 1.0 g of the DILs was miscible with 1–5 mL of water at 100 °C. However, when the temperature was reduced to room temperature, a large amount of water was required, and 145 mL and 45 mL of water were necessary for DIL1 and DIL2, respectively. The order of water-miscibility is DIL4 > DIL3 > DIL2 > DIL1.
As the linkage chain of dications increased, the miscibility of DILs with water was expected to decrease. Nevertheless, good miscibility was observed with DIL2 and DIL4 compared with DIL1 and DIL3 due to the low symmetry of the linkage chains of these DILs. Due to the introduction of carboxyl groups, DIL3 and DIL4 exhibited higher water-miscibility compared with DIL1 and DIL2. Recent studies have shown that most functional MILs are hydrophilic, which increases the difficulty for their recovery and reuse. The functional DILs in this study may provide a new approach for the treatment of lignocelluloses (Fig. 2).
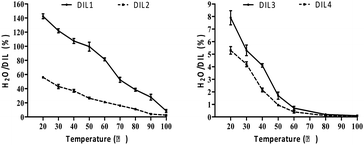 |
| Fig. 2 Miscibility of DILs with water. DIL1: [PF6][(PYR)C4(MIM)][Br]; DIL2: [PF6][(PYR)C5(MIM)][Br]; DIL3: [PF6][(PYR)C4(IMCH2COOH)][Br]; DIL4: [PF6][(PYR)C5(IMCH2COOH)][Br]. | |
Determination of reducing sugars
The constituents of raw corn stalk (21.32% lignin, 20.39% hemicelluloses, 42.73% cellulose and 0.79% ash) were determined in accordance with the National Renewable Energy Laboratory (NREL) method.13 The yields of reducing sugars and cellulosic residues during the DILs-treatment were determined according to eqn (1).
When corn stalk was treated with DIL3 and DIL4, high yields of reducing sugars were achieved, 27.8% and 31.6%, respectively, indicating the hydrolysis of cellulose and hemicelluloses (Fig. 3). Moreover, the yield of reducing sugars was 5.6% and 6.2% when corn stalk was treated with DIL1 or DIL2, respectively. Therefore, the acidity of DILs assisted in hydrolyzing most of hemicelluloses and partial cellulose to achieve the efficient hydrolysis of corn stalk.
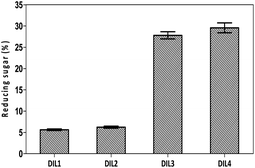 |
| Fig. 3 Yields of reducing sugars. DIL1: [PF6][(PYR)C4(MIM)][Br]; DIL2: [PF6][(PYR)C5(MIM)][Br]; DIL3: [PF6][(PYR)C4(IMCH2COOH)][Br]; DIL4: [PF6][(PYR)C5(IMCH2COOH)][Br]. | |
Determination of DIL-treated residues
After the DIL treatment, the yields of cellulosic residues were obtained in the range of 61–78% (Fig. 4). Particularly, a yield of 74.3–77.8% of cellulosic materials was achieved using DIL1 and DIL2. A low yield of cellulosic materials (61.2–65.7%) was observed with treatment with DIL3 and DIL4. This could be attributed to the effectiveness of DIL3 and DIL4 to decompose and hydrolyze the corn stalk.
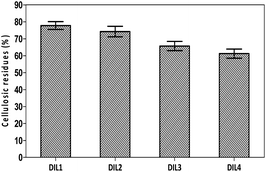 |
| Fig. 4 Yields of cellulosic residues. DIL1: [PF6][(PYR)C4(MIM)][Br]; DIL2: [PF6][(PYR)C5(MIM)][Br]; DIL3: [PF6][(PYR)C4(IMCH2COOH)][Br]; DIL4: [PF6][(PYR)C5(IMCH2COOH)][Br]. | |
Enzymatic saccharification
Enzymatic hydrolysis of cellulosic residues was studied by measuring the total reducing sugars. The yields of sugars were calculated according to eqn (2). After 72 h of the enzymatic reaction, high yields of reducing sugars were obtained in the range of 70.1–91.0% (Fig. 5), and a medium to high yield of glucose was achieved (55.7–77.9%).
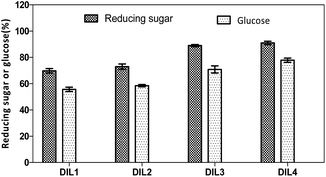 |
| Fig. 5 Yields of reducing sugars and glucose. DIL1: [PF6][(PYR)C4(MIM)][Br]; DIL2: [PF6][(PYR)C5(MIM)][Br]; DIL3: [PF6][(PYR)C4(IMCH2COOH)][Br]; DIL4: [PF6][(PYR)C5(IMCH2COOH)][Br]. | |
It was reported that cotton stalk was pretreated at 150 °C for 30 min in [EMIM]CH3COO to release 65% of reducing sugars after 72 h of enzymatic hydrolysis.14 Rice straw was pretreated by cholinium argininate at 90 °C for 12 h to afford 74.6% of glucose after 72 h of enzymatic hydrolysis.15 Particularly, high yields of reducing sugars and glucose were obtained with DIL3 and DIL4, which is possibly due to the effective decomposition of lignocelluloses by the DILs to enable a successful enzymatic hydrolysis.
Reuse of dicationic ionic liquids
DILs were recycled and reused for the determination of sugar analysis (Fig. 6). Sugar yield using fresh DIL treatment (1st run) is considered to be 100%.
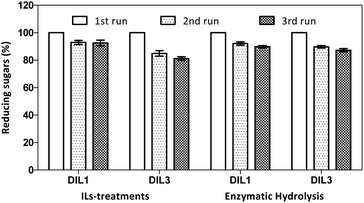 |
| Fig. 6 Yields of sugars using reused DILs. DIL1: [PF6][(PYR)C4(MIM)][Br]; DIL3: [PF6][(PYR)C4(IMCH2COOH)][Br]. | |
During the DIL treatment of corn stalk, the sugar rates were 92–93% with the DIL1 treatment. The sugar rates were 81–84% with the DIL3 treatment. No significant difference was observed when recycled DILs were used during the DIL treatment.
The reused ILs were further evaluated during the enzymatic hydrolysis of DIL-treated residues (Fig. 6). The sugar rates were 90–92% for the DIL1 treatment, and 87–90% for the cellulosic materials obtained from the DIL3 treatment. Therefore, no obvious difference was found for enzymatic reactions.
Herein, different anions play a critical role in the miscibility of DILs with water. DILs exhibit both hydrophilicity due to Br− and hydrophobicity due to PF6−, leading to their alternating water-miscibility. Moreover, the miscibility of DILs with water depends on the dealing temperature. The low symmetry of DILs could promote the water-miscibility of DILs. In addition, an introduction of a carboxyl group could provide the acidic nature of DILs.
The functional DILs are responsible for an efficient disruption of the compact structure of lignocelluloses. Ionic liquids with anions such as Br−, Cl−, and CH3SO3− are effective in weakening the hydrogen-bonding network of the polymer chains.16,17 Hence, the anion (Br−) of DILs enables the disruption of inter and intra-molecular hydrogen bonding in polymers. Moreover, the imidazolium cations of DILs particularly interact with the aromatic rings of lignin through π–π interactions to promote lignin dissolution.18 A carboxyl group in the cations of DILs assists to destruct the intrinsic link of lignocelluloses and promote the hydrolysis of hemicelluloses and cellulose. Therefore, the hydrogen bond of bromide and the acidic activity of the carboxyl group contribute to the effective treatment of lignocelluloses and high enzymatic digestibility.
Materials and methods
Sample collection
Corn stalk was supplied by a local farm in Harbin City (China). The corn stalk was milled to pass through 40-mesh sieves. All the chemicals including 1,4-dibromobutane (99%), 1,5-dibromopentane (99%), pyridine (99%), 1H-imidazole-1-acetic acid (99%), 1-methylimidazole (99%) and potassium hexafluorophosphate (99%) were purchased from Aldrich Reagent Co., Ltd (Shanghai, China).
Synthesis of dicationic ionic liquids
Pyridine (1.0 mmol) and 1, 4-dibromobutane (1.0 mmol) were reacted at 60 °C for 4 days. The resulting product was purified by silica gel column chromatography elution with CH2Cl2/MeOH (3/1) and vacuum-dried at 60 °C for 4 h. The product (1.0 mmol) was reacted with KPF6 (1.0 mmol) in deionized water at room temperature for 4 h to give [(PYR)C4Br][PF6], which was vacuum-dried for 4 h. [(PYR)C4Br][PF6] (0.1 mmol) and 1-methylimidazole (0.1 mmol) were reacted in ethyl acetate at 60 °C for 24 h. The product was then dried under vacuum at 60 °C for 4 h to afford hexafluorophosphate [1-(1-pyridinium-yl-butyl)-4-methylimidazolium] bromide ([PF6][(PYR)C4(MIM)][Br]). A similar protocol was employed for the preparation of hexafluorophosphate [1-(1-pyridinium-yl-pentyl)-4-methylimidazolium] bromide ([PF6][(PYR)C5(MIM)][Br]).11
[PF6][(PYR)C4(MIM][Br]: 1H-NMR (400 MHz, DMSO-d6) (ppm): 9.08 (m, 2H), 9.06 (m, 1H), 8.64 (t, 1H), 8.19 (t, 2H), 7.74 (s, 1H), 7.71 (s, 1H), 4.63 (t, 2H), 4.22 (t, 2H), 3.85 (s, 3H), 1.92 (q, 2H), 1.83 (q, 2H). MS ESI (m/z) = 362[(PYR)C4(MIM][PF6].
[PF6][(PYR)C5(MIM)][Br]: 1H-NMR (400 MHz, DMSO-d6) (ppm): 9.06 (m, 2H), 9.02 (m, 1H), 8.61 (m, 1H), 8.17 (m, 2H), 7.74 (s, 1H), 7.70 (s, 1H), 4.59 (t, 2H), 4.19 (t, 2H), 3.85 (s, 3H), 1.96 (m, 2H), 1.83 (m, 2H), 1.25 (m, 2H). MS ESI (m/z) = 376[(PYR)C5(MIM][PF6].
[(PYR)C4Br][PF6] (0.1 mmol) and 1H-imidazole-1-acetic acid (0.1 mmol) were reacted in an aqueous solution at 80 °C for 96 h. After that, water was removed by rotary evaporation at 60 °C for 4 h. The resulting product was purified by column chromatography on silica. After the removal of solvents, the product was dried under vacuum at 60 °C for 4 h to give hexafluorophosphate [1-(1-pyridinium-yl-butyl)-4-(imidazolium-1-aceticacid)]-1H-bromide ([PF6][(PYR)C4(IMCOOH)][Br]). A similar protocol was employed for the preparation of hexafluorophosphate [1-(1-pyridinium-yl-pentyl)-4-(imidazolium-1-aceticacid)]-1H-bromide ([PF6][(PYR)C5(IMCOOH)][Br]).
[PF6][(PYR)C4(IMCH2COOH)][Br]: 1H-NMR (400 MHz, DMSO-d6) (ppm): 9.13 (m, 2H), 8.73 (m, 1H), 8.62 (m, 1H), 8.18 (m, 2H), 7.58 (s, 1H), 7.47 (s, 1H), 5.06 (s, 2H), 4.64 (t, 2H), 4.17 (t, 2H), 1.96 (m, 2H), 1.41 (m, 2H). MS ESI (m/z) = 406 [(PYR)C4(IMCOOH)][PF6].
[PF6][(PYR)C5(IMCH2COOH)][Br]: 9.19 (d, 1H), 9.16 (d, 1H),8.79 (m, 1H), 8.64 (m, 1H), 8.19 (m, 2H), 7.61 (s, 1H), 7.51 (s, 1H), 5.07 (s, 2H), 4.66 (m, 2H), 4.14 (m, 2H), 1.94 (m, 2H), 1.45 (m, 2H), 1.29 (m, 2H). MS ESI (m/z) = 420[(PYR)C5(IMCOOH)][PF6].
Miscibility of DILs with solvents
Each of the DILs (0.1 g) was mixed with an appropriate amount of an organic solvent at room temperature. Each DIL (0.1 g) was mixed with an appropriate amount of water at different temperatures. The mixture was shaken vigorously every 5 min for 30 s. If the mixture was immiscible within 30 min, an appropriate amount of the solvent was added into the mixture until the mixture became a clear solution.
Analysis of corn stalk
300 mg of the powdered corn stalk was mixed with 3 mL of 72% (w/w) H2SO4. The mixture was stirred vigorously every 10 min at 30 °C for 60 min. Thereafter, 84 mL of deionized water was added into the mixture, corresponding to 4% (w/w) H2SO4 aqueous solution. The mixture was taken into an autoclave at 121 °C for 60 min. After that, the mixture was centrifuged to afford the filtrate, which was determined for glucose and xylose using HPLC analysis (Agilent Technologies, Santa Clara, CA, USA). Acid soluble lignin was determined using a UV-mini-1240 spectrometer (Shimadzu Corp., Tokyo, Japan). The filtered solids were then taken into a muffle furnace at 575 °C to afford the acid-insoluble lignin and ash. The content of cellulose and hemicellulose was calculated from glucose and xylose by conversion factors of 0.90 and 0.88, respectively.13
DILs-treatment of corn stalk
Corn stalk (200 mg) was added into DILs (4 g) and deionized water (3 mL). The mixture was stirred at 100 °C for 5 h in a reaction kettle in an oil bath. After that, 10 mL of deionized water was added into the mixture. The solid was filtered to afford DIL-treated residues. The filtrate was measured for reducing sugar according to the dinitrosalicylic acid (DNS) method.20 Sugar analysis was conducted on an UV-mini-1240 spectrometer (Shimadzu Corp., Tokyo, Japan). Each experiment was conducted in triplicate. The yields of reducing sugar and cellulosic residues were determined as the ratio of the mass of reducing sugar in the mass of the corn stalk that was subjected to the DIL treatment according to eqn (1). |
 | (1) |
Enzymatic hydrolysis
Enzymatic reaction was performed with 1% (g ml−1) of corn stalk and 0.02 g of cellulase in 0.1 M sodium citrate buffer (pH 4.8) on the shaker at 150 rpm at 50 °C for 72 h. The enzymatic reaction was quenched at 100 °C for 10 min. The reducing sugar was measured by DNS method using an UV-mini-1240 spectrometer (Shimadzu Corp., Tokyo, Japan). The yields of glucose was measured on an Agilent 1100 HPLC instrument equipped with an Agilent ZORBAX carbohydrate column (Agilent Technologies, Santa Clara, CA, USA). Each experiment was conducted in triplicate. The yields of reducing sugar and glucose were determined as the ratio of the mass of reducing sugar or glucose in the mass of the residues that was subjected to enzymatic hydrolysis according to eqn (2). |
 | (2) |
Recycle and reuse of ionic liquids
After the mixture was cooled to 4 °C, DIL1 precipitated out of the solution and was filtered for reuse. Alternatively, 20 mL of acetonitrile was used to extract DILs after the removal of water from the mixture. Acetonitrile was then evaporated to provide DILs. DILs were dried in a vacuum at 60 °C for 6 h.
Conclusion
DILs with hydrophobic and acidic nature were prepared. The miscibility of DILs with water varied at different temperatures. High yields of reducing sugar were obtained when corn stalk was treated with DILs having acidic properties. Enzymatic hydrolysis was successfully performed with DIL-treated residues. No significant reduction of sugars was found for the recycled DILs. The combination of the DIL treatment and cellulase enables the effective degradation of corn stalk.
Conflicts of interest
There are no conflicts to declare.
Acknowledgements
Bi-Xian Zhang and Xue-Yang Wang contributed equally to this work. This work was supported by Key Program of Natural Science Foundation of Heilongjiang Province, China (JJ2020ZD0111); Postdoctoral Scientific Research Start-up-Fund of Heilongjiang Province, China (LBH-Q19053); Leading Talent Echelon Backup Leaders Project of Heilongjiang Province, China (First-class Fund of Innovative R & D).
Notes and references
- M. Taha, E. Shahsavari, K. Al-Hothaly, A. Mouradov, A. T. Smith, A. S. Ball and E. M. Adetutu, Appl. Biochem. Biotechnol., 2015, 175, 3709–3728 CrossRef CAS PubMed.
- K. Karimi and M. J. Taherzadeh, Bioresour. Technol., 2016, 203, 348–356 CrossRef CAS PubMed.
- H. T. Tan and K. T. Lee, Chem. Eng. J., 2012, 183, 448–458 CrossRef CAS.
- K. Ababtain, G. Babu, X. Lin, M. T.-F. Rodrigues, H. Gullapalli, P. M. Ajayan, M. W. Grinstaff and L. M. R. Arava, ACS Appl. Mater. Interfaces, 2016, 8, 15242–15249 CrossRef CAS PubMed.
- Z. Y. Zhang, M. D. Harrison, D. W. Rackemann, W. O. S. Doherty and I. M. O'Hara, Green Chem., 2016, 18, 360–381 RSC.
- X. Zhu, C. Peng, H. Chen, Q. Chen, Z. K. Zhao, Q. Zheng and H. Xie, ChemistrySelect, 2018, 3, 7945–7962 CrossRef CAS.
- R. P. Swatloski, S. K. Spear, J. D. Holbrey and R. D. Rogers, J. Am. Chem. Soc., 2002, 124, 4974–4975 CrossRef CAS PubMed.
- X. Gao, R. Kumar, S. Singh, B. A. Simmons, V. Balan, B. E. Dale and C. E. Wyman, Biotechnol. Biofuels, 2014, 7, 71 CrossRef PubMed.
- Y. Dong, J. Holm, J. Karkkainen, J. Nowicki and U. Lassi, Biomass Bioenergy, 2014, 70, 461–467 CrossRef CAS.
- A. S. Amarasekara and P. Shanbhag, BioEnergy Res., 2012, 6, 719–724 CrossRef.
- H. Y. Han, X. Geng, B. X. Zhang, J. Meng, X. Liu, X. M. He, Z. G. Liu, Y. F. Gao, D. liu and X. M. Hu, RSC Adv., 2019, 9, 29652–29658 RSC.
- K. Shill, S. Padmanabhan, Q. Xin, J. M. Prausnitz, D. S. Clark and H. W. Blanch, Biotechnol. Bioeng., 2011, 108, 511–520 CrossRef CAS PubMed.
- A. Sluiter, B. Hames, R. Ruiz, C. Scarlata, J. Sluitter, D. Templeton and D. Crocker, Laboratory Analytical Procedure (LAP), Technical Report NREL/TP-510-42618, National Renewable Energy Laboratory (NREL), U.S. Dept. of Energy, Golden, co, 2011, https://www.nrel.gov/docs/gen/fy13/42618.pdf Search PubMed.
- N. I. Haykir, E. Bahcegul, N. Bicak and U. Bakir, Ind. Crops Prod., 2013, 41, 430–436 CrossRef CAS.
- Y. X. An, M. H. Zong, H. Wu and N. Li, Bioresour. Technol., 2015, 192, 165–171 CrossRef CAS PubMed.
- M. Abe, Y. Fukaya and H. Ohno, Green Chem., 2010, 12, 1274–1280 RSC.
- N. Muhammad, Z. Man, M. A. Bustam, M. I. A. Mutalib, C. D. Wilfred and S. Rafiq, Appl. Biochem. Biotechnol., 2011, 165, 998–1009 CrossRef CAS PubMed.
- M. Jiang, M. M. Zhao, Z. W. Zhou, T. Huang, X. L. Chen and Y. Wang, Ind. Crops Prod., 2011, 33, 734–738 CrossRef CAS.
- Y. X. Sun, Y. Y. Wang, B. B. Shen, B. X. Zhang and X. M. Hu, R. Soc. Open Sci., 2018, 5, 181230 CrossRef CAS PubMed.
- G. L. Miller, Anal. Biochem., 1959, 31, 426–428 CAS.
- P. Zhang, S. J. Dong, H. H. Ma, B. X. Zhang, Y. F. Wang and X. M. Hu, Ind. Crops Prod., 2015, 76, 688–696 CrossRef CAS.
Footnote |
† These authors contributed equally to this work. |
|
This journal is © The Royal Society of Chemistry 2022 |
Click here to see how this site uses Cookies. View our privacy policy here.