DOI:
10.1039/D2QO01120D
(Research Article)
Org. Chem. Front., 2022,
9, 5213-5218
Synthesis of the cyclic heptapeptide core of callipeltin A†
Received
12th July 2022
, Accepted 30th July 2022
First published on 23rd August 2022
Abstract
Macrolactonisation of a novel heptapeptide precursor with PyAOP proved to be an excellent method for preparation of the cyclic depsipeptide core of callipeltin A. The individual building blocks were obtained in high yield and selectivity and successive coupling allowed for the straightforward preparation of the linear heptapeptide from tyrosine 6 in 27% over 12 steps.
Introduction
Callipeltin A (Fig. 1), first isolated in 1996 by Zampella et al. from the marine sponge Callipelta sp. near the east coast of New Caledonia in the Pacific Ocean, is a novel decapeptide that consists of a cyclic heptapeptide core and a highly functionalised sidechain R.1 The side chain contains several complex nonproteinogenic amino acids along with a rare terminal β-hydroxy acid. The natural product has undergone several structural revisions, including the reconfiguration of the β-hydroxy acid,2a determination of the configuration of the β-methoxy tyrosine residue,2b and reassignment of both threonine moieties to D-allo-threonine.2c To date, the callipeltin family includes 17 representatives up to callipeltin Q.2a,3
 |
| Fig. 1 Structure of callipeltin A and B. | |
The callipeltins belong to a class of structurally related marine natural products, such as mirabamides,4 papuamides5 and neamphamides.6 While callipeltin A displays some notable antiviral, antifungal and cytotoxic activity,1,3a,7 the structural complexity of the natural product, in particular, has raised the interest of synthetic chemists. Several research groups reported asymmetric routes toward the amino acid building blocks (2R,3R)-β-methoxy tyrosine,8 (3S,4R)-dimethyl-L-glutamine (diMeGln)9 and (2R,3R,4S)-4-amino-7-guanidino-2,3-dihydroxy-heptanoic acid (AGDHE).10 Additionally, the synthesis of hydroxy acid (2R,3R,4R) 3-hydroxy-2,4,6-trimethylheptanoic acid (TMEHA) was described.11 In 2005 and 2006, Lipton et al. achieved the first synthesis of the callipeltins D, E and B via solid-phase peptide synthesis.12a–c More recently, Konno et al. reported a similar route toward callipeltin E along with the synthesis of callipeltin B and M.12d,e While both research teams could synthesise the simplified representative callipeltin B, containing a dimethyl pyroglutamic acid instead of the complex side chain, no successful synthesis of the parent structure of callipeltin A has been reported to date.
To enable the synthesis of callipeltin A and derivatives thereof for structure–activity relationship (SAR) studies, the development of a flexible and robust synthetic route is highly desirable.
Results and discussion
Herein, we report the straightforward access of the cyclic heptapeptide core of callipeltin A, the parent structure of this class of natural products.
To begin our synthesis of the peptide core, we prepared the nonproteinogenic amino acid building blocks, β-methoxy tyrosine and D-allo-threonine. The synthesis of the tyrosine moiety was based on an asymmetric Sharpless dihydroxylation, which provided diol 2 in a highly enantio-selective fashion (Scheme 1).13 We achieved the selective nosylation of the α-hydroxy group with nosyl-chloride treatment. Furthermore, the immediate substitution with sodium azide afforded azido ester 3 in good yield. The second hydroxy group was converted into the corresponding methyl ether using a protocol of Cuevas with methyl iodide in the presence of silver oxide.13 Azide 4 was reduced by the Staudinger reaction, and the resulting amine was Boc-protected (5). Finally, the saponification of the methyl ester provided protected tyrosine 6 in almost quantitative yield.
 |
| Scheme 1 Preparation of protected tyrosine 6. | |
The synthesis of the protected D-allo-threonine building block was attempted via three successive Matteson homologations14 from known methyl boronic ester 7.15 The first homologation introduced the benzyl-protected hydroxy functionality by treatment of the intermediary α-chloro boronic ester with sodium benzylate in the presence of zinc chloride (Scheme 2). A second homologation followed by substitution with sodium azide afforded α-azido boronic ester 9 as a single stereoisomer. The ensuing homologation of boronic ester 9 to the corresponding carboxylic acid under typical conditions initially suffered from low conversion rates and the formation of several side products. These issues could be avoided when the reaction was carried out with dibromomethane instead of dichloromethane to yield the corresponding α-bromo boronic ester.16 The oxidation of the intermediary α-bromo boronic ester under Pinnick-type conditions followed by treatment with TMS-diazomethane afforded azido ester 10. In addition, azide reduction was achieved via Staudinger reaction, and the resulting amine was protected as its Alloc carbamate 11. The saponification of the methyl ester with lithium hydroxide gave rise to protected D-allo-threonine 12 in a quantitative fashion.
 |
| Scheme 2 Matteson homologation toward D-allo-threonine 12. | |
The peptide chain assembly started with tyrosine 6, which was coupled with N-Me-Ala-OH (Scheme 3). Best results were obtained with the uronium coupling reagent HBTU, which minimised the elimination of methanol from the β-methoxy tyrosine upon activation, thus affording dipeptide 13 in acceptable yield. We prepared the required N-methyl glutamine for the ensuing coupling step from commercially available Fmoc-Gln(Trt)-OH by hemiaminal formation and ionic hydrogenation to install the N-methyl group (see ESI† for more details).17
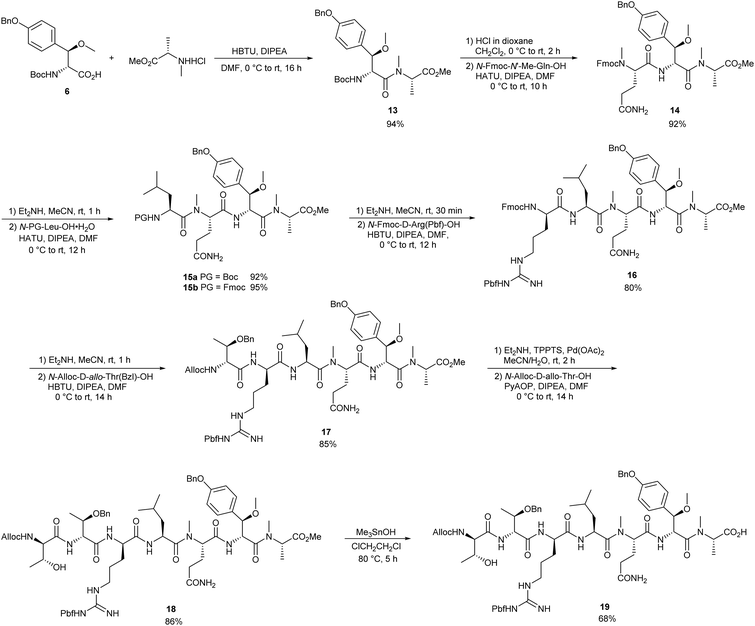 |
| Scheme 3 Synthesis of linear peptide 19. | |
The N-terminal deprotection of dipeptide 13 with hydrochloric acid and subsequent coupling of the resulting hydrochloride with N-Fmoc-N′-Me-Gln-OH in the presence of HATU and DIPEA gave rise to tripeptide 14. After the treatment of 14 with excess diethylamine to achieve Fmoc deprotection, the free amine was reacted with Boc- and Fmoc-Leu-OH by activation with HATU to obtain tetrapeptide 15a and 15b, respectively. However, the removal of the Boc group was impossible under various conditions because of the decomposition of the peptide under Lewis and Brønsted acidic conditions. In contrast, the treatment of Fmoc-tetrapeptide 15b with excess diethylamine led to the clean deprotection of the N-terminus. Furthermore, subsequent coupling with commercially available N-Fmoc-D-Arg(Pbf)-OH in the presence of HBTU gave pentapeptide 16 in good yield.
After N-terminal Fmoc deprotection was carried out under identical conditions once more, the resulting amine was reacted with D-allo-threonine 17via HBTU activation. The final Alloc-deprotection was achieved by palladium catalysed allylic alkylation with water-soluble phosphine ligand TPPTS (3,3′,3′′-phosphanetriyltris-(benzenesulfonic acid) trisodium salt).18 The ensuing coupling initially suffered from low conversion when typical coupling reagents such as HBTU, EDC, HATU or COMU were used. However, PyAOP,19 the nitrogen analogue of PyBOP known to be an exceptional reagent for coupling and cyclisation of sensitive substrates, worked excellently. As a result, heptapeptide 18 could be obtained in 86% yield.
While methyl ester 18 underwent saponification rapidly, a loss in the molecular mass of 58 was detected, corresponding to the loss of an allyl alcohol fragment. NMR analysis verified the assumption of N-terminal oxazolidinone formation via base-induced intramolecular cyclisation. Attempts to suppress the oxazolidinone formation using different metal hydroxides or performing the reaction at a lower temperature gave similar results. Fortunately, the use of the mild and significantly less basic saponification reagent Me3SnOH, initially described by Nicolaou et al.,20 selectively afforded the desired carboxylic acid 19 in good yield.
With the hydroxy acid 19 in hand, we then examined the macrocyclisation via a protocol described by Konno et al., who achieved cyclisation of a similar linear peptide in 44% yield. They used an excess of DIC and DMAP at 45 °C during their synthesis of callipeltin B.12e In the case of linear heptapeptide 19, macrolactone 20 was obtained in only 32% along with 64% of N-acylurea resulting from acyl migration of the intermediary O-acylisourea (Table 1, entry 1). While such yields are common for the cyclisation of complex peptides and depsipeptides, we made additional attempts to increase the yield.
Table 1 Attempts of macrolactonisation
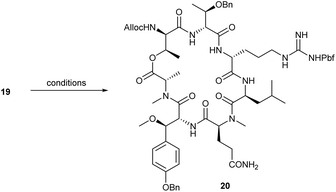
|
Entry |
Conditions |
Solvent |
c [mM] |
Y [%] |
1 |
DIC (5 eq.), DMAP (20 eq.), 45 °C, 72 h |
DMF |
7.5 |
32% |
2 |
Yamaguchi reagent (1.5 eq.), DMAP (1–20 eq.), NEt3 (5.0 eq.) 20 °C, 24 h |
Toluene |
1.0 |
— |
3 |
DIC (5 eq.), DMAP (20 eq.), 70 °C, 72 h |
DMF |
7.5 |
39% |
4 |
PyAOP (1.2 eq.), DMAP (20 eq.), 45 °C, 72 h |
DMF |
7.5 |
59% |
5 |
PyAOP (1.2 eq.), DMAP (20 eq.), 70 °C, 72 h |
DMF |
7.5 |
83% |
First, the reaction was carried out under Yamaguchi conditions.21 However, all experiments using this protocol showed no product formation (entry 2); thus, the initial reaction conditions were re-examined. The reaction was carried out with excesses of DIC and DMAP at various temperatures and concentrations, but the yield could only be improved slightly at 70 °C (entry 3).
In subsequent attempts, the use of PyAOP, known to be an excellent reagent for macrolactamisation, providing exceptional results in earlier peptide couplings, was studied (entries 4 and 5). As expected, without the addition of DMAP, the use of PyAOP at room temperature resulted in no conversion, owing to the lower nucleophilicity of the alcohol compared to amine couplings. When an excess of DMAP was added, however, and the reaction was conducted at elevated temperatures, macrolactone 20 was obtained in 59% yield. Increasing the temperature to 70 °C resulted in the formation of macrolactone in exceptional yield, surprisingly, without significant erosion of the adjacent stereocentre of the activated N-methyl alanine. Trace amounts of the epimer (1–2%) were readily removed during purification via preparative HPLC. The obtained macrolactone 20 represents a protected derivative of the peptide core of callipeltin A. After the cleavage of the N-terminal Alloc protection group, this cyclic peptide should allow for coupling with the sidechain to access the natural product quickly.
Conclusions
In conclusion, we developed a straightforward approach to obtaining the protected cyclic depsipeptide core of callipeltin A. This route includes the preparation of the nonproteinogenic amino acid building blocks in a highly stereoselective fashion and the subsequent assembly of the peptide chain via successive deprotection and peptide coupling steps. The homologation of chiral boronic esters using Matteson's protocol was an effective tool for preparing protected D-allo-threonine 12. Further application of this method in the synthesis of amino acids is ongoing. Furthermore, we achieved the cyclisation of linear peptide 19 with PyAOP in excellent yield without significant erosion of the adjacent stereocentre of the activated amino acid. After Alloc deprotection, the resulting amine should readily be coupled with the side chain of callipeltin A to afford the natural product in a few additional steps. The synthesis of the sidechain and the coupling of both parts of callipeltin A are underway and will be reported in due course.
Conflicts of interest
There are no conflicts to declare.
Acknowledgements
Financial support by the Saarland University and the DFG (Ka 880/13-1 and Bruker Neo 500 - 447298507) is gratefully acknowledged.
References
- A. Zampella, M. V. D'Auria, L. Gomez Paloma, A. Casapullo, L. Minale, C. Debitus and Y. Henin, Callipeltin A, an Anti-HIV Cyclic Depsipeptide from the New Caledonian Lithistida Sponge Callipelta, sp., J. Am. Chem. Soc., 1996, 118, 6202–6209 CrossRef CAS.
-
(a) A. Zampella, A. Randazzo, N. Borbone, S. Luciani, L. Trevisi, C. Debitus and M. V. D'Auria, Isolation of callipeltins A–C and of two new open-chain derivatives of callipeltin A from the marine sponge Latrunculia sp. A revision of the stereostructure of callipeltins, Tetrahedron Lett., 2002, 43, 6163–6166 CrossRef CAS;
(b) A. Zampella, R. D'Orsi, V. Sepe, A. Casapullo, M. C. Monti and M. V. D'Auria, Concise Synthesis of All Stereoisomers of β-Methoxytyrosine and Determination of the Absolute Configuration of the Residue in Callipeltin A, Org. Lett., 2005, 7, 3585–3588 CrossRef CAS PubMed;
(c) C. Bassarello, A. Zampella, M. C. Monti, L. Gomez-Paloma, M. V. D'Auria, R. Riccio and G. Bifulco, Quantum Mechanical Calculation of Coupling Constants in the Configurational Analysis of Flexible Systems: Determination of the Configuration of Callipeltin A, Eur. J. Org. Chem., 2006, 604–609 CrossRef CAS.
-
(a) M. V. D'Auria, A. Zampella, L. G. Paloma, L. Minale, C. Debitus, C. Roussakis and V. Le Bert, Callipeltins B and C; Bioactive Peptides from a Marine Lithistida Sponge Callipelta sp., Tetrahedron, 1996, 52, 9589–9596 CrossRef;
(b) V. Sepe, R. D'Orsi, N. Borbone, M. V. D'Auria, G. Bifulco, M. C. Monti, A. Catania and A. Zampella, Callipeltins F–I: new antifungal peptides from the marine sponge Latrunculia sp., Tetrahedron, 2006, 62, 833–840 CrossRef CAS;
(c) M. V. D'Auria, V. Sepe, R. D'Orsi, F. Bellotta, C. Debitus and A. Zampella, Isolation and structural elucidation of callipeltins J–M: antifungal peptides from the marine sponge Latrunculia sp., Tetrahedron, 2007, 63, 131–140 CrossRef;
(d) M. Stierhof, K. Ø. Hansen, M. Sharma, K. Feussner, K. Subko, F. F. Díaz-Rullo, J. Isaksson, I. Pérez-Victoria, D. Clarke, E. Hansen, M. Jaspars and J. N. Tabudravu, New cytotoxic callipeltins from the Solomon Island marine sponge Asteropus sp., Tetrahedron, 2016, 72, 6929–6934 CrossRef CAS.
-
(a) A. Plaza, E. Gustchina, H. L. Baker, M. Kelly and C. A. Bewley, Mirabamides A-D, Depsipeptides from the Sponge Siliquariaspongia mirabilis That Inhibit HIV-1 Fusion, J. Nat. Prod., 2007, 70, 1753–1760 CrossRef CAS PubMed;
(b) Z. Lu, R. M. Van Wagoner, M. K. Harper, H. L. Baker, J. N. A. Hooper, C. A. Bewley and C. M. Ireland, Mirabamides E-H, HIV-Inhibitory Depsipeptides from the Sponge Stelletta Clavosa, J. Nat. Prod., 2011, 74, 185–193 CrossRef CAS PubMed.
-
(a) P. W. Ford, K. R. Gustafson, T. C. McKee, N. Shigematsu, L. K. Maurizi, L. K. Pannell, D. E. Williams, E. Dilip de Silva, P. Lassota, T. M. Allen, R. Van Soest, R. J. Andersen and M. R. Boyd, Papuamides A-D, HIV-Inhibitory and Cytotoxic Depsipeptides from the Sponges Theonella mirabilis and Theonella swinhoei Collected in Papua New Guinea, J. Am. Chem. Soc., 1999, 121, 5899–5909 CrossRef CAS;
(b) P. Prasad, W. Aalbersberg, K.-D. Feussner and R. M. Van Wagoner, Papuamides E and F, Cytotoxic Depsipeptides from the Marine Sponge Melophlus sp., Tetrahedron, 2011, 67, 8529–8531 CrossRef CAS PubMed.
-
(a) N. Oku, K. R. Gustafson, L. K. Cartner, J. A. Wilson, N. Shigematsu, S. Hess, L. K. Pannell, M. R. Boyd and J. B. McMahon, Neamphamide A, a New HIV-Inhibitory Depsipeptide from the Papua New Guinea Marine Sponge Neamphius huxleyi., J. Nat. Prod., 2004, 67, 1407–1411 CrossRef CAS PubMed;
(b) Y. Yamano, M. Arai and M. Kobayashi, Neamphamide B, new cyclic depsipeptide, as an anti-dormant mycobacterial substance from a Japanese marine sponge of Neamphius sp., Bioorg. Med. Chem. Lett., 2012, 22, 4877–4881 CrossRef CAS PubMed;
(c) T. D. Tran, N. B. Pham, G. Fechner, D. Zencak, H. T. Vu, J. N. A. Hooper and R. J. Quinn, Cytotoxic Cyclic Depsipeptides from the Australian Marine Sponge Neamphius huxleyi., J. Nat. Prod., 2012, 75, 2200–2208 CrossRef CAS PubMed.
-
(a) L. Trevisi, S. Bova, G. Cargnelli, D. Danieli-Betto, M. Floreani, E. Germinario, M. V. D'Auria and S. Luciani, Callipeltin A, a Cyclic Depsipeptide Inhibitor of the Cardiac Sodium–Calcium Exchanger and Positive Inotropic Agent, Biochem. Biophys. Res. Commun., 2000, 279, 219–222 CrossRef CAS PubMed;
(b) L. Trevisi, G. Cargnelli, G. Ceolotto, I. Papparella, A. Semplicini, A. Zampella, M. V. D'Auria and S. Luciani, Callipeltin A: sodium ionophore effect and tension development in vascular smooth muscle, Biochem. Pharmacol., 2004, 68, 1331–1338 CrossRef CAS PubMed;
(c) M. Kikuchi, Y. Watanabe, M. Tanaka, K. Akaji and H. Konno, Synthesis and cytotoxicity of the depsipeptides analogues of callipeltin B, Bioorg. Med. Chem. Lett., 2011, 21, 4865–4868 CrossRef CAS PubMed.
-
(a) N. Okamoto, O. Hara, K. Makino and Y. Hamada, Diastereoselective Synthesis of All Stereoisomers of β-Methoxytyrosine, a Component of Papuamides, J. Org. Chem., 2002, 67, 9210–9215 CrossRef CAS PubMed;
(b) D. B. Hansen, X. Wan, P. J. Carroll and M. M. Joullié, Stereoselective Synthesis of Four Stereoisomers of β-Methoxytyrosine, a Component of Callipeltin A, J. Org. Chem., 2005, 70, 3120–3126 CrossRef CAS PubMed;
(c) H. Konno, S. Aoyama, K. Nosaka and K. Akaji, Stereoselective Synthesis of β-Methoxytyrosine Derivatives for Identification of the Absolute Configuration of Callipeltin E, Synthesis, 2007, 3666–3672 CrossRef CAS;
(d) D. C. Cranfill and M. A. Lipton, Enantio- and Diastereoselective Snythesis of (R,R)-β-Methoxytyrosine, Org. Lett., 2007, 9, 3511–3513 CrossRef CAS PubMed.
-
(a) B. Liang, P. J. Carroll and M. M. Joullié, Progress toward the Total Synthesis of Callipeltin A(I): Asymmetric Synthesis of (3S,4R,)-3,4-Dimethylglutamine, Org. Lett., 2000, 2, 4157–4160 CrossRef CAS PubMed;
(b) C. M. Acevedo, E. F. Kogut and M. A. Lipton, Synthesis and Analysis of the Sterically Constrained L-Glutamine Analogues (3S,4R,)-3,4-Dimethyl-L-glutamine and (3S,4R)-3,4-Dimethyl-L-pyroglutamic Acid, Tetrahedron, 2001, 57, 6353–6359 CrossRef CAS;
(c) N. Okamoto, O. Hara, K. Makino and Y. Hamada, Stereoselective Synthesis of (3S,4R,)-3,4-Dimethyl-(S)-glutamine and the Absolute Stereochemistry of the Natural Product from Papuamides and Callipeltin, Tetrahedron: Asymmetry, 2001, 12, 1353–1358 CrossRef CAS;
(d) W. Xie, D. Ding, W. Zi, G. Li and D. Ma, Total Synthesis and Structure Assignment of Papuamide B, A Potent Marine Cyclodepsipeptide with Anti-HIV Properties, Angew. Chem., Int. Ed., 2008, 47, 2844–2848 CrossRef CAS PubMed.
-
(a) S. Chandrasekhar, T. Ramachandar and B. V. Rao, Chiron Approach to Callipeltin A: First Synthesis of Fully Protected (2R,3R,4S,)-4,7-Diamino-2,3-dihydroxy Heptanoic Acid, Tetrahedron: Asymmetry, 2001, 12, 2315–2321 CrossRef CAS;
(b) A. Ravi Kumar and B. Venkateswara Rao, An Efficient Synthesis of Protected (2R,3R,4S,)-4,7-Diamino-2,3-dihydroxyheptanoic Acid, a Constituent of Callipeltins A and D, Tetrahedron Lett., 2003, 44, 5645–5647 CrossRef CAS;
(c) J. C. Thoen, Á. I. Morales-Ramos and M. A. Lipton, A. Synthesis of the Unnatural Amino Acid AGDHE, a Constituent of the Cyclic Depsipeptides Callipeltins A and D, Org. Lett., 2002, 4, 4455–4458 CrossRef CAS PubMed;
(d) S. Chandrasekhar, M. S. Reddy, G. S. Kiranbabu and A. S. K. Murthy, Synthesis of Protected (2R,3R,4S,)-4,7-Diamino-2,3-dihydroxyheptanoic Acid, a Constituent of Callipeltins A and D, Tetrahedron Lett., 2006, 47, 7307–7309 CrossRef CAS;
(e) J. Jeon, S. K. Hong, J. S. Oh and Y. G. Kim,
J., Stereoselective Synthesis of Protected (2R,3R,4S,)-4,7-Diamino-2,3-dihydroxyheptanoic Acid: A Novel Amino Acid of Callipeltins A and D, Org. Chem., 2006, 71, 3310–3313 CrossRef CAS PubMed;
(f) R. Yoshino, Y. Tokairin and H. Konno, Synthesis of fully protected (2R,3R,4S,)-4-Amino-7-guanidino-2,3-dihydroxy Heptanoic Acid, Tetrahedron Lett., 2017, 58, 1604–1606 CrossRef CAS.
-
(a) A. Zampella and M. V. D'Auria, Stereoselective Synthesis of (2R,3R,4R,)-3-Hydroxy-2,4,6-trimethylheptanoic Acid and Determination of the Absolute Stereochemistry of the Natural Product from Callipeltin A, Tetrahedron: Asymmetry, 2002, 13, 1237–1239 CrossRef CAS;
(b) J. A. Turk, G. S. Visbal and M. A. Lipton, Asymmetric Synthesis of Four Diastereomers of 3-Hydroxy-2,4,6-trimethyl-heptanoic Acid: Proof of Configurational Assignment, J. Org. Chem., 2003, 68, 7841–7844 CrossRef CAS PubMed;
(c) K. A. Parker and Q. Xie, Asymmetric Catalysis Route to anti,anti Stereotriads, Illustrated by Applications, Org. Lett., 2008, 10, 1349–1352 CrossRef CAS PubMed;
(d) G. Sabitha, K. Yadagiri, G. Chandrashekhar and J. S. Yadav, Synthetic Studies on Callipeltin A: Stereoselective Synthesis of (2R,3R,4S,)-3- Hydroxy-2,4,6-trimethylheptanoic Acid, Synthesis, 2010, 4307–4311 CrossRef CAS;
(e) Y. Tokairin and H. Konno, Preparation of (2R,3R,4R,)-3-Hydroxy-2,4,6-trimethylheptanoic Acid via Enzymatic Desymmertization, Tetrahedron, 2017, 73, 39–45 CrossRef CAS.
-
(a) D. C. Cranfill, Á. I. Morales-Ramos and M. A. Lipton, Solid-Phase Synthesis of Callipeltin D. Stereochemical Confirmation of the Unnatural Amino Acid AGDHE, Org. Lett., 2005, 7, 5881–5883 CrossRef CAS PubMed;
(b) S. Çalimsiz, Á. I. M. Ramos and M. A. Lipton, Solid-Phase Synthesis and Configurational Reassigment of Callipeltin E. Implications for the Structures of Callipeltins A and B, J. Org. Chem., 2006, 71, 6351–6356 CrossRef PubMed;
(c) R. Krishnamoorthy, L. D. Vazquez-Serrano, J. A. Turk, J. A. Kowalski, A. G. Benson, N. T. Breaux and M. A. Lipton, Solid-Phase Total Synthesis and Structure Proof of Callipeltin B, J. Am. Chem. Soc., 2006, 128, 15392–15393 CrossRef CAS PubMed;
(d) M. Kikuchi, K. Nosaka, K. Akaji and H. Konno, Solid phase total synthesis of callipeltin E isolated from marine sponge Latrunculia sp., Tetrahedron Lett., 2011, 52, 3872–3875 CrossRef CAS;
(e) M. Kikuchi and H. Konno, Total Synthesis of Callipeltin B and M, Peptidyl Marine Natural Products, Org. Lett., 2014, 16, 4324–4327 CrossRef CAS PubMed.
- M. J. Martin, R. Rodriguez-Acebes, Y. Garcia-Ramos, V. Martinez, C. Murcia, I. Digon, I. Marco, M. Pelay-Gimeno, R. Fernández, F. Reyes, A. M. Francesch, S. Munt, J. Tulla-Puche, F. Albericio and C. Cuevas, Stellatolides, a New Cyclodepsipeptide Family from the Sponge Ecionemia acervus: Isolation, Solid-Phase Total Synthesis, and Full Structural Assignment of Stellatolide A, J. Am. Chem. Soc., 2014, 136, 6754–6762 CrossRef CAS PubMed.
- Reviews:
(a) D. S. Matteson, Boronic Esters in Stereodirected Synthesis, Tetrahedron, 1989, 45, 1859–1885 CrossRef CAS;
(b) D. S. Matteson, Boronic Esters in Asymmetric Synthesis, J. Org. Chem., 2013, 78, 10009–10023 CrossRef CAS PubMed;
(c) S. Kirupakaran, H.-G. Korth and C. Hirschhäuser, A Complementary Toolbox of Iterative Methods for Stereoselective Synthesis, Synthesis, 2018, 50, 2307–2322 CrossRef CAS;
(d) D. S. Matteson, B. S. L. Collins, V. K. Aggarwal and E. Ciganik, The Matteson Reaction, Org. React., 2021, 105, 427–860 Search PubMed.
- J. Gorges and U. Kazmaier, Matteson Homologation-based
Total Synthesis of Lagunamide A, Org. Lett., 2018, 20, 2033–2036 CrossRef CAS PubMed.
- T. J. Michnick and D. S. Matteson, (Bromomethyl)lithium: Efficient in situ Reactions, Synlett, 1991, 631–632 CrossRef CAS.
- T. Sun, W. Zhang, C. Zong, P. Wang and Y. Li, Total Synthesis and Stereochemical Reassignment of Tasiamide B, J. Pept. Sci., 2010, 16, 364–374 CAS.
-
(a) S. Lemaire-Audoire, M. Savignac, E. Blart, G. Pourcelot, J. P. Genêt and J.-M. Bernard, Selective Deprotective Method Using Palladium - Water soluble Catalysts, Tetrahedron Lett., 1994, 35, 8783–8786 CrossRef CAS;
(b) S. Lemaire-Audoire, M. Savignac, E. Blart, J.-M. Bernard and J. P. Genêt, Synthesis of Peptides Using Palladium Promoted Selective Removal of Allyloxy-carbonyl Protecting Groups in Aqueous Medium, Tetrahedron Lett., 1997, 38, 2955–2958 CrossRef CAS.
- F. Albericio, M. Cases, J. Alsina, S. A. Triolo, L. A. Carpino and S. A. Kates, On the Use of PyAOP, a Phosphonium Salt Derived from HOAt, in Solid-Phase Peptide Synthesis, Tetrahedron Lett., 1997, 38, 4853–4856 CrossRef CAS.
- K. C. Nicolaou, A. A. Estrada, M. Zak, S. H. Lee and B. S. Safina, A Mild and Selective Method for the Hydrolysis of Esters with Trimethyltin Hydroxide, Angew. Chem., Int. Ed., 2005, 44, 1378–1382 CrossRef CAS PubMed.
-
(a) J. Inanaga, K. Hirata, H. Saeki, T. Katsuki and M. Yamaguchi, A Rapid Esterification by Means of Mixed Anhydride and Its Application to Large-ring Lactonization, Bull. Chem. Soc. Jpn., 1979, 52, 1989–1993 CrossRef CAS;
(b) J. R. Cochrane, D. H. Yoon, C. S. P. McErlean and K. A. Jolliffe, A Macrolactonization Approach to the Total Synthesis of Antimicrobial Cyclic Depsipeptide LI-FO4a and Diastereoisomeric Analogues, Beilstein J. Org. Chem., 2012, 8, 1344–1351 CrossRef CAS PubMed;
(c) M. Hikota, H. Tone, K. Horita and O. Yonemitsu, Chiral Synthesis of Polyketide-Derived Natural Products. 27. Stereoselective Synthesis of Erythronolide A via an Extremly Efficient Macrolactonization by the Modified Yamaguchi Method, J. Org. Chem., 1990, 55, 7–9 CrossRef CAS;
(d) W. R. Roush, K. Koyama, M. L. Curtin and K. J. Moriarty, Studies on the Synthesis of Nargenicin A1: Highly Stereoselective Synthesis of the Complete Carbon Framework via the Transannular Diels-Alder Reaction of an 18-Membered Macrolide, J. Am. Chem. Soc., 1996, 118, 7502–7512 CrossRef CAS;
(e) M. Hikota, Y. Sakurai, K. Horita and O. Yonemitsu, Synthesis of Erythronolide A via a Very Efficient macrolactonization under Usual Acylation Conditions with the Yamaguchi Reagent, Tetrahedron Lett., 1990, 31, 6367–6370 CrossRef CAS.
Footnote |
† Electronic supplementary information (ESI) available: Experimental details, compound characterization, copies of 1H and 13C NMR spectra. See DOI: https://doi.org/10.1039/d2qo01120d |
|
This journal is © the Partner Organisations 2022 |
Click here to see how this site uses Cookies. View our privacy policy here.