DOI:
10.1039/D1QO01603B
(Research Article)
Org. Chem. Front., 2022,
9, 75-80
Alkyl-modified nucleobases with 6/5/7/5 ring systems from the insect Cyclopelta parva†
Received
25th October 2021
, Accepted 4th November 2021
First published on 5th November 2021
Abstract
(±)-Cyclopeltains A and B (1 and 2), two pairs of unusual alkylated adenine derivatives, were isolated from the insect Cyclopelta parva and structurally characterized. 1 and 2 both possess a 6/5/7/5 ring system arising from the alkylation of N7 and N10 by a C6 unit and the formation of a furan ring. A plausible biosynthetic pathway for 1 and 2 was proposed. Biological evaluation of the enantiomers of 1 and 2 revealed that (−)-1 and (+)-2 could inhibit the production of TNF-α and IL-6 and the expression of iNOS, COX-2, and p-NF-κB.
Introduction
Nonpeptidal small molecules in insects might play multiple roles in their life processes. For example, cantharidin, formonitrile, benzoquinone, and histamine in insects are well-known chemical weapons for defensive purposes. A recent study revealed that phenylacetonitrile could act as an olfactory aposematic signal and a precursor of hypertoxic hydrogen cyanide to protect gregarious locusts from predation of natural enemies, representing a clever chemical defense strategy in the animal kingdom.1 More examples are that structurally diverse insect pheromones are used as powerful tools for chemical communication. More recently, 4-vinylanisole as an aggregation pheromone in locusts has been identified, unveiling the agricultural devastation caused by Locusta migratoria.2 Beyond the above two aspects, small molecules such as ecdysteroids as important hormones in insects control the growth, development, ecdysteroidogenesis, and metabolism of insects. On our continuous efforts towards the isolation of nonpeptidal small molecules from insects, dozens of structurally intriguing compounds were characterized by us and these compounds exhibited potent biological activities.3–5 During our further efforts on insects, Cyclopelta parva was investigated. This insect can be seen in most places of China, and previous studies have revealed its anti-bacterial, anticancer, and antifatigue properties.6–8 Our previous study on C. parva led to the isolation of xanthine and dihydroxydopamine conjugates.9,10 Obviously, these compounds are modified nucleobases. As is known DNA methylation is an epigenetic mechanism which refers to the C5 position of the cytosine being methylated to form 5-methylcytosine. DNA methylation plays an important role in the regulation of gene expression. Traditionally, four nucleobases, adenine (A), cytosine (C), guanine (G), and thymine (T), are thought to be invariable in DNA. However, a new nucleobase diaminopurine was discovered in 1977,11 and its widespread occurrence and biosynthesis in phages were unveiled in 2021,12 representing a milestone in DNA study. Since we have identified modified derivatives of xanthine and dihydroxydopamine from the title insect, whether the other analogues are present in this insect and exploration of their biological roles is therefore necessary. A continuing study on C. parva led to the identification of (±)-cyclopeltains A and B (1 and 2) (Fig. 1), which are both novel C6 alkyl chain modified adenine derivatives. In this paper, we report their isolation, structure identification, and biological activities toward inflammation.
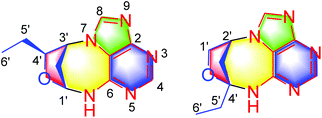 |
| Fig. 1 Chemical structures of compounds 1 and 2. | |
Results and discussion
(±)-Cyclopeltain A (1) was isolated as a yellowish solid, and its molecular formula was found to be C11H13N5O by the analysis of its HRESIMS, 13C NMR, and DEPT spectra, indicating 8 degrees of unsaturation. The 1H NMR spectrum of 1 (Table 1) shows two downfield proton signals (δH 8.52, s, H-4; δH 8.62, s, H-8) and one broad singlet at δH 9.41. The 13C NMR and DEPT spectra (Table 1) display eleven carbons classified into one methyl, two methylenes, five methines (two sp2, two oxygenated sp3), and three nonprotonated carbons (all sp2). The architecture of 1 was determined by 2D NMR experiments (Fig. 2). The 1H–1H COSY spectrum gives correlations of H-6′/H-5′, H-5′/H-4′, H-4′/H-3′, H-3′/H-2′, and H-2′/H-1′, indicating the presence of C-1′–C-6′. The HMBC correlations of H-1′/C-4′, in consideration of the chemical shifts of C-1′ (δC 83.3) and C-4′ (δC 86.4), suggest the formation of ring D. Apart for the signals accounting for C-1′–C-6′, the remaining 13C NMR signals along with the diagnostic 1H NMR for H-4, H-8, and NH imply the presence of an adenine residue. In order to understand the connection pattern between C-1′–C-6′ and the adenine part, the HMBC spectrum was further analyzed. The observed HMBC correlations of H-3′/C-1, C-8, H-1′/C-6, NH/C-1′, C-2′ suggest the formation of ring C, a seven-membered ring. Thus, the planar structure of 1 was deduced (Fig. 1). The rings C and D fused via C-1′–C3′ make 1 an endocyclic structure.
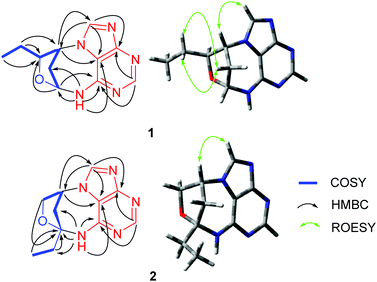 |
| Fig. 2 Important 2D NMR correlations of 1 and 2. | |
Table 1
1H (600 MHz) and 13C NMR (150 MHz) data of 1 and 2 in DMSO-d6 (δ in ppm, J in Hz)
No. |
1
|
2
|
δ
H
|
δ
C
|
δ
H
|
δ
C
|
1 |
|
110.8 s |
|
110.8 s |
2 |
|
156.8 s |
|
150.2 s |
4 |
8.52 (s) |
150.0 s |
8.33 (s) |
152.1 d |
6 |
|
150.7 s |
|
159.4 s |
8 |
8.62 (s) |
144.8 d |
8.41 (s) |
144.1 d |
1′ |
5.58 (dd, 7.8, 5.3) |
83.3 d |
Ha: 3.89 (dd, 9.3, 3.3) |
73.1 t |
Hb: 3.76 (dd, 9.3, 1.8) |
2′ |
Ha: 2.95 (ddd, 14.4, 7.8, 5.8) |
37.0 t |
5.34 (dd, 5.5, 3.3) |
57.6 d |
|
Hb: 2.33 (d, 14.4) |
3′ |
5.22 (d, 5.8) |
59.9 d |
Ha: 2.67 (dd, 14.1, 5.5) |
42.0 t |
Hb: 2.29 (dd, 14.1, 1.8) |
4′ |
3.85 (dd, 7.9, 6.0) |
86.4 d |
|
94.5 s |
5′ |
1.48 (m) |
26.9 t |
Ha: 2.07 (m) |
32.3 t |
Hb: 1.87 (m) |
6′ |
0.92 (t, 7.4) |
9.8 q |
1.00 (t, 7.4) |
8.7 q |
6-NH |
9.41 (brs) |
|
8.46 (brs) |
|
There are three chiral carbons in compound 1. The key ROESY correlations of H-5′/Ha-2′, Hb-2′, in consideration of the endocyclic nature of 1, clearly indicate the relative configurations at C-1′, C-3′, and C-4′. So far, many insect-derived small molecules have been isolated as racemates. In this context, the racemic nature of 1 was routinely examined by chiral HPLC. It was found that 1 is a racemate. Subsequent chiral HPLC separation afforded enantiomers (+)-1 and (−)-1. To clarify the absolute configurations of 1's antipodes, electronic circular dichroism (ECD) calculations were carried out. The results show that the calculated ECD spectrum of (1′R,3′R,4′S)-1 matches well with the experimental spectrum of (−)-1 (Fig. 3), indicating (1′R,3′R,4′S)-1 for (−)-1 and (1′S,3′S,4′R)-1 for (+)-1.
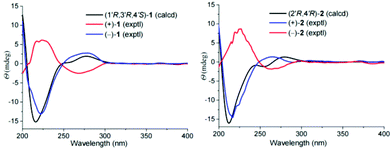 |
| Fig. 3 Calculated and experimental ECD spectra of 1 and 2. | |
(±)-Cyclopeltain B (2) was isolated as a yellowish solid. Its molecular formula C11H13N5O was deduced on the basis of the HRESIMS, 13C NMR, and DEPT spectra, having 8 degrees of unsaturation. The 13C NMR and DEPT spectra (Table 1 and S2†) give eleven carbons ascribed to one methyl, three sp3 methylenes (one oxygenated), three methines (two sp2 and one sp3), and four nonprotonated carbons (three sp2 and one sp3). These characteristics in combination with the diagnostic proton signals at H-4 (δH 8.33, s) H-8 (δH 8.41, s), and NH (δH 8.46, brs) observed in the 1H NMR spectrum (Table 1) suggest that 2 is an analogue of 1, differing by a methine (C-4′) in 1 being replaced by a quaternary carbon (C-4′) in 2. This alteration is evidenced by the 1H–1H COSY correlations of H-1′/H-2′, H-2′/H-3′, and H-5′/H-6′ and HMBC correlations of H-2′, H-3′, H-5′, H-6′/C-4′ (δC 94.5). Thus, the planar structure of 2 was assigned (Fig. 1).
As for the relative configuration of 2, the endocyclic formation in 2 indicates the configurations at bridgehead carbon atoms. Similarly, the absolute configuration of 2 was assigned by ECD calculations. The results show that the calculated ECD spectrum of (2′R,4′R)-2 agrees well with that of (+)-2, suggesting (2′R,4′R)-2 for (+)-2 and (2′S,4′S)-2 for (−)-2.
For a long time, four nucleobases, A, C, G, and T, are thought to be invariable. Although a number of nucleobase analogues exist exemplified by several nucleoside analogues being used as anticancer and antiviral agents in medicine, naturally occurring new nucleobases are rare. In 1950, 5-methylcytosine in nucleic acids was discovered.13 Thereafter, 6-methylaminopurine in bacterial DNA was reported in 1968.14 Until the year 2015, N6-methyladenine was discovered respectively in Chlamydomonas, Caenorhabditis elegans, and Drosophila.15–17 These findings indicate that modification of nucleobases could occur in different patterns. Under this circumstance, our present identification of alkylated adenines might provide new insight into nucleobase modification. We therefore hypothesized a plausible pathway for the biogenesis of 1 and 2 (Scheme 1). In the previous studies, we found many heteromers of adenine and 2-hexenal derivatives from Aspongopus chinensis and C. parva.9,10,18 This may account for the ubiquity of adenine and hexenal derivatives in these two species. Firstly, 2-hexenal could give rise to compounds 3 and 4, which is followed by the Michael addition reactions of adenine to produce intermediates 1-A and 2-A, respectively. Further 1-A and 2-A could undergo electron migration and then nucleophilic addition reactions to form intermediates 1-B and 2-B, respectively. Finally, contraction of the tetrahydrofuran ring via nucleophilic addition forms compounds 1 and 2.
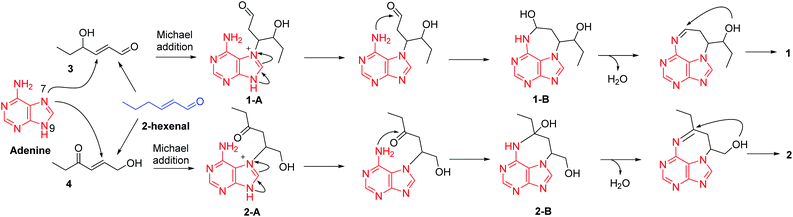 |
| Scheme 1 A plausible pathway for the biogenesis of 1. | |
In this study, the anti-inflammatory activity of the compounds was evaluated. In detail, we measured the secretion of proinflammatory cytokines including TNF-α and IL-6 pretreated with 1 and 2 followed by LPS stimulation. The ELISA assay shows that compounds (–)-1 and (+)-2 are able to inhibit the production of TNF-α and IL-6 at 20 μM induced by bacterial LPS in RAW264.7 cells (Fig. S27A and B†). Subsequently, we examined the drug toxicity of compounds (–)-1 and (+)-2 in RAW264.7 cells. The CCK-8 assay shows that compounds (–)-1 and (+)-2 at the indicated concentrations do not affect the viability of the RAW264.7 cells (Fig. S27C and D†), thus excluding the potential toxic effects of compounds (–)-1 and (+)-2. Furthermore, ELISA analysis shows that compound (–)-1 could dose-dependently inhibit LPS-induced TNFα (Fig. 4A) and IL-6 (Fig. 4B) production. We next evaluated the effect of compound (–)-1 on the activation of the transcription factor nuclear factor-κB (NF-κB) pathway. NF-κB plays a key role in the transcriptional regulation of inflammatory cytokines and the development of inflammation.
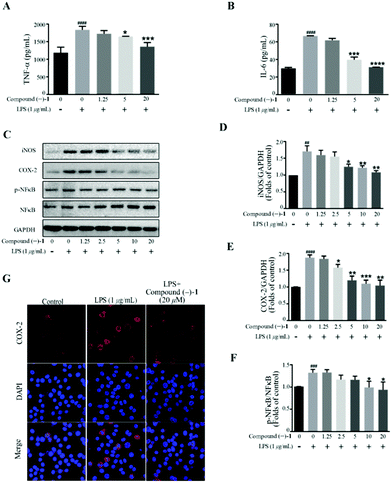 |
| Fig. 4 Compound (–)-1 suppresses proinflammatory production in LPS-induced RAW 264.7 cells. Cell were incubated in different concentrations of compound (–)-1 for 2 h and then exposed to 1 μg mL−1 LPS for 18 h. (A and B) The level of TNF-α (A) and IL-6 (B) in the supernatants was examined by ELISA. (C–F) The protein level of iNOS, COX-2, NF-κB and phosphor-NF-κB was determined by western blotting; GAPDH was used as a control. (G) The expression of COX-2 was analyzed by immunostaining with COX-2 (red) and DAPI (blue); the images were obtained by confocal microscopy. Data represent mean ± SD values of three experiments. *P < 0.05, **P < 0.01, ***P < 0.001 and ****P < 0.0001 compared with LPS alone. ###P < 0.001 and ####P < 0.0001 compared with DMSO alone. | |
Western blot analysis confirms that compound (–)-1 significantly inhibits LPS-induced activation of NF-κB in RAW264.7 cells (Fig. 4C and F). Similar results were also obtained from the expression level of inflammatory enzymes, such as iNOS and COX-2, which can be evaluated by activated NF-κB. As shown in Fig. 4C, LPS treatment markedly increases the protein levels of iNOS and COX-2 in RAW264.7 cells, and the effects are downregulated by compound (–)-1 (Fig. 4C–E). Moreover, the immunostaining results confirm the effect of compound (–)-1 regulating the expression of COX-2 (Fig. 4G). Hence, compound (–)-1 has a potential role in the amelioration of LPS induced inflammation in a concentration-dependent manner. Besides, as determined by the ELISA assay, pretreatment with compound (+)-2 also inhibits the LPS-induced production of secreted cytokines TNFα and IL-6 (Fig. 5A and B). Moreover, LPS-induced upregulated expression of phospho-NF-κB, iNOS, and COX-2 is blocked by compound (+)-2 at 20 μM (Fig. 5C–G). LPS treatment markedly enhanced the production of nitrite oxide. In a further study, we found that both compounds (–)-1 and (+)-2 significantly inhibited LPS-induced attenuation of the production of nitrite oxide (Fig. S29†).
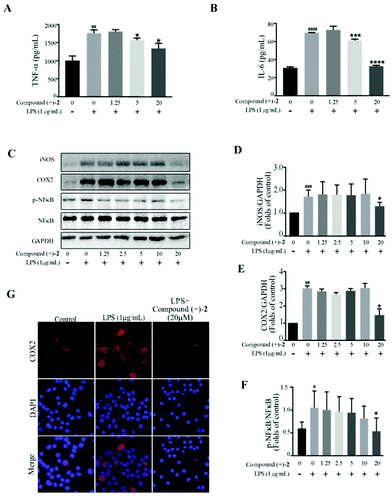 |
| Fig. 5 Compound (+)-2 suppresses proinflammatory production in LPS-induced RAW 264.7 cells. Cell were incubated in different concentrations of compound (+)-2 for 2 h and then exposed to 1 μg mL−1 LPS for 18 h. (A and B) The level of TNF-α (A) and IL-6 (B) in the supernatants was examined by ELISA. (C–F) The protein level of iNOS, COX-2, NF-κB and phosphor-NF-κB was determined by western blotting; GAPDH was used as a control. (G) The expression of COX-2 was analyzed by immunostaining with COX-2 (red) and DAPI (blue); the images were obtained by confocal microscopy. Data represent mean ± SD values of three experiments. *P < 0.05, **P < 0.01, ***P < 0.001 and ****P < 0.0001 compared with LPS alone. ##P < 0.01, ###P < 0.001 and ####P < 0.0001 compared with DMSO alone. | |
Conclusions
In summary, two pairs of unusual alkylated adenine derivatives were isolated from the insect C. parva. Compounds 1 and 2 both possess a 6/5/7/5 ring system arising from the alkylation of N7 and N10 by a C6 unit and the formation of a tetrahydrofuran ring. A plausible biosynthetic pathway for 1 and 2 was proposed. Biological evaluation of the enantiomers of 1 and 2 indicated that (−)-1 and (+)-2 could inhibit the production of TNF-α and IL-6 and the expression of iNOS, COX-2, and p-NF-κB. These findings suggest that the anti-inflammatory potential of these compounds would be worth further study.
Experimental section
General procedures
Column chromatography was performed using silica gel (200–300 mesh; Qingdao Marine Chemical Inc., P.R. China), macroporous adsorbent (Rohm Haas AMBERLITETM™ XAD™ 16N, USA), YMC gel ODS-A-HG (40–60 μm; YMC Co., Japan), MCI gel CHP 20P (75–150 μm, Mitsubishi Chemical Industries, Tokyo, Japan), and Sephadex LH-20 (Amersham Pharmacia, Sweden). Semi-preparative or analytic HPLC was carried out using an Agilent 1260 liquid chromatograph, and the column used was a 250 mm × 10 mm, i.d., 5 μm, YMC-Pack ODS-A and a 250 mm × 4.6 mm, i.d., 5 μm, Daicel Chiralpak (AD-H). NMR spectra were recorded on a Bruker AV-600 spectrometer, with TMS as an internal standard. HRESIMS were collected using a Shimadzu LC-20AD AB SCIEX triple TOF X500R MS spectrometer (Shimadzu Corporation, Tokyo, Japan). Optical rotations were measured on an Anton Paar MCP-100 digital polarimeter. UV spectra and CD spectra were obtained on a Jasco J-815 circular dichroism spectrometer (JASCO, Japan).
Insect material
The dry whole bodies of C. parva were purchased from Hunan Zhenxing Co. Ltd for Chinese Materia Medica, Changsha, P.R. China, in December 2017. The material was identified by Prof. De-Po Yang at School of Pharmaceutical Sciences, Sun Yat-Sen University, P.R. China. A voucher specimen (No. CHYX0628) is deposited at the School of Pharmaceutical Sciences, Shenzhen University, P.R. China.
Extraction and isolation
The air-dried powdered C. parva (45 kg) was extracted with 80% aqueous ethanol (3 × 270 L, 24 h each) at room temperature. The combined extracts were concentrated to obtain a crude extract, which was suspended in water and successively partitioned with petroleum ether and ethyl acetate. The water-soluble extract (4.0 kg) was divided into six portions (Fr.1–Fr.6) using a macroporous adsorbent AMBERLITE XAD 16N column eluted with gradient aqueous EtOH (10
:
90–100
:
0). Fr.2 (170 g) was separated using an MCI gel CHP 20P eluted with gradient aqueous MeOH (2
:
98–100
:
0) to afford nine fractions (Fr.2.1–Fr.2.9). Fr.2.4 (25.8 g) was subjected to an ODS column on an MPLC system eluted with gradient aqueous MeOH (2
:
98–100
:
0, flow rate: 20 mL min−1) to afford seven fractions (Fr.2.4.1–Fr.2.4.7). Fr.2.4.2 (2.07 g) was further fractionated into seven parts using Sephadex LH-20 (MeOH/H2O, 75
:
25), of which Fr.2.4.2.2 (567 mg) was subjected to an ODS column eluted with gradient aqueous MeOH (2
:
98–100
:
0) to provide four portions (Fr.2.4.2.2.1–Fr.2.4.2.2.4). Fr.2.4.2.2.2 (10.1 mg) was purified by semi-preparative HPLC (MeOH/H2O, 18
:
82, flow rate: 3 mL min−1) to give racemic compound 2 (Rt = 21.9 min, 2.19 mg). Racemic compound 2 was separated by semi-preparative HPLC on a chiral phase (AD-H, n-hexane/ethanol, 40
:
60, flow rate: 1 mL min−1) to afford compounds (–)-2 (Rt = 6.5 min, 0.88 mg) and ( + )-2 (Rt = 28.7 min, 0.80 mg). Fr.2.4.2.2.3 (140.3 mg) was further separated by preparative HPLC (MeOH/H2O, 5
:
95–100
:
0, flow rate: 10 mL min−1) to give six fractions (Fr.2.4.2.2.3.1–Fr.2.4.2.2.3.6). Fr.2.4.2.2.3.1 (8.5 mg) was purified by sequential semi-preparative HPLC (MeCN/H2O, 10
:
90, flow rate: 3 mL min−1) to give racemic compound 1 (Rt = 17.2 min, 2.84 mg). Racemic compound 1 was separated by semi-preparative HPLC on a chiral phase (AD-H, n-hexane/ethanol, 40
:
60, flow rate: 1 mL min−1) to afford compounds (–)-1 (Rt = 10.8 min, 1.1 mg) and ( + )-1 (Rt = 13.2 min, 1.2 mg).
(±)-Cyclopeltain A (1): a yellowish solid; UV (MeOH) λmax(log
ε) 280 (2.81), 217 (2.88) nm; {[α]20D + 20.0 (c 0.03, MeOH); CD (MeOH) Δε222 −0.54, Δε280+1.61; (+)-1}; {[α]20D −23.3 (c 0.03, MeOH); CD (MeOH) Δε222 +1.04, Δε280−4.55; (–)-1}; MS (ESI) m/z: 232 [M + H]+; HRMS (ESI) m/z: [M + H]+ Calcd for C11H13N5O 232.1193; found 232.1187; for 1H and 13C NMR data, see Table 1.
(±)-Cyclopeltain B (2): a yellowish solid; UV (MeOH) λmax (log
ε) 278 (2.61), 217 (2.78) nm; {[α]20D + 34.4 (c 0.03, MeOH); CD (MeOH) Δε218 +0.40, Δε271−2.84; (+)-2}; {[α]20D −33.3 (c 0.03, MeOH); CD (MeOH) Δε218−0.43, Δε271 +1.48; (–)-2}; MS (ESI) m/z: 232 [M + H]+; HRMS (ESI) m/z: [M + H]+ Calcd for C11H13N5O 232.1193; found 232.1188; for 1H and 13C NMR data, see Tables 1 and S1.†
ECD calculation for compounds 1 and 2
Molecular Merck force field (MMFF) and DFT/TDDFT calculations were performed with the Spartan'14 software package (Wavefunction Inc., Irvine, CA, USA) and the Gaussian09 program package.19 Conflex conformational search generated low-energy conformers within a 10 kcal mol−1 energy were furnished by the software CONFLEX 7. The predominant conformers were optimized by DFT calculation at the B3LYP/6-31G (d, p) level with the PCM in MeOH. ECD calculations further were conducted at the CAM-B3LYP/def2SVP level with the PCM in MeOH. For comparisons of the calculated curves and experimental CD spectra, the program SpecDis 1.62 was used.
Conflicts of interest
There are no conflicts of interest.
Acknowledgements
This study was supported by the National Key Research and Development Program of China (2017YFA0503900), Shenzhen Government's Plan of Science and Technology (JCYJ20170412110504956), the Shenzhen Fundamental Research Program (JCYJ20200109114225087), the National Science Fund for Distinguished Young Scholars (81525026), and the Guangdong Key Laboratory for Functional Substances in Medicinal Edible Resources and Healthcare Products (2021B1212040015).
Notes and references
- J. N. Wei, W. B. Shao, M. M. Cao, J. Ge, P. C. Yang, L. Chen, X. H. Wang and L. Kang, Phenylacetonitrile in locusts facilitates an antipredator defense by acting as an olfactory aposematic signal and cyanide precursor, Sci. Adv., 2019, 5, eaav5495 CrossRef PubMed.
- X. J. Guo, Q. Q. Yu, D. F. Chen, J. N. Wei, P. C. Yang, J. Yu, X. H. Wang and L. Kang, 4-Vinylanisole is an aggregation phenomone in locusts, Nature, 2020, 584, 584–588 CrossRef CAS PubMed.
- Y. M. Yan, T. Xu, Z. C. Tu, H. J. Zhu and Y. X. Cheng, Sulfur and nitrogen-containing compounds from the whole bodies of Blaps japanensis, Bioorg. Chem., 2020, 102, 104086 CrossRef CAS.
- Y. M. Yan, J. Ai, Y. N. Shi, Z. L. Zuo, B. Hou, J. Luo and Y. X. Cheng, (±)-Aspongamide A, an N-acetyldopamine trimer isolated from the insect Aspongopus chinensis, is an inhibitor of p-Smad3, Org. Lett., 2014, 16, 532–535 CrossRef CAS PubMed.
- W. Y. Ding, Y. M. Yan, X. H. Meng, L. A. Nafie, T. Xu, R. K. Dukor, H. B. Qin and Y. X. Cheng, Isolation, total synthesis, and absolute configuration determination of renoprotective dimeric N-acetyldopamine−adenine hybrids from the insect Aspongopus chinensis, Org. Lett., 2020, 22, 5726–5730 CrossRef CAS.
- Z. W. Song, W. P. Yin, P. Liu, J. F. Dong, Y. L. Jiang and T. Gao, Advances in chemical constituents and pharmacological effects of familiar Pentatomoids, Chin. J. Appl. Ent., 2011, 48, 753–756 CAS.
- Z. H. Yang, X. Y. Yang, X. H. Yuan, Z. H. Yang, X. Y. Yang, X. H. Yuan, K. M. Luo, X. Y. Luo, Y. J. Zhang, Y. L. Guo and Y. Pei, Purification of the antimicrobial peptide AMP-1 of Cyclopelta parva distant by HPLC, Southwest Agric. Univ., 2001, 23, 104–107 Search PubMed.
- Y. F. Wu, M. L. Cao, J. Tan and J. J. Guo, Comparison of the inhibitory effects between Aspongopus chinensis and Cyclopelta parva on proliferation of human gastric cancer cell SGC–7901, J. Mountain Agric. Bio., 2018, 37, 11–14 Search PubMed.
- H. Chen, Y. M. Yan, D. W. Wang and Y. X. Cheng, Parvaxanthines D–F and Asponguanosines C and D, racemic natural hybrids from the insect Cyclopelta parva, Molecules, 2021, 26, 3531 CrossRef CAS.
- J. Li, Y. P. Li, F. Y. Qin, Y. M. Yan, H. X. Zhang and Y. X. Cheng, Racemic xanthine and dihydroxydopamine conjugates from Cyclopelta parva and their COX-2 inhibitory activity, Fitoterapia, 2020, 142, 104534 CrossRef CAS PubMed.
- M. D. Kirnos, I. Y. Khudyakov, N. I. Alexandrushkina and B. F. Vanyushin, 2-aminoadenine is an adenine substituting for a base in S-2L cyanophage DNA, Nature, 1977, 270, 369–370 CrossRef CAS.
- Y. Zhou, X. X. Xu, Y. F. Wei, Y. Cheng, Y. Guo, I. Khudyakov, F. L. Liu, P. He, Z. Y. Song, Z. Li, Y. Gao, E. L. Ang, H. M. Zhao, Y. Zhang and S. W. Zhao, A widespread pathway for substitution of adenine by diaminopurine in phage genomes, Science, 2021, 372, 512–516 CrossRef CAS PubMed.
- G. R. Wyatt, Occurrence of 5-methylcytosine in nucleic acids, Nature, 1950, 166, 237–238 CrossRef CAS.
- B. F. Vanyushin, A. N. Belozersky, N. A. Kokurina and D. X. Kadirova, 5-Methylcytosine and 6-methylamino-purine in bacterial DNA, Nature, 1968, 218, 1066–1067 CrossRef CAS PubMed.
- Y. Fu, G. Z. Luo, K. Chen, X. Deng, M. Yu, D. Han, Z. Hao, J. Liu, X. Lu and L. Doré,
N
6-methyldeoxyadenosine marks active transcription start sites in Chlamydomonas, Cell, 2015, 161, 879–892 CrossRef CAS.
- E. L. Greer, M. A. Blanco, L. Gu, E. Sendinc, J. Z. Liu, D. Aristizábal-Corrales, C. H. Hsu, L. Aravind, C. He and Y. Shi, DNA methylation on N6-adenine in C. elegans, Cell, 2015, 161, 868–878 CrossRef CAS.
- G. Q. Zhang, H. Huang, D. Liu, Y. Cheng, X. L. Liu, W. X. Zhang, R. C. Yin, D. P. Zhang, P. Zhang, J. Z. Liu, C. Y. Li, B. D. Liu, Y. W. Luo, Y. X. Zhu, N. Zhang, S. M. He, C. He, H. L. Wang and D. H. Chen,
N
6-Methyladenine DNA modification in Drosophila, Cell, 2015, 161, 893–906 CrossRef CAS PubMed.
- L. Di, Y. N. Shi, Y. M. Yan, L. P. Jiang, B. Hou, X. L. Wang, Z. L. Zuo, Y. B. Chen, C. P. Yang and Y. X. Cheng, Nonpeptide small molecules from the insect Aspongopus chinensis and their neural stem cell proliferation stimulating properties, RSC Adv., 2015, 5, 70985–70991 RSC.
-
M. J. Frisch, G. W. Trucks, H. B. Schlegel, G. E. Scuseria, M. A. Robb, J. R. Cheeseman, G. Scalmani, V. Barone, B. Mennucci, G. A. Petersson, H. Nakatsuji, M. Caricato, X. Li, H. P. Hratchian, A. F. Izmaylov, J. Bloino, G. Zheng, J. L. Sonnenberg, M. Hada, M. Ehara, K. Toyota, R. Fukuda, J. Hasegawa, M. Ishida, T. Nakajima, Y. Honda, O. Kitao, H. Nakai, T. Vreven, J. A. Montgomery, J. E. Peralta, F. Ogliaro, M. Bearpark, J. J. Heyd, E. Brothers, K. N. Kudin, V. N. Staroverov, R. Kobayashi, J. Normand, K. Raghavachari, A. Rendell, J. C. Burant, S. S. Iyengar, J. Tomasi, M. Cossi, N. Rega, J. M. Millam, M. Klene, J. E. Knox, J. B. Cross, V. Bakken, C. Adamo, J. Jaramillo, R. Gomperts, R. E. Stratmann, O. Yazyev, A. J. Austin, R. Cammi, C. Pomelli, J. W. Ochterski, R. L. Martin, K. Morokuma, V. G. Zakrzewski, G. A. Voth, P. Salvador, J. J. Dannenberg, S. Dapprich, A. D. Daniels, O. Farkas, J. B. Foresman, J. V. Ortiz, J. Cioslowski and D. J. Fox, Gaussian 09, Revision D.01, Gaussian Inc., Wallingford, CT, 2009 Search PubMed.
Footnotes |
† Electronic supplementary information (ESI) available: NMR data for compound 2, NMR and HRESIMS spectra of 1 and 2, ECD calculations for 1 and 2, and bioassay methods. See DOI: 10.1039/d1qo01603b |
‡ These authors contributed equally. |
|
This journal is © the Partner Organisations 2022 |
Click here to see how this site uses Cookies. View our privacy policy here.