DOI:
10.1039/D1QO01083B
(Research Article)
Org. Chem. Front., 2022,
9, 51-57
A combined experimental and computational study of NHC-catalyzed allylation of allenoate with MBH esters: new regiospecific and stereoselective access to 1,5-enyne†
Received
23rd July 2021
, Accepted 9th November 2021
First published on 9th November 2021
Abstract
An NHC-catalyzed regiospecific allylation of α-substituted allenoates with MBH carbonates derived from aryl aldehyde furnished highly functionalized 1,5-enynes bearing a quaternary carbon successfully. Combining with DFT calculations, the reaction mechanism of this conversion was proposed. This method has the advantages of high regioselectivity, good yields and mild reaction conditions. This transformation not only provided a new access to 1,5-enyne, but also enriched the chemistry of allenoates and NHC catalysis.
Introduction
1,5-Enynes are important and versatile synthetic intermediates with tunable transformations into a diverse array of cyclic skeletons.1 So far, great achievements have been developed to assemble this valuable building block successfully, e.g., the allylation of propargylic electrophiles activated by either stoichiometric or catalytic amount of Lewis acids (Scheme 1a),2 the Pd-catalyzed cross-coupling of chiral propargyl acetates with allyl boronates (Scheme 1b).3 However, the development of an organocatalyzed, efficient and facile protocol to construct this skeleton is still highly desirable due to its synthetic significance.
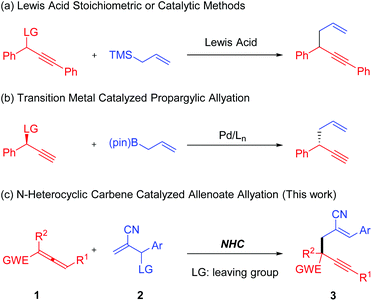 |
| Scheme 1 Selective strategies to achieve functionalized 1,5-enynes. | |
Allenoates are reported as important synthetic materials to construct a wealth of valuable structurally complex molecules.4 So far, numerous allenoate-involved reactions catalyzed by phosphine or tertiary amine have been disclosed since Lu's pioneering work on phosphine-promoted [3 + 2] cycloaddition of allenoates with electron-deficient olefins in 1995.5,6 Recently, N-heterocyclic carbenes (NHCs) have been widely employed to promote the conversions of aldehydes, carboxylic acids and carboxylic acids derivatives.7 As a type of Lewis bases, NHCs should also be able to facilitate those reactions catalyzed by phosphine or tertiary amine. Compared with the well-established phosphine or amine-catalyzed transformations of allenoates, in sharp contrast, the potential of the catalytic activity of NHC concerning the conversions of allenoates remains largely underexplored. The first study on the annulation variation was presented by Ye et al., where the imidazonium-based NHC was used to catalyze the [2 + 2 + 2] annulation of allenoate with two molecules of trifluoromethylketone, providing a six-membered cycloadduct.8
Subsequently, the Scheidt's group developed an NHC-catalyzed formal [2 + 2] annulation of γ-substituted allenoates with trifluoromethyl ketones for the diastereoselective assembly of oxetane.9 In terms of this, our group also reported a [4 + 2] annulation of chalcone with allenoate in the presence of imidazolium salt to build up a pyran scaffold with good chemoselectivity distinct from the previous works promoted by phosphine or tertiary amine (Scheme 2).10 In addition, NHC-catalyzed aldol-like condensations of allenoates with isatins have also been achieved by our lab recently.11
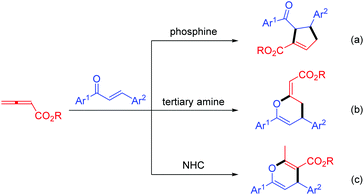 |
| Scheme 2 Reactions of allenoate with chalcone. | |
During the past decades, Morita–Baylis–Hillman (MBH) esters have evolved into important precursors for the syntheses of various complicated molecules and biologically active products.12 Besides, they could also act as allylation reagents to introduce allyl group into the organic molecules.13 For instance, our team described a regiodivergent allylation of N-acylhydrazones with MBH ester recently.14 To continue our studies on the NHC-catalyzed transformations of allenoates and the exploration of new reaction patterns of MBH esters and allenoates, herein we performed an NHC-catalyzed reaction of MBH esters with allenoates, affording highly functionalized 1,5-enynes with regiospecifically and excellent stereoselectively (Scheme 1c).
Results and discussion
Our investigation commenced with the screening of NHC catalysts for the model reaction of 1a and 2a (Table 1), which showed that triazole-based catalysts could not catalyze the reaction. Thiazole salts could deliver trace products, and only imidazole catalysts pushed the reaction forward successfully (Table 1, entries 1–4). The adjustment of electronic nature of the catalyst through the replacement of t-butyl with aryl groups (catalyst B, C) led to the striking decrease of reaction yield. At the same time, we found that the steric hindrance of the catalyst substituent had a great influence on the reaction for the employment of catalyst D lowered the yield substantially. In addition, the installment of a methyl group on the backbone of catalyst had detrimental impact on the reaction. After several attempts, we found that catalyst A was able to furnish product 3a in 71% yield under ambient temperature in THF presented by Cs2CO3 (Table 1, entry 1). Subsequently, other Lewis bases including phosphine and tertiary amine were assessed, but no corresponding product was obtained (Table 1, entries 5–7). The investigation of solvents demonstrated that THF was superior to other counterparts. Further studies showed that THF was better than other ether-type solvents (Table 1, entries 8–16). Slight enhancement of the reaction temperature had no positive effect on the reaction yield (Table 1, entry 22). It should be worth mentioning that the unemployment of 4 Å MS led to a dramatic decrease in yield (Table 1, entry 23). Additionally, the increase of the amount of Cs2CO3 could not elevate the yield of product 3a (Table 1, entry 24). Thus, the optimal reaction conditions were identified eventually.
Table 1 Survey on reaction conditions for the formation of 3aa
Having determined the optimal reaction conditions, the scope of variously substituted allenoates 1 and MBH esters 2 was investigated to delineate the influence of electronic and steric effects (Table 2). By placing a strong electron-donating group, such as CH3O, at the para-position of phenyl of R3, substituted 1,5-enyne 3ab was furnished in low yield (Table 2, entry 2). Even if the reaction time was extended to 72 hours, the corresponding MBH carbonate could not be consumed completely. The incorporation of a relatively weak electron-donating methyl group at positions C2, C3 or C4 of the phenyl ring of R3 did not affect the efficiency of this reaction substantially (Table 2, entries 3–5). However, the methyl group at ortho position of the phenyl ring of R3 retarded the formation of cis-product (3ae, Z/E = 5
:
1), presumably due to the negative steric hindrance effect (Table 2, entry 5). Installation of electron-withdrawing groups at positions C2, C3 or C4 of the phenyl ring in the MBH carbonates 2 had not any obvious impact on the reactivity of allylation either (Table 2, entries 6–10). Limitedly, MBH carbonate (2k) derived from aliphatic aldehyde could not take part in this reaction system (Table 2, entry 18). Subsequently, the allenoates 1 scope was investigated. The α-benzyl allenoate was also proved to be suitable in such type of conversion (Table 2, entries 11, 12 and 17). Among these substrates, the allenoate (1c) possessing γ-phenyl provided the desired products in excellent yields (Table 2, entries 13–16). Allenoates having α,γ-dimethyl (1e) or γ-phenyl (1f) could also afford a moderate yield of expected product (Table 2, entries 18 and 19). These results highlighted the wide substrate scope, regiospecificity and high stereoselectivity of NHC-catalyzed allylation of allenoate with MBH esters.
Table 2 Exploration of substrate scopea
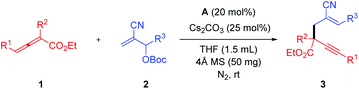
|
Entry |
R1/R2 |
R3 |
3, yieldb (%) |
t (h) |
Z/E ratioc |
Reactions were performed with 1a (0.12 mmol), 2a (0.10 mmol), A (0.02 mmol, 4.3 mg), and Cs2CO3 (0.025 mmol 8.1 mg) in THF (1.5 mL) under N2 at rt.
Isolated yields.
Z/E ratio determined by 1H NMR assay. NR = no reaction, ND = not detected.
|
1 |
H/CH3(1a) |
C6H5(2a) |
3aa, 71 |
12 |
20/1 |
2 |
H/CH3(1a) |
p-CH3OC6H4(2b) |
3ab, 36 |
72 |
20/1 |
3 |
H/CH3(1a) |
p-CH3C6H4(2c) |
3ac, 74 |
12 |
20/1 |
4 |
H/CH3(1a) |
m-CH3C6H4(2d) |
3ad, 68 |
12 |
20/1 |
5 |
H/CH3(1a) |
o-CH3C6H4(2e) |
3ae, 71 |
12 |
5/1 |
6 |
H/CH3(1a) |
p-ClC6H4(2f) |
3af, 82 |
12 |
20/1 |
7 |
H/CH3(1a) |
p-BrC6H4(2g) |
3ag, 76 |
12 |
20/1 |
8 |
H/CH3(1a) |
m-BrC6H4(2h) |
3ah, 78 |
12 |
20/1 |
9 |
H/CH3(1a) |
o-BrC6H4(2i) |
3ai, 59 |
12 |
20/1 |
10 |
H/CH3(1a) |
p-NO2C6H4(2j) |
3aj, 72 |
12 |
20/1 |
11 |
H/Bn(1b) |
C6H5(2a) |
3ba, 66 |
12 |
20/1 |
12 |
H/Bn(1b) |
p-ClC6H4(2f) |
3bf, 84 |
12 |
20/1 |
13 |
Ph/CH3(1c) |
C6H5(2a) |
3ca, 91 |
12 |
20/1 |
14 |
Ph/CH3(1c) |
p-CH3C6H4(2c) |
3cc, 88 |
12 |
20/1 |
15 |
Ph/CH3(1c) |
p-ClC6H4(2f) |
3cf, 92 |
12 |
20/1 |
16 |
Ph/CH3(1c) |
p-BrC6H4(2g) |
3cg, 90 |
12 |
20/1 |
17 |
CH3/Bn(1d) |
C6H5(2a) |
3da, 83 |
12 |
20/1 |
18 |
CH3/CH3(1e) |
C6H5(2a) |
3ea, 49 |
12 |
20/1 |
19 |
Bn/CH3(1f) |
C6H5(2a) |
3fa, 47 |
12 |
20/1 |
20 |
Ph/CH3(1c) |
C6H5CH2CH2(2k) |
3ck, NR |
24 |
ND |
Finally, an unsubstituted allenoate (1g) was subject to this protocol and the reaction system became so complicated that we tried our best to obtain a purified product (3ga) in poor yield. Single-crystal X-ray diffraction showed that it was formed by the reaction of allenoate and MBH in the molar ratio of 1
:
2,15 and therefore the molar ratio of allenoate to MBH ester was set up to 1
:
2 to simplify the reaction system. However, the reaction delivered the mixture as before, providing product 3ga in moderate yield (Scheme 3).
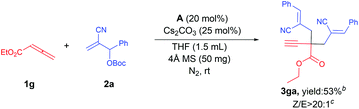 |
| Scheme 3 Reaction of Unsubstituted Allenoate with MBH Ester.aa Reactions were performed with 1a (0.10 mmol), 2a (0.20 mmol), A (0.02 mmol, 4.3 mg), and Cs2CO3 (0.025 mmol 8.1 mg) in THF (1.5 mL) under N2 at rt. b Isolated yields. c Z/E ratio determined by 1H NMR assay. | |
Based on our observations, substrate 3a was employed to illustrate the reaction process, as shown in Fig. 1 and 2. DFT calculations were performed to shed some light on the reaction mechanism, which confirmed that the possible catalytic cycle contains five steps. All the structures have been optimized at the M06-2X16/6-31G(d,p) level in THF solvent using the in SMD mode17 in the Gaussian 09 program,18 and then the frequencies were computed at the same level to confirm the expected structures and provide the zero-point and thermal corrections. Higher basis set was employed to refine the single-point energy, which was used to plus the free energy corrections. More details and computed results have been provided in ESI.† In the first step, the carbene carbon of NHC attacks on the allene via transition state TS1 with an energy barrier of 18.7 kcal mol−1. In the second step, the C–C bond formation occurs via transition state TS2 with an energy barrier of 13.5 kcal mol−1. We have also considered and excluded the C–C bond formation pathway associated with the γ-carbon position via transition state TS2′ with an energy barrier of 15.0 kcal mol−1. Subsequently, the OBoc− group can be dissociated via transition state TS3. It should be noted that the energy barrier via transition state TS3 is 2.4 kcal mol−1, but the energy difference between M2 and TS3 becomes negative (−0.5 kcal mol−1), indicating the reaction step is a barrier-less process. In addition, we have also scrutinized and ruled out the dissociation pathway associated with the Z-isomer via transition state TS3′ with an energy barrier of 3.2 kcal mol−1. In the fourth step, it is a deprotonation process via transition state TS4 with an energy barrier of 6.7 kcal mol−1. The last step is the dissociation of the product 3 and NHC catalyst via transition state TS5 with an energy barrier of 14.3 kcal mol−1.
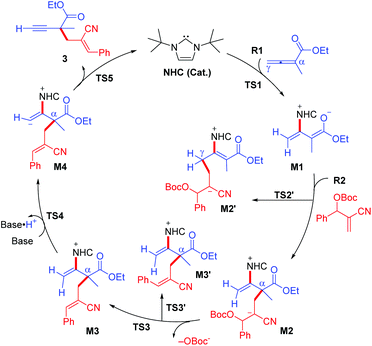 |
| Fig. 1 Our proposed reaction pathway. | |
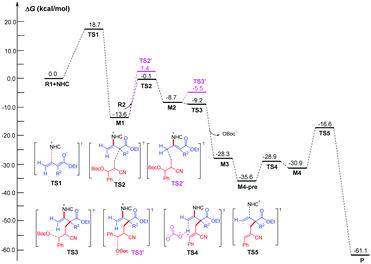 |
| Fig. 2 Relative Gibbs free-energy profile for NHC-catalyzed alkynylation by performing DFT calculate. | |
Conclusions
In summary, we have described a regiospecific allylation of α-substituted allenoate with MBH carbonate derived from aryl aldehyde, providing a facile access to the 1,5-enynes with multiple functional groups and a quaternary carbon in moderate to good yields with high E/Z selectivity. Further studies on the application of this protocol are underway in our lab.
Conflicts of interest
There are no conflicts of interest to declare.
Acknowledgements
We are grateful for financial support by National Natural Science Foundation of China (No. 21871113, 21773214, 21372101), a project Funded by the Priority Academic Program Development of Jiangsu Higher Education Institution and TAPP.
Notes and references
- For selected works, see:
(a) M. Nishida, N. Adachi, K. Onozuka, H. Matsumura and M. Mori, Intramolecular Cyclizations of Enynes Using RuClH(CO)(PPh3)3, J. Org. Chem., 1998, 63, 9158–9159 CrossRef CAS;
(b) O. Rhode and H. M. R. Hoffmann, Stereocontrolled Synthesis of Heteroannular Acetals from Functionalized 1,5-Enynes via Radical Cascades—Construction of Quaternary and 1,2-Diquaternary Centres in Polycyclic Systems, Tetrahedron, 2000, 56, 6479–6488 CrossRef CAS;
(c) M. R. Luzung, J. P. Markham and F. D. Toste, Catalaytic Isomerization of 1,5-Enynes to Bicyclo[3.1.0]hexenes, J. Am. Chem. Soc., 2004, 126, 10858–10859 CrossRef CAS;
(d) Y. Chen, D. M. Ho and C. Lee, Ruthenium-Catalyzed Hydrative Cyclization of 1,5-Enynes, J. Am. Chem. Soc., 2005, 127, 12184–12185 CrossRef CAS PubMed;
(e) J. Sun, M. P. Conley, L. Zhang and S. A. Kozmin, Au- and Pt-Catalyzed Cycloisomerizations of 1,5-Enynes to Cyclohexadienes with a Broad Alkyne Scope, J. Am. Chem. Soc., 2006, 128, 9705–9710 CrossRef CAS PubMed;
(f) S. Wang and L. Zhang, A Gold-Catalyzed Unique Cycloisomerization of 1,5-Enynes: Efficient Formation of 1-Carboxycyclohexa-1,4-dienes and Carboxyarenes, J. Am. Chem. Soc., 2006, 128, 14274–14275 CrossRef CAS PubMed;
(g) A. Buzas and F. Gagosz, Gold(I) Catalyzed Isomerization of 5-en-2-yn-1-yl Acetates: An Efficient Access to Acetoxy Bicyclo[3.1.0]hexenes and 2-Cycloalken-1-ones, J. Am. Chem. Soc., 2006, 128, 12614–12615 CrossRef CAS PubMed;
(h) O. Debleds and J.-M. Campagne, 1,5-Enyne Metathesis, J. Am. Chem. Soc., 2008, 130, 1562–1563 CrossRef CAS;
(i) K. Fukamizu, Y. Miyake and Y. Nishibayashi, Catalytic Cycloisomerization of 1,5-Enynes to 1,3-Cyclohexadienes via Ruthenium Vinylidene Intermediates, Angew. Chem., Int. Ed., 2009, 48, 2534–2537 CrossRef CAS PubMed;
(j) Y. Horino, T. Yamamoto, K. Ueda, S. Kuroda and F. D. Toste, Au(I)-Catalyzed Cycloisomerizations Terminated by sp3 C−H Bond Insertion, J. Am. Chem. Soc., 2009, 131, 2809–2811 CrossRef CAS;
(k) B. Crone, S. F. Kirsch and K.-D. Umland, Electrophilic Cyclization of 1,5-Enynes, Angew. Chem., Int. Ed., 2010, 49, 4661–4664 CrossRef CAS PubMed;
(l) D. Vasu, H.-H. Hung, S. Bhunia, S. A. Gawade, A. Das and R.-S. Liu, Gold-Catalyzed Oxidative Cyclization of 1,5-Enynes Using External Oxidants, Angew. Chem., Int. Ed., 2011, 50, 6911–6914 CrossRef CAS;
(m) C.-H. Chen, Y.-C. Tsai and R.-S. Liu, Gold-Catalyzed Cyclization/Oxidative [3 + 2] Cycloadditions of 1,5-Enynes with Nitrosobenzenes without Additional Oxidants, Angew. Chem., Int. Ed., 2013, 52, 4599–4603 CrossRef CAS;
(n) Z.-Z. Chen, S. Liu, W.-J. Hao, G. Xu, S. Wu, J.-N. Miao, B. Jiang, S.-L. Wang, S.-J. Tu and G. Li, Catalytic arylsulfonyl radical-triggered 1,5-enyne-bicyclizations and hydrosulfonylation of α,β-conjugates, Chem. Sci., 2015, 6, 6654–6658 RSC;
(o) K. Speck, K. Karaghiosoff and T. Magauer, Sequential O–H/C–H Bond Insertion of Phenols Initiated by the Gold(I)-Catalyzed Cyclization of 1-Bromo-1,5-enynes, Org. Lett., 2015, 17, 1982–1985 CrossRef CAS;
(p) G.-Q. Chen, W. Fang, Y. Wei, X.-Y. Tang and M. Shi, Gold(I)-catalyzed dehydrogenative cycloisomerization of 1,5-enynes, Chem. Commun., 2016, 52, 10799–10802 RSC;
(q) G.-Q. Chen, W. Fang, Y. Wei, X.-Y. Tang and M. Shi, Divergent reaction pathways in gold-catalyzed cycloisomerization of 1,5-enynes containing a cyclopropane ring: dramatic ortho substituent and temperature effects, Chem. Sci., 2016, 7, 4318–4328 RSC;
(r) L.-Z. Yu, Y. Wei and M. Shi, Synthesis of Polysubstituted Polycyclic Aromatic Hydrocarbons by Gold-Catalyzed Cyclization–Oxidation of Alkylidenecyclopropane-Containing 1,5-Enynes, ACS Catal., 2017, 7, 4242–4247 CrossRef CAS;
(s) S. K. Scott, J. N. Sanders, K. E. White, R. A. Yu, K. N. Houk and A. J. Grenning, Controlling, Understanding, and Redirecting the Thermal Rearrangement of 3,3-Dicyano-1,5-enynes, J. Am. Chem. Soc., 2018, 140, 16134–16139 CrossRef CAS;
(t) J. Guo, X. Peng, X. Wang, F. Xie, X. Zhang, G. Liang, Z. Sun, Y. Liu, M. Cheng and Y. Liu, A gold-catalyzed cycloisomerization/aerobic oxidation cascade strategy for 2-aryl indenones from 1,5-enynes, Org. Biomol. Chem., 2018, 16, 9147–9151 RSC.
- For selected works, see:
(a) S. L. Schreiber, T. Sammakia and W. E. Crowe, A Lewis acid-mediated version of the Nicholas reaction: Synthesis of syn-alkylated products and cobalt-complexed cycloalkynes, J. Am. Chem. Soc., 1986, 108, 3128–3130 CrossRef CAS;
(b) M. Hayashi, A. Inubushi and T. Mukaiyama, A Convenient Method for the Preparation of Secondary Propargylic Ethers. The Reaction of Acetals with 1-Trimethylsilylalkynes Promoted by the Combined Use of Catalytic Amounts of Tin(IV) Chloride and Zinc Chloride, Chem. Lett., 1987, 16, 1975–1978 CrossRef;
(c) J.-P. Dau-Schmidt and H. Mayr, Relative Reactivities of Alkyl Chlorides under Friedel-Crafts Conditions, Chem. Ber., 1994, 127, 205–212 CrossRef CAS;
(d) T. J. J. Müller and A. Netz, SN1 reactions with planar chiral (arene)Cr(CO)3-substituted α-propargyl cations - regio- and diastereoselective additions to novel ambident electrophiles, Tetrahedron Lett., 1999, 40, 3145–3148 CrossRef;
(e) T. Ishikawa, M. Okano, T. Aikawa and S. Saito, Novel Carbon–Carbon Bond-Forming Reactions Using Carbocations Produced from Substituted Propargyl Silyl Ethers by the Action of TMSOTf, J. Org. Chem., 2001, 66, 4635–4642 CrossRef CAS PubMed;
(f) M. R. Luzung and F. D. Toste, Rhenium-Catalyzed Coupling of Propargyl Alcohols and Allyl Silanes, J. Am. Chem. Soc., 2003, 125, 15760–15761 CrossRef CAS;
(g) T. Schwier, M. Rubin and V. Gevorgyan, B(C6F5)3-Catalyzed Allylation of Propargyl Acetates with Allylsilanes, Org. Lett., 2004, 6, 1999–2001 CrossRef CAS PubMed;
(h) Y. Kuninobu, E. Ishii and K. Takai, Rhenium- and Gold-Catalyzed Coupling of Aromatic Aldehydes with Trimethyl(phenylethynyl)silane: Synthesis of Diethynylmethanes, Angew. Chem., Int. Ed., 2007, 46, 3296–3299 CrossRef CAS;
(i) G. W. Kabalka, M.-L. Yao and S. Borella, Generation of Cations from Alkoxides: Allylation of Propargyl Alcohols, J. Am. Chem. Soc., 2006, 128, 11320–11321 CrossRef CAS PubMed;
(j) Z.-P. Zhan, J.-L. Yu, H.-J. Liu, Y.-Y. Cui, R.-F. Yang, W.-Z. Yang and J.-P. Li, A General and Efficient FeCl3-Catalyzed Nucleophilic Substitution of Propargylic Alcohols, J. Org. Chem., 2006, 71, 8298–8301 CrossRef CAS PubMed;
(k) R. Sanz, A. Martínez, D. Miguel, J. M. Álvarez-Gutiérrez and F. Rodríguez, Synthesis of 1,5-Enynes by Brønsted Acid Catalyzed Substitution of Propargylic Alcohols and One-Pot Synthesis of Bicyclo[3.1.0]hexenes, Synthesis, 2007, 3252–3256 CrossRef CAS;
(l) A. J. Lepore, D. M. Pinkerton and B. L. Ashfeld, Relay Redox and Lewis Acid Catalysis in the Titanocene- Catalyzed Multicomponent Assembly of 1,5-Enynes, Adv. Synth. Catal., 2013, 355, 1500–1504 CrossRef CAS;
(m) J. Mao, H. Li, H. Wen, M. Li, X. Fan and W. Bao, Palladium-Catalyzed Two-Component Domino Coupling Reaction of (Z)-β-Bromostyrenes with Norbornenes: Synthesis of 1,5-Enynes, Adv. Synth. Catal., 2016, 358, 1873–1879 CrossRef CAS.
- M. J. Ardolino and J. P. Morken, Construction of 1,5-Enynes by Stereospecific Pd-Catalyzed Allyl–Propargyl Cross-Couplings, J. Am. Chem. Soc., 2012, 134, 8770–8773 CrossRef CAS PubMed.
- For selected works, see:
(a) S. Ma, Electrophilic Addition and Cyclization Reactions of Allenes, Acc. Chem. Res., 2009, 42, 1679–1688 CrossRef CAS PubMed;
(b) B. J. Cowen and S. J. Miller, Enantioselective catalysis and complexity generation from allenoates, Chem. Soc. Rev., 2009, 38, 3102–3116 RSC;
(c) C.-K. Pei and M. Shi, Asymmetric Cyclization Reactions of Allenoates with Imines or α,β-Unsaturated
Ketones Catalyzed by Organocatalysts Derived from Cinchona Alkaloids, Chem. – Eur. J., 2012, 18, 6712–6716 CrossRef CAS PubMed;
(d) S. Yu and S. Ma, Allenes in Catalytic Asymmetric Synthesis and Natural Product Syntheses, Angew. Chem., Int. Ed., 2012, 51, 3074–3112 CrossRef CAS;
(e) F. López and J. L. Mascareñas, [4 + 2] and [4 + 3] catalytic cycloadditions of allenes, Chem. Soc. Rev., 2014, 43, 2904–2915 RSC;
(f) Z. Wang, X. Xu and O. Kwon, Phosphine catalysis of allenes with electrophiles, Chem. Soc. Rev., 2014, 43, 2927–2940 RSC;
(g) T. Wang, X. Han, F. Zhong, W. Yao and Y. Lu, Amino Acid-Derived Bifunctional Phosphines for Enantioselective Transformations, Acc. Chem. Res., 2016, 49, 1369–1378 CrossRef CAS;
(h) W. Zhou, H. Wang, M. Tao, C.-Z. Zhu, T.-Y. Lin and J. Zhang, Phosphine-catalyzed enantioselective [3 + 2] cycloadditions of γ-substituted allenoates with β-perfluoroalkyl enones, Chem. Sci., 2017, 8, 4660–4665 RSC.
- C. Zhang and X. Lu, Phosphine-Catalyzed Cycloaddition of 2,3-Butadienoates or 2-Butynoates with Electron-Deficient Olefins. A Novel [3 + 2] Annulation Approach to Cyclopentenes, J. Org. Chem., 1995, 60, 2906–2908 CrossRef CAS.
- For reviews, see:
(a) B. J. Cowen and S. J. Miller, Enantioselective catalysis and complexity generation from allenoates, Chem. Soc. Rev., 2009, 38, 3102–3116 RSC;
(b) S. Ma, Electrophilic Addition and Cyclization Reactions of Allenes, Acc. Chem. Res., 2009, 42, 1679–1688 CrossRef CAS;
(c) H. Li and Y. Lu, Enantioselective Construction of All-Carbon Quaternary Stereogenic Centers by Using Phosphine Catalysis, Asian J. Org. Chem., 2017, 6, 1130–1145 CrossRef CAS;
(d) H. Guo, Y. C. Fan, Z. Sun, Y. Wu and O. Kwon, Phosphine Organocatalysis, Chem. Rev., 2018, 118, 10049–10293 CrossRef CAS PubMed;
(e) E.-Q. Li and Y. Huang, Recent advances in phosphine catalysis involving γ-substituted allenoates, Chem. Commun., 2020, 56, 680–694 RSC.
-
(a) D. M. Flanigan, F. Romanov-Michailidis, N. A. White and T. Rovis, Organocatalytic Reactions Enabled by N-Heterocyclic Carbenes, Chem. Rev., 2015, 115, 9307–9387 CrossRef CAS PubMed;
(b) D. Enders, O. Niemeier and A. Henseler, Organocatalysis by N-Heterocyclic Carbenes, Chem. Rev., 2007, 107, 5606–5655 CrossRef CAS PubMed;
(c) X. Bugaut and F. Glorius, Organocatalytic umpolung: N-heterocyclic carbenes and beyond, Chem. Soc. Rev., 2012, 41, 3511–3522 RSC;
(d) Y. Que, T. Li, C. Yu, X.-S. Wang and C. Yao, Enantioselective Assembly of Spirocyclic Oxindole-dihydropyranones through NHC-Catalyzed Cascade Reaction of Isatins with N-Hydroxybenzotriazole Esters of α,β-Unsaturated Carboxylic Acid, J. Org. Chem., 2015, 80, 3289–3294 CrossRef CAS PubMed;
(e) Y. Que, Y. Xie, T. Li, C. Yu, S. Tu and C. Yao, An N-Heterocyclic Carbene-Catalyzed Oxidative γ-Aminoalkylation of Saturated Carboxylic Acids through in Situ Activation Strategy: Access to δ-Lactam, Org. Lett., 2015, 17, 6234–6237 CrossRef CAS PubMed;
(f) Y. Wang, J. Pan, J. Dong, C. Yu, T. Li, X.-S. Wang, S. Shen and C. Yao, N-Heterocyclic Carbene-Catalyzed [4 + 2] Cyclization of Saturated Carboxylic Acid with o-Quinone Methides through in Situ Activation: Enantioselective Synthesis of Dihydrocoumarins, J. Org. Chem., 2017, 82, 1790–1795 CrossRef CAS;
(g) Y. Hu, D. Pan, L. Cong, Y. Yao, C. Yu, T. Li and C. Yao, NHC-Catalyzed Efficient Syntheses of Isoquinolinones or Isochromanones through Formal [4 + 2] Cycloaddition of o-Quinodimethanes with Acylhydrazones or Ketones, ChemistrySelect, 2018, 3, 1708–1712 CrossRef CAS.
- L. Sun, T. Wang and S. Ye, N-Heterocyclic Carbene-Catalyzed [2 + 2 + 2] Annulation of Allenoates with Trifluoromethylketones, Chin.
J. Chem., 2012, 30, 190–194 CrossRef CAS.
- S. S. Lopez, A. A. Jaworski and K. A. Scheidt, NHC-Catalyzed Formal [2 + 2] Annulations of Allenoates for the Synthesis of Substituted Oxetanes, J. Org. Chem., 2018, 83, 14637–14645 CrossRef CAS.
-
(a) A. Voituriez, A. Panossian, N. Fleury-Brégeot, P. Retailleau and A. Marinetti, 2-Phospha[3]ferrocenophanes with Planar Chirality: Synthesis and Use in Enantioselective Organocatalytic [3 + 2] Cyclizations, J. Am. Chem. Soc., 2008, 130, 14030–14031 CrossRef CAS;
(b) K. D. Ashtekar, R. J. Staples and B. Borhan, Development of a Formal Catalytic Asymmetric [4 + 2] Addition of Ethyl-2,3-butadienoate with Acyclic Enones, Org. Lett., 2011, 13, 5732–5735 CrossRef CAS PubMed;
(c) Y. Hu, S. Li, Z. Wang, Y. Yao, T. Li, C. Yu and C. Yao, NHC-Catalyzed Hetero-Diels–Alder Reaction of Allenoate with Chalcone: Synthesis of Polysubstituted Pyranyl Carboxylate, J. Org. Chem., 2018, 83, 3361–3366 CrossRef CAS.
- S. Li, Z. Tang, Y. Wang, D. Wang, Z. Wang, C. Yu, T. Li, D. Wei and C. Yao, NHC-Catalyzed Aldol-Like Reactions of Allenoates with Isatins: Regiospecific Syntheses of γ-Functionalized Allenoates, Org. Lett., 2019, 21, 1306–1310 CrossRef CAS PubMed.
- For reviews, see:
(a) V. Singh and S. Batra, Advances in the Baylis–Hillman reaction-assisted synthesis of cyclic frameworks, Tetrahedron, 2008, 64, 4511–4574 CrossRef CAS;
(b) G.-N. Ma, J.-J. Jiang, M. Shi and Y. Wei, Recent extensions of the Morita–Baylis–Hillman reaction, Chem. Commun., 2009, 5496–5514 RSC;
(c) D. Basavaiah, B. S. Reddy and S. S. Badsara, Recent Contributions from the Baylis−Hillman Reaction to Organic Chemistry, Chem. Rev., 2010, 110, 5447–5674 CrossRef CAS PubMed;
(d) T.-Y. Liu, M. Xie and Y.-C. Chen, Organocatalytic asymmetric transformations of modified Morita–Baylis–Hillman adducts, Chem. Soc. Rev., 2012, 41, 4101–4112 RSC;
(e) N.-J. Zhong, Y.-Z. Wang, L. Cheng, D. Wang and L. Liu, Recent advances in the annulation of Morita–Baylis–Hillman adducts, Org. Biomol. Chem., 2018, 16, 5214–5227 RSC.
-
(a) C.-W. Cho, J.-R. Kong and M. J. Krische, Phosphine-Catalyzed Regiospecific Allylic Amination and Dynamic Kinetic Resolution of Morita–Baylis–Hillman Acetates, Org. Lett., 2004, 6, 1337–1339 CrossRef CAS PubMed;
(b) J. Liu, Z. Han, X. Wang, F. Meng, Z. Wang and K. Ding, Palladium-Catalyzed Asymmetric Construction of Vicinal Tertiary and All-Carbon Quaternary Stereocenters by Allylation of β-Ketocarbonyls with Morita–Baylis–Hillman Adducts, Angew. Chem., Int. Ed., 2017, 56, 5050–5054 CrossRef CAS;
(c) Q.-Q. Zhao, J. Chen, D.-M. Yan, J.-R. Chen and W.-J. Xiao, Photocatalytic Hydrazonyl Radical-Mediated Radical Cyclization/Allylation Cascade: Synthesis of Dihydropyrazoles and Tetrahydropyridazines, Org. Lett., 2017, 19, 3620–3623 CrossRef CAS PubMed;
(d) J. Qi, J. Zheng and S. Cui, Fe(III)-Catalyzed Hydroallylation of Unactivated Alkenes with Morita–Baylis–Hillman Adducts, Org. Lett., 2018, 20, 1355–1358 CrossRef CAS PubMed;
(e) G.-Y. Chen, F. Zhong and Y. Lu, Highly Enantioselective and Regioselective Substitution of Morita–Baylis–Hillman Carbonates with Nitroalkanes, Org. Lett., 2011, 13, 6070–6073 CrossRef CAS PubMed.
- F. Sun, T. Yin, A. Feng, Y. Hu, C. Yu, T. Li and C. Yao, Base-promoted regiodivergent allylation of N-acylhydrazones with Morita–Baylis–Hillman carbonates by tuning the catalyst, Org. Biomol. Chem., 2019, 17, 5283–5293 RSC.
- Crystallographic data (excluding structure factors) for the structures (3ga) in this paper have been deposited with the Cambridge Crystallographic Data Centre as supplementary publication no. CCDC 2117224.†.
-
(a) Y. Zhao and D. G. Truhlar, Exploring the Limit of Accuracy of the Global Hybrid Meta Density Functional for Main-Group Thermochemistry, Kinetics, and Noncovalent Interactions, J. Chem. Theory Comput., 2008, 4, 1849–1868 CrossRef CAS PubMed;
(b) Y. Zhao and D. G. Truhlar, Density Functionals with Broad Applicability in Chemistry, Acc. Chem. Res., 2008, 41, 157–167 CrossRef CAS PubMed.
- A. V. Marenich, C. J. Cramer and D. G. Truhlar, Universal Solvation Model Based on Solute Electron Density and on a Continuum Model of the Solvent Defined by the Bulk Dielectric Constant and Atomic Surface Tensions, J. Phys. Chem. B, 2009, 113, 6378–6396 CrossRef CAS PubMed.
-
G. W. Trucks, M. J. Frisch, H. B. Schlegel, G. E. Scuseria, M. A. Robb, J. R. Cheeseman, G. Scalmani, V. Barone, B. Mennucci, G. A. Petersson, H. Nakatsuji, M. Caricato, X. Li, H. P. Hratchian, A. F. Izmaylov, J. Bloino, G. Zheng, J. L. Sonnenberg, M. Hada, M. Ehara, K. Toyota, R. Fukuda, J. Hasegawa, M. Ishida, T. Nakajima, Y. Honda, O. Kitao, H. Nakai, T. Vreven, J. A. Montgomery, Jr., J. E. Peralta, F. Ogliaro, M. Bearpark, J. J. Heyd, E. Brothers, K. N. Kudin, V. N. Staroverov, T. Keith, R. Kobayashi, J. Normand, K. Raghavachari, A. Rendell, J. C. Burant, S. S. Iyengar, J. Tomasi, M. Cossi, N. Rega, J. M. Millam, M. Klene, J. E. Knox, J. B. Cross, V. Bakken, C. Adamo, J. Jaramillo, R. Gomperts, R. E. Stratmann, O. Yazyev, A. J. Austin, R. Cammi, C. Pomelli, J. W. Ochterski, R. L. Martin, K. Morokuma, V. G. Zakrzewski, G. A. Voth, P. Salvador, J. J. Dannenberg, S. Dapprich, A. D. Daniels, O. Farkas, J. B. Foresman, J. V. Ortiz, J. Cioslowski and D. J. Fox, Revision C.01, 2010 Search PubMed.
Footnotes |
† Electronic supplementary information (ESI) available. CCDC 2117224. For ESI and crystallographic data in CIF or other electronic format see DOI: 10.1039/d1qo01083b |
‡ These authors contributed equally to this work. |
|
This journal is © the Partner Organisations 2022 |
Click here to see how this site uses Cookies. View our privacy policy here.