DOI:
10.1039/D1OB01791H
(Communication)
Org. Biomol. Chem., 2022,
20, 98-105
Pyrrovobasine, hybrid alkylated pyrraline monoterpene indole alkaloid pseudodimer discovered using a combination of mass spectral and NMR-based machine learning annotations†
Received
12th September 2021
, Accepted 27th September 2021
First published on 27th September 2021
Abstract
A new vobasine–tryptamine-based monoterpene indole alkaloid pseudodimer was isolated from the stem bark of Voacanga africana. As a minor constituent occurring in a thoroughly investigated plant, this molecule was targeted based on a molecular networking strategy and a rational MS2-guided phytochemical investigation led to its isolation. Its structure was formally established based on HRMS, 1D/2D NMR data, and the application of the tool Small Molecule Accurate Recognition Technology (SMART 2.0). Its absolute configuration was assigned by the exciton chirality method and TD-DFT ECD calculations. Besides featuring an unprecedented intermonomeric linkage in the small group of vobasine/tryptamine hybrids, pyrrovobasine also represents the first pyrraline-containing representative in the whole monoterpene indole alkaloids group. Biosynthetic hypotheses possibly underpinning these structural oddities are proposed here.
Introduction
Fueled by the diverse ethnopharmacological claims related to Voacanga africana Stapf ex Scott-Elliot, decades of intensive phytochemical campaigns unveiled the array of specialized products it shelters with a salient emphasis on its monoterpene indole alkaloid (MIA) content. Notwithstanding the long-held interest in V. africana chemistry, new appendages of MIAs are continuously being reported from this plant source.1–3 The tremendous advances in MS2-based data processing strategies and in sensitivity of NMR techniques provide natural product chemists with ever-expanding capabilities for exploration of compounds occurring in minute amounts.4,5 These refined analytical workflows pave the way for the re-investigation of deeply dug models using a state-of-the-art pipeline to gear the isolation efforts towards so-far overlooked chemical entities.6–12 In the frame of our quest for new MIA-type specialized metabolites, and benefitting from our recent upload to the GNPS spectral libraries of the MIADB,13 we embarked on a molecular-networking-based phytochemical investigation of V. africana stem bark. The observation of a molecular family clustered around a node tentatively tagged as ceridimine, a rare vobasine/tryptamine-type pseudodimer so far only known from Pagiantha cerifera (Pancher & Sebert) Markgr.,14 sparked our interest and guided us to isolate the newly-reported pyrrovobasine (1) following a MS2-streamlined isolation workflow. Besides representing the first instance of vobasine–tryptamine dimer featuring a C-3–C-2′ interunit bonding,15,16 pyrrovobasine is also the first pyrraline-containing member in the huge phytochemical class of MIAs.
Results and discussion
To reach a wide and untargeted insight into the indolomonoterpenic alkaloid content of V. africana, both the alkaloid and crude ethanolic extracts of its stem bark were profiled by HPLC-HRMS2 (positive polarity). These data were subsequently processed using the classical molecular-networking workflow17 and dereplicated against the MIADB-implemented GNPS spectral libraries. A cautious examination of the obtained molecular network (Fig. S1†) highlighted a molecular family consisting of four nodes (Fig. S2†) instigating a connection with a central node tentatively tagged as ceridimine. This constellation held a peculiar signifiance as only very few derivatives of ceridimine,14 pertaining to the rare phytochemical class of vobasine–tryptamine pseudodimers, have been reported so far with none being consistent with the currently disclosed nominal masses. With this in mind, the LC-DAD-MS profile related to the alkaloidal extract of V. africana was monitored to check which of these compounds could be isolated in sufficient yield for full structure elucidation, leading us to guide our isolation efforts towards the ion detected at m/z 605.303. Notwithstanding its relatively elevated molecular mass, the mass spectrum of this compound lacked the diagnostic isotopic pattern for doubly charged ion species encountered in most dimeric monoterpene indole alkaloids. This observation hinted that this molecule comprises a unique basic site, which strengthened our interest in isolating it. The molecular formula retrieved from this mass spectrometric signal, C37H40N4O4, returned no hit in the Dictionary of Natural Products,18 deftly convincing us to undertake its MS2-streamlined isolation.
Compound 1 (Fig. 1) was isolated from the alkaloidic extract of V. africana stem bark by repetitive chromatographic separations following a MS2-guided phytochemical pipeline. Obtained as a brown amorphous solid, its molecular formula was confirmed to be C37H40N4O4 based on the protonated molecular ion at m/z 605.3122 (calcd for C37H41N4O4, 605.3122), indicating 20 degrees of hydrogen deficiency. The cursory examination of the NMR data of 1 revealed NMR landmarks evocative of a 3-vobasinyl unit.19 Accordingly, the 1H and 13C NMR data of 1 showcased the presence of four aromatic resonances, an upfield-shifted methyl ester group (δH 2.49/δC 169.2; 51.0), an N4-Me group (δH 2.89/δC 40.5) and an ethylidene side chain (δH 5.60/δC 126.6; δH 1.73/δC 13.0). Alongside the crosspeaks related to the contiguous aromatic hydrogens of the indole core, the COSY spectrum confirmed the occurrence of an isolated aminomethylene (C-21), of an ethylidene fragment, and of an extended CH2CHCHCHCH2CH spin system, displaying chemical shifts diagnostic of the C6–C5–C16–C15–C14–C3 appendage of a vobasine-type MIA, further validated by the key HMBC crosspeaks outlined in Fig. 2B. The shielded chemical shift of the ester methyl linked to C-16 placed it within the shielding zone of the indole nucleus.6
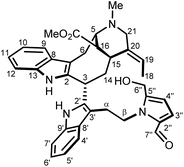 |
| Fig. 1 Chemical structure of pyrrovobasine (1). | |
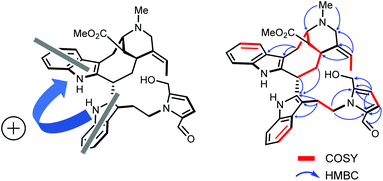 |
| Fig. 2 (A) Two electronic dipoles denoting a positive chirality. (B) Selected 2D NMR correlations for 1. | |
Consistent with the molecular network which determined 1 as a close derivative of ceridimine, the remaining signals hinted the occurrence of an additional free side chain-bearing tryptamine unit. Four additional resonances related to aromatic/olefinic protons substantiated this assumption, as well as the two vicinal methylenic protons that determined a CH2CH2N fragment. The structural elements elucidated so far determined 1 as a vobasine–tryptamine pseudodimer. This small phytochemical class is so far represented by three molecules, all obtained from an apocynaceous source: ceridimine (from P. cerifera),14 demethylceridimine (from the by-then Peschiera buchtienii (H.J.P.Winkl.) Markgr.,20 now known as Tabermaemontana cymosa Jacq.), and hunteriatryptamine (from Hunteria zeylanica (Retz.) Gardner ex Thwaites).21 All these closely related molecules featured a linkage between the C-3 position of the vobasinyl residue to the C-6′ site of the tryptamine component. Regarding 1, the HMBC correlations from the protons resonating at δH 2.02 (H-14), at δH 4.87 (H-3), and at δH 3.42 (H2-α) to C-2′ (δC 137.9) were diagnostic of a so-far unprecedented C-3–C-2′ connection between the vobasine and tryptamine units. The β-orientation of H-3 was established based on the magnitude of the coupling constant values (dd, J = 13.4, 2.7 Hz), in excellent agreement with former literature reports.22
At this stage of the structure elucidation process, a few signal patterns were still pending assignment: two contiguous aromatic/olefinic protons disclosing an unusual coupling constant value of 3.9 Hz [δH 6.97 (1H, d, J = 3.9 Hz)/δC 124.3; δH 6.30 (1H, d, J = 3.9 Hz)/δC 110.7], a shielded aldehydic proton [δH 9.56 (1H, s)/δC 179.9], a set of oxygenated methylenic protons [δH 4.63 (1H, d, J = 13.5 Hz) and 4.77 (1H, d, J = 13.5 Hz)/δC 56.7], and finally two low-field shifted quaternary carbons (δC 141.9 and 132.9). To expedite the elucidation of this specific fragment, the HSQC data retrieved from it were collated in a .csv file prior to being uploaded into the SMART 2.0 (Small Molecule Accurate Recognition Technology) platform. This artificial intelligence-based tool is used to generate structure hypotheses from HSQC data through a deep convolutional neural network architecture that includes the 1H–13C HSQC spectra related to more than 53
000 natural products (encompassing approximately 15% of the known natural products as of 2020).23,24 SMART analysis revealed 5-(hydroxymethyl)pyrrole-2-carbaldehyde, or pyrraline, to be the preferred hit (cosine value ≈ 0.97), guiding us to consider it to account for the so far unassigned structural elements. Accordingly, SMART assistance tended to elucidate the structure of 1 as indicated in Fig. 1, in good agreement with molecular formula requirements. The key HMBC correlations outlined in Fig. 2B confirmed this tentative structure as the nitrogen atom of the pyrrole nucleus was shown to correspond to that of the side chain of the tryptamine unit, as evidenced based on the HMBC correlations from the protons resonating at δH 4.84 (H2-β) to both C-2′′ (δC 132.9) and C-5′′ (δC 141.9). A rapid literature survey indicated that pyrraline-containing natural products display highly similar chemical shifts values, also for aromatic quaternaries left unconsidered throughout SMART analyses.25 The absolute configuration of 1 was determined using the exciton chirality CD method.26 The positive sign of the first Cotton effect [λmax 237 nm (Δε + 31)] and the negative sign of the second one [λmax 222 nm (Δε − 21)] (Fig. 3) indicated a right-handed screw between the two indole chromophores (Fig. 2A).27 The good fit between the calculated ECD spectrum of the lowest-energy conformer of 1 and the experimental ECD spectrum confirmed the proposed absolute configuration (Fig. 3). These structural features allowed to elucidate the structure of 1, namely pyrrovobasine, as shown in Fig. 1.
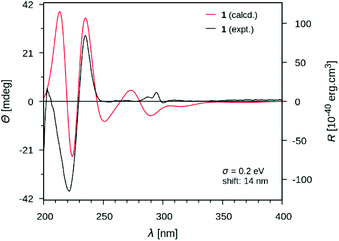 |
| Fig. 3 ECD spectra of 1 (experimental and calculated at the B3LYP/6-31G* level). | |
Pyrrovobasine features several structural oddities which are worth being stressed out. As already pinpointed, the nature of the connection between the tryptamine and the vobasine subunits meets no precedents in this small group of pseudodimers. The electrophilic nature of vobasinol C-3 (Fig. 4) is illustrious and, as such, dimeric MIA structures disclosing a 3-vobasinyl residue following its attack by an electron-rich center are of considerable generality.15 As per the tryptamine component, a literature survey indicates that an array of different substituents can be encountered at a tryptaminic C-2 position such as prenyl groups,28 bromine atoms,29 methyl groups,30 a beta-carboline,31 or may even lead to disulfide-bonded dimeric structures.32 The depiction of the resonance contributors related to the indolic system reveals that all non-bridgehead carbons can serve as functional carbanion equivalents.33,34
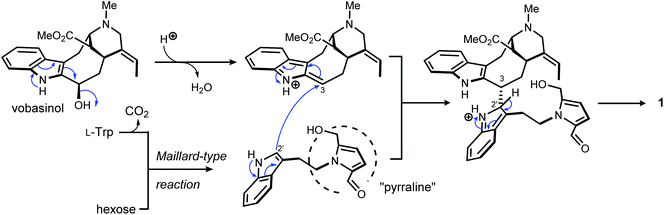 |
| Fig. 4 Possible biosynthetic logic of pyrrovobasine (1). | |
At last, 5-hydroxymethylpyrrole-2-carbaldehyde, sometimes referred to as pyrraline, is known from a wealth of natural sources, but also from cooked foods.35 No enzymatic biosynthetic pathway to this nucleus has been proposed so far and it is rather believed that this ring system arises by condensation of amines and sugars, following the so-called Maillard reaction (Fig. 4).36 Consistent with this viewpoint, 2-formylpyrrole-containing natural substances are ubiquitous in nature and these molecules – recently reviewed by Brimble et al. are classified based on the nature of their amine component, falling into three major types: amino acids, biogenic amines or amino sugars.35 Tryptamine-based pyrrole alkaloids have already been reported from macromycetes in particular.37,38 Yet, to the best of our knowledge, pyrrovobasine represents the first example of pyrraline-containing monoterpene indole alkaloid.
The in vitro antibacterial activities of compound 1 were evaluated against Mycobacterium smegmatis, M. abscessus, M. bovis BCG, Staphylococcus aureus and Pseudomonas aeruginosa. The results showed that pyrrovobasine (1) exerts no antibacterial activity (MIC > 50 μg mL−1).
Conclusions
In summary, we have described the first member of the pyrrovobasine family of natural products from the stem bark of V. africana, representing the first example of a pyrraline-containing monoterpene indole alkaloid. The isolation process was enabled by exploiting a molecular networking approach and the structure elucidation was assisted by an NMR-based machine learning tool.
Experimental section
General experimental procedures
Optical rotations were measured at 25 °C on a Polaar 32 polarimeter. UV spectra were recorded on a Lightwave II + WPA 7126 V. 1.6.1 spectrophotometer. ECD spectra were measured at 25 °C on a JASCO J-810 spectropolarimeter. IR spectra were recorded with a PerkinElmer type 257 spectrometer. The NMR spectra were recorded on a Bruker AM-600 (600 MHz) NMR spectrometer equipped with a TCI 5 mm cryoprobe using CDCl3 as a solvent. The solvent signal was used as a reference. Sunfire preparative C18 columns (150 × 19 mm, i.d. 5 μm and 150 × 30 mm, i.d. 5 μm; Waters) were used for preparative HPLC separations using a Waters Delta Prep equipped with a binary pump (Waters 2525) and a UV–visible diode array detector (190–600 nm, Waters 2996). Silica 330 g, 120 g, and 24 g Grace cartridges were used for flash chromatography using an Armen Instrument spot liquid chromatography flash apparatus. Chemicals and solvents were purchased from Sigma-Aldrich.
Plant material
The fresh bark of V. africana (Yaounde National Herbarium voucher specimen No. HNY/1949; Victor Nana (collector)) was collected from the campus of the University of Yaounde I, Cameroon in December 2019.
Extraction and isolation
The air-dried and powdered bark of V. africana (2.3 kg) was extracted three times with MeOH at room temperature. The resulting extract was concentrated under reduced pressure to obtain a crude extract (231 g). The obtain extract was dissolved in the mixture MeOH–H2O 10% and alkalinized with NH3 (pH = 12) and extracted with CH2Cl2 (3 × 1.5 L, 1 h each, 20 °C, atmospheric pressure). The mixture was concentrated under vacuum at 38 °C to yield 50.5 g of an alkaloid extract. A part of this residue (VADCM, 25 g) was subjected to flash chromatography using a silica 330 g Grace cartridge with a gradient of CH2Cl2–MeOH (100
:
0 to 0
:
100) at 80 mL min−1 to afford fifteen fractions, VA1–VA15, according to their TLC profiles. Fractions VA4–VA6 were gathered (14.7 g) and were subjected to a second flash chromatography using a silica 120 g Grace cartridge with a gradient of CH2Cl2–MeOH (100
:
0 to 0
:
100) at 80 mL min−1 to afford thirteen fractions VAB1–VAB13. Among them, VAB6 (1.7 g) was selected for further fractionation and submitted to Sephadex LH20 and eluted with a mixture of MeOH
:
CH2Cl2 (8
:
2) to afford fifteen fractions VAB61–VAB615. VAB67 (761 mg) was submitted again to Sephadex LH-20 (4 cm × 90 cm) and eluted with a mixture of MeOH
:
CH2Cl2 (8
:
2) to afford thirteen fractions VAB671–VAB6713. VAB6713 underwent a preparative HPLC separation using a gradient of MeOH–H2O with 0.1% formic acid (grad 10–80% in 25 min) to give compound 1 (3.2 mg, yield 0.0005%).
Physical and spectroscopic data of 1
Pyrrovobasine (1).
Brown amorphous solid; [α]25.0D + 160 (c 0.05, MeOH); IR νmax 3400, 1720 cm−1; UV (MeOH) λmax (log
ε) 232 (2.42), 296.1 (3.3) nm; 1H and 13C NMR data, see Table 1; HR-ESI-MS m/z 605.3122 [M + H]+ (calcd for C37H41N4O4, 605.3122); MS/MS spectrum was deposited in the GNPS spectral library under the identifier: CCMSLIB00006710432.
Table 1
1H and 13C NMR Data for 1 (CDCl3, 600/150 MHz)
No. |
δ
H, mult (J, Hz) |
δ
C
|
No. |
δ
H, mult (J, Hz) |
δ
C
|
1 (NH) |
8.24, br s |
|
1′ (NH) |
8.30, br s |
|
2 |
|
135.8 |
|
|
|
3 |
4.87, dd (13.4, 2.7) |
35.8 |
2′ |
|
137.9 |
5 |
4.42, t (8.5) |
60.7 |
3′ |
|
107.6 |
6 |
3.63, m |
21.5 |
4′ |
7.72, m |
118.9 |
|
3.74, m |
|
|
|
|
|
|
|
5′ |
7.08, ov. |
120.0 |
7 |
|
106.8 |
|
|
|
8 |
|
129.1 |
6′ |
7.08, ov. |
122.9 |
9 |
7.55, d (7.7) |
117.7 |
|
|
|
10 |
7.11, ov. |
120.0 |
7′ |
7.16, ov. |
110.8 |
11 |
7.15, ov. |
122.9 |
8′ |
|
128.4 |
12 |
7.16, ov. |
110.8 |
9′ |
|
135.8 |
13 |
|
136.4 |
α |
3.42, m |
26.7 |
14 |
2.02, ddd (15.0, 6.7, 3.1) |
35.2 |
β |
4.67, ov. |
46.9 |
|
2.75, q (13.4) |
|
|
4.84, dd (13.7, 6.7) |
|
15 |
3.89, m |
32.5 |
2′′ |
|
132.9 |
16 |
2.99, br s |
43.8 |
3′′ |
6.97, d (3.9) |
124.3 |
|
|
|
4′′ |
6.30, d (3.9) |
110.7 |
18 |
1.73, d (6.9) |
13.0 |
5′′ |
|
141.9 |
|
|
|
6′′ |
4.63, d (13.5) |
56.7 |
19 |
5.60, q (6.9) |
126.6 |
|
4.67, d (13.5) |
|
|
|
|
7′′ |
9.56, s |
179.9 |
20 |
|
132.9 |
|
|
|
21 |
4.23, d (13.7) |
51.4 |
|
|
|
|
3.45, ov. |
|
|
|
|
OOMe |
|
169.2 |
|
|
|
COO![[M with combining low line]](https://www.rsc.org/images/entities/char_004d_0332.gif) ![[e with combining low line]](https://www.rsc.org/images/entities/char_0065_0332.gif) |
2.49, s |
51.0 |
|
|
|
N-Me |
2.89, s |
40.5 |
|
|
|
Data dependent LC-HRMS2 analyses
LC-ESI-HRMS2 analyses were achieved by coupling the LC system to a hybrid quadrupole time of-flight mass spectrometer Agilent 6530 (Agilent Technologies, Massy, France) equipped with an ESI† source, operating in positive-ion mode. Source parameters were set as follows: capillary temperature at 320 °C, source voltage at 3500 V, sheath gas flow rate at 10 L min−1. The divert valve was set to waste for the first 3 min. MS scans were operated in full-scan mode from m/z 100 to 1700 (0.1 s scan time) with a mass resolution of 11
000 at m/z 922. MS1 scan was followed by MS2 scans of the three most intense ions above an absolute threshold of 5000 counts. Selected parent ions were fragmented with on collision energie fixed at 50 eV and an isolation window of 1.3 amu. Calibration solution, containing two internal reference masses (purine, C5H4N4, m/z 121.050873, and HP-921 [hexakis-(1H,1H,3H-tetrafluoropentoxy)phosphazene], C18H18O6N3P3F24, m/z 922.0098). A permanent MS/MS exclusion list criterion was set to prevent oversampling of the internal calibrants. LC-UV and MS data acquisition and processing were performed using MassHunter Workstation software (Agilent Technologies, Massy, France).
Molecular networking
The MS2 data files related to the alkaloid extract of the barks of V. africana were converted from the .d (Agilent) standard data-format to .mzXML format using the MSConvert software, part of the ProteoWizard package.39 A molecular network was created using the online Molecular Networking workflow (version release_8) at GNPS17 (http://gnps.ucsd.edu) with a parent mass tolerance of 0.02 Da and a MS/MS fragment ion tolerance of 0.02 Da. A network was then created where edges were filtered to have a cosine score above 0.65 and more than 6 matched peaks. Further edges between two nodes were kept in the network if and only if each of the nodes appeared in each other's respective top 10 most similar nodes. The spectra in the network were then searched against GNPS spectral libraries. All matches kept between network spectra and library spectra were required to have a score above 0.6 and at least 6 matched peaks. The molecular networking data were analyzed and visualized using Cytoscape (ver. 3.6.0).40
Computational methods
The lowest energy conformer of compound 1 was fully optimized in vacuo and without constraint using DFT41,42 with the hybrid Becke-3-parameter-Lee–Yang–Parr43,44 exchange– correlation functional and the 6-31G* basis,45 as implemented in the Gaussian 16 revB.01 software package.46 Upon geometrical optimization convergence, a frequency calculation within the harmonic approximation was conducted at the same level of theory and conformer was characterized as a minimum by the absence of imaginary frequency. TDDFT at the same level of theory was then employed to predict energies as well as rotational strengths of the first 60 electronic transitions. The UCSF Chimera v1.1147 software package was used for the depiction of the most stable conformer of compound 1. ECD spectrum envelope was then calculated using SpecDis v1.7148 using sigma/gamma value of 0.2 eV and a shift of +14 nm and rendered using Gnuplot v5.2.49
Biological evaluation
Antibacterial activity assay by microdilutions.
The antibacterial activity was assessed as previously described50 against Staphylococcus aureus (ATCC 6538), Pseudomonas aeruginosa (ATCC 15442), Mycobacterium smegmatis (CIP 7326) and Mycobactrium abscessus (M. abscessus subsp. bolletii, ITM-M001006, BCCM, Belgium). The assays were performed in 96-well plates using a broth microdilution method in order to identify the minimal inhibitory concentration (MIC). Two-fold serial dilutions (100 μL in 200 μL final volume) of the molecules were first performed in triplicates in Mueller Hinton broth (or in the 7H9 medium when working with M. abscessus). Then, 100 μL bacteria (100 fold dilution of 0.5 Mc Farland) were added into each well. Negative (without bacteria) and positive (without additional compounds) controls were present in each plate. The MIC value were first interpreted after 24 h incubation at 37 °C (or 2–3 days incubation for M. abscessus), corresponding to the lowest concentration inhibiting bacteria growth after visual inspection. This visual interpretation was always verified by adding MTT reagent, 3-(4,5-dimethylthiazol-2-yl)-2,5-diphenyltetrazolium bromide, (0.5 mg mL−1 final concentration) to allow formazan crystal formation in viable cells during 4 h additional incubation period. A final naked eye inspection was performed to record the results. This assay was performed in triplicate in three separate experiments. Positive control drugs were vancomycin hydrochloride for Staphylococcus aureus, cetrimide for Pseudomonas aeruginosa, and rifampicin for Mycobacterium smegmatis.
Activity assay against M. bovis BCG.
To assess the antimycobacterial activity of our compounds on M. bovis BCG, the MIC was assessed by the microdilution method as previously described,51 performed in 7H9 medium supplemented with 10% albumin-dextrose complex and 0.2% glycerol in a polycarbonate tube to reach a final volume of 1 ml. Briefly, 0.5 mL of 2-fold serial drug dilutions were inoculated with 0.5 mL (at 5 × 105 CFU per mL) early exponential culture. Vancomycin at a final concentration of 25 μg mL−1 (far under its MIC > 500 mg mL−1) was eventually added to investigate the possible synergy between the two drugs. The MIC was interpreted as the lowest drug concentration that inhibited visible bacterial growth, defined by clump formation, when growth of the 1% inoculum drug-free control became visible (after 5–10 days growth).
The antimycobacterial activity was further assessed to investigate whether at the MIC, compounds could have an irreversible antimycobacterial activity (bactericidal activity) or a reversible effect (bacteriostatic activity) on M. bovis BCG. This was assessed by spreading 10 μL out of the 1 mL tubes from the broth microdilution method on 7H11 agar plate. Proliferation of bacteria colonies was interpreted as bacteria having encountered mycobacteriostatic compounds at their MIC, while absence of proliferation was interpreted as bacteria having encountered mycobactericidal compounds at their MBC (minimal bactericidal concentration).
Author contributions
The manuscript was written through contributions of all authors. All authors have given approval to the final version of the manuscript.
Conflicts of interest
There are no conflicts to declare.
Acknowledgements
We are very grateful to OPCW (Organisation for the Prohibition of Chemical Weapons) for the postdoctoral fellowship of H. F. and the financial support from ARES-CCD (Belgium). In addition, this work was supported by the French ANR grant ANR-20-CE43-0010. Leo Goehrs (Alionis) is gratefully acknowledged for the donation of the computing hardware.
Notes and references
- Q. Zhao, W.-T. Zhu, X. Ding, Z.-Q. Huo, P. O. Donkor, T. A. Adelakun, X.-J. Hao and Y. Zhang, Voacafrines A-N, aspidosperma-type monoterpenoid indole alkaloids from Voacanga africana with AChE inhibitory activity, Phytochemistry, 2021, 181, 112566 CrossRef CAS PubMed.
- M. Harada, K. N. Asaba, M. Iwai, N. Kogure, M. Kitajima and H. Takayama, Asymmetric Total Synthesis of an Iboga-Type Indole Alkaloid, Voacangalactone, Newly Isolated from Voacanga africana, Org. Lett., 2012, 14, 5800–5803 CrossRef CAS PubMed.
- C.-F. Ding, H.-X. Ma, J. Yang, X.-J. Qin, G. S. S. Njateng, H.-F. Yu, X. Wei, Y.-P. Liu, W.-Y. Huang, Z.-F. Yang, X.-H. Wang and X.-D. Luo, Antibacterial Indole Alkaloids with Complex Heterocycles from Voacanga africana, Org. Lett., 2018, 20, 2702–2706 CrossRef CAS PubMed.
- M. A. Beniddir, K. B. Kang, G. Genta-Jouve, F. Huber, S. Rogers and J. J. J. van der Hooft, , Advances in decomposing complex metabolite mixtures using substructure- and network-based computational metabolomics approaches, Nat. Prod. Rep., 2021 10.1039/D1NP00023C.
- A. E. Fox Ramos, L. Evanno, E. Poupon, P. Champy and M. A. Beniddir, Natural products targeting strategies involving molecular networking: different manners, one goal, Nat. Prod. Rep., 2019, 36, 960–980 RSC.
- E. Otogo N'Nang, P. Le Pogam, T. Ndong Mba, C. Sima Obiang, E. Mouray, P. Grellier, B. Kumulungui, P. Champy and M. A. Beniddir, Targeted Isolation of Hemitheion from Mostuea brunonis, a Proposed Biosynthetic Intermediate of Theionbrunonines, J. Nat. Prod., 2021, 84, 1409–1413 CrossRef.
- T. Kouamé, G. Bernadat, V. Turpin, M. Litaudon, A. T. Okpekon, J.-F. Gallard, K. Leblanc, S. Rharrabti, P. Champy, E. Poupon, M. A. Beniddir and P. Le Pogam, Structure Reassignment of Melonine and Quantum-Chemical Calculations-Based Assessment of Biosynthetic Scenarios Leading to Its Revised and Original Structures, Org. Lett., 2021, 23, 5964–5968 CrossRef.
- C. F. Alcover, G. Bernadat, F. A. Kabran, P. Le Pogam, K. Leblanc, A. E. Fox Ramos, J.-F. Gallard, E. Mouray, P. Grellier, E. Poupon and M. A. Beniddir, Molecular Networking Reveals Serpentinine-Related Bisindole Alkaloids from Picralima nitida, a Previously Well-Investigated Species, J. Nat. Prod., 2020, 83, 1207–1216 CrossRef CAS.
- T. Kouamé, A. T. Okpekon, N. F. Bony, A. D. N'Tamon, J.-F. Gallard, S. Rharrabti, K. Leblanc, E. Mouray, P. Grellier, P. Champy, M. A. Beniddir and P. Le Pogam, Corynanthean-Epicatechin Flavoalkaloids from Corynanthe pachyceras, Molecules, 2020, 25, 2654 CrossRef.
- G. Cauchie, E. O. N'Nang, J. J. J. van der Hooft, P. Le Pogam, G. Bernadat, J.-F. Gallard, B. Kumulungui, P. Champy, E. Poupon and M. A. Beniddir, Phenylpropane as an Alternative Dearomatizing Unit of Indoles: Discovery of Inaequalisines A and B Using Substructure-Informed Molecular Networking, Org. Lett., 2020, 22, 6077–6081 CrossRef CAS.
- A. D. N'Tamon, A. T. Okpekon, N. F. Bony, G. Bernadat, J.-F. Gallard, T. Kouamé, B. Séon-Méniel, K. Leblanc, S. Rharrabti, E. Mouray, P. Grellier, M. Ake, N. C. C. Amin, P. Champy, M. A. Beniddir and P. Le Pogam, Streamlined targeting of Amaryllidaceae alkaloids
from the bulbs of Crinum scillifolium using spectrometric and taxonomically-informed scoring metabolite annotations, Phytochemistry, 2020, 179, 112485 CrossRef PubMed.
- A. E. Fox Ramos, C. Pavesi, M. Litaudon, V. Dumontet, E. Poupon, P. Champy, G. Genta-Jouve and M. A. Beniddir, CANPA: Computer-Assisted Natural Products Anticipation, Anal. Chem., 2019, 91, 11247–11252 CrossRef CAS PubMed.
- A. E. Fox Ramos, P. Le Pogam, C. Fox Alcover, E. Otogo N'Nang, G. Cauchie, H. Hazni, K. Awang, D. Bréard, A. M. Echavarren, M. Frédérich, T. Gaslonde, M. Girardot, R. Grougnet, M. S. Kirillova, M. Kritsanida, C. Lémus, A.-M. Le Ray, G. Lewin, M. Litaudon, L. Mambu, S. Michel, F. M. Miloserdov, M. E. Muratore, P. Richomme-Peniguel, F. Roussi, L. Evanno, E. Poupon, P. Champy and M. A. Beniddir, Collected mass spectrometry data on monoterpene indole alkaloids from natural product chemistry research, Sci. Data, 2019, 6, 15 CrossRef.
- G. Baudouin, F. Tillequin, M. Bert and M. Koch, Ceridimine: a novel type of bisindole monoterpene alkaloid, J. Chem. Soc., Chem. Commun., 1986, 3–4 RSC.
-
T.-S. Kam and Y.-M. Choo, in The Alkaloids: Chemistry and Biology, ed. G. A. Cordell, Academic Press, 2006, vol. 63, pp. 181–337 Search PubMed.
-
M. Kitajima and H. Takayama, in The Alkaloids: Chemistry and Biology, ed. H.-J. Knölker, Academic Press, 2016, vol. 76, pp. 259–310 Search PubMed.
- M. Wang, J. J. Carver, V. V. Phelan, L. M. Sanchez, N. Garg, Y. Peng, D. D. Nguyen, J. Watrous, C. A. Kapono, T. Luzzatto-Knaan, C. Porto, A. Bouslimani, A. V. Melnik, M. J. Meehan, W.-T. Liu, M. Crusemann, P. D. Boudreau, E. Esquenazi, M. Sandoval-Calderon, R. D. Kersten, L. A. Pace, R. A. Quinn, K. R. Duncan, C.-C. Hsu, D. J. Floros, R. G. Gavilan, K. Kleigrewe, T. Northen, R. J. Dutton, D. Parrot, E. E. Carlson, B. Aigle, C. F. Michelsen, L. Jelsbak, C. Sohlenkamp, P. Pevzner, A. Edlund, J. McLean, J. Piel, B. T. Murphy, L. Gerwick, C.-C. Liaw, Y.-L. Yang, H.-U. Humpf, M. Maansson, R. A. Keyzers, A. C. Sims, A. R. Johnson, A. M. Sidebottom, B. E. Sedio, A. Klitgaard, C. B. Larson, C. A. Boya P, D. Torres-Mendoza, D. J. Gonzalez, D. B. Silva, L. M. Marques, D. P. Demarque, E. Pociute, E. C. O'Neill, E. Briand, E. J. N. Helfrich, E. A. Granatosky, E. Glukhov, F. Ryffel, H. Houson, H. Mohimani, J. J. Kharbush, Y. Zeng, J. A. Vorholt, K. L. Kurita, P. Charusanti, K. L. McPhail, K. F. Nielsen, L. Vuong, M. Elfeki, M. F. Traxler, N. Engene, N. Koyama, O. B. Vining, R. Baric, R. R. Silva, S. J. Mascuch, S. Tomasi, S. Jenkins, V. Macherla, T. Hoffman, V. Agarwal, P. G. Williams, J. Dai, R. Neupane, J. Gurr, A. M. C. Rodriguez, A. Lamsa, C. Zhang, K. Dorrestein, B. M. Duggan, J. Almaliti, P.-M. Allard, P. Phapale, L.-F. Nothias, T. Alexandrov, M. Litaudon, J.-L. Wolfender, J. E. Kyle, T. O. Metz, T. Peryea, D.-T. Nguyen, D. VanLeer, P. Shinn, A. Jadhav, R. Muller, K. M. Waters, W. Shi, X. Liu, L. Zhang, R. Knight, P. R. Jensen, B. O. Palsson, K. Pogliano, R. G. Linington, M. Gutierrez, N. P. Lopes, W. H. Gerwick, B. S. Moore, P. C. Dorrestein and N. Bandeira, Sharing and community curation of mass spectrometry data with Global Natural Products Social Molecular Networking, Nat. Biotechnol., 2016, 34, 828–837 CrossRef CAS.
-
DNP
http://dnp.chemnetbase.com/
(accessed Aug 27, 2021).
- H. Takayama, S. Suda, I.-S. Chen, M. Kitajima, N. Aimi and S.-i. Sakai, Two New Dimeric Indole Alkaloids from Tabernaemontana subglobosa Merr. from Taiwan, Chem. Pharm. Bull., 1994, 42, 280–284 CrossRef CAS.
- M. Azoug, A. Loukaci, B. Richard, J.-M. Nuzillard, C. Moreti, M. Zèches-Hanrot and L. Le Men-Olivier, Alkaloids from stem bark and leaves of Peschiera buchtieni, Phytochemistry, 1995, 39, 1223–1228 CrossRef CAS.
- S. Subhadhirasakul, H. Takayama, Y. Miyabe, M. Kitajima, D. Ponglux, S.-i. Sakai and N. Aimi, Two novel sarpagine-type indole alkaloids from the leaves of Hunteria zeylanica in Thailand, Heterocycles, 1995, 9, 2049–2056 Search PubMed.
- T.-S. Kam and K.-M. Sim, Conodurine, conoduramine, and ervahanine derivatives from Tabernaemontana corymbosa, Phytochemistry, 2003, 63, 625–629 CrossRef CAS PubMed.
- C. Zhang, Y. Idelbayev, N. Roberts, Y. Tao, Y. Nannapaneni, B. M. Duggan, J. Min, E. C. Lin, E. C. Gerwick, G. W. Cottrell and W. H. Gerwick, Small Molecule Accurate Recognition Technology (SMART) to Enhance Natural Products Research, Sci. Rep., 2017, 7, 14243 CrossRef PubMed.
- R. Reher, H. W. Kim, C. Zhang, H. H. Mao, M. Wang, L.-F. Nothias, A. M. Caraballo-Rodriguez, E. Glukhov, B. Teke, T. Leao, K. L. Alexander, B. M. Duggan, E. L. Van Everbroeck, P. C. Dorrestein, G. W. Cottrell and W. H. Gerwick, A Convolutional Neural Network-Based Approach for the Rapid Annotation of Molecularly Diverse Natural Products, J. Am. Chem. Soc., 2020, 142, 4114–4120 CrossRef CAS PubMed.
- T. M. Zennie, J. M. Cassady and R. F. Raffauf, Funebral, a New Pyrrole Lactone Alkaloid from Quararibea funebris, J. Nat. Prod., 1986, 49, 695–698 CrossRef CAS.
- N. Harada and K. Nakanishi, Exciton chirality method and its application to configurational and conformational studies of natural products, Acc. Chem. Res., 1972, 5, 257–263 CrossRef CAS.
- A. E. Nugroho, Y. Hirasawa, N. Kawahara, Y. Goda, K. Awang, A. H. A. Hadi and H. Morita, Bisnicalaterine A, a Vobasine−Vobasine Bisindole Alkaloid from Hunteria zeylanica, J. Nat. Prod., 2009, 72, 1502–1506 CrossRef CAS.
- J. Xu, Y. C. Song, Y. Guo, Y. N. Mei and R. X. Tan, Fumigaclavines D–H, New Ergot Alkaloids from Endophytic Aspergillus fumigatus, Planta Med., 2014, 80, 1131–1137 CrossRef CAS.
- C. K. Narkowicz, A. J. Blackman, E. Lacey, J. H. Gill and K. Heiland, Convolutindole A and Convolutamine H, New Nematocidal Brominated Alkaloids from the Marine Bryozoan Amathia convoluta, J. Nat. Prod., 2002, 65, 938–941 CrossRef CAS.
- L. S. Mulwa, R. Jansen, D. F. Praditya, K. I. Mohr, J. Wink, E. Steinmann and M. Stadler, Six Heterocyclic Metabolites from the Myxobacterium Labilithrix luteola, Molecules, 2018, 23, 542 CrossRef.
- T. A. Foderaro, L. R. Barrows, P. Lassota and C. M. Ireland, Bengacarboline, a New β-Carboline from a Marine Ascidian Didemnum sp., J. Chem. Org., 1997, 62, 6064–6065 CrossRef CAS.
- A. M. Wolters, D. A. Jayawickrama and J. V. Sweedler, Comparative Analysis of a Neurotoxin from Calliostoma canaliculatum by On-Line Capillary Isotachophoresis/H NMR and Diffusion 1H NMR, J. Nat. Prod., 2005, 68, 162–167 CrossRef CAS PubMed.
- C. T. Walsh, Biological Matching of Chemical Reactivity: Pairing Indole Nucleophilicity with Electrophilic Isoprenoids, ACS Chem. Biol., 2014, 9, 2718–2728 CrossRef CAS.
- M. E. Tanner, Mechanistic studies on the indole prenyltransferases, Nat. Prod. Rep., 2015, 32, 88–101 RSC.
- J. M. Wood, D. P. Furkert and M. A. Brimble, 2-Formylpyrrole natural products: origin, structural diversity, bioactivity and synthesis, Nat. Prod. Rep., 2019, 36, 289–306 RSC.
- M. Hellwig and T. Henle, Baking, Ageing, Diabetes: A Short History of the Maillard Reaction, Angew. Chem., Int. Ed., 2014, 53, 10316–10329 CrossRef CAS.
- W.-G. Shan, Y. Wang, L.-F. Ma and Z.-J. Zhan, A new pyrrole alkaloid from the mycelium of Inonotus obliquus, J. Chem. Res., 2017, 41, 392–393 CrossRef CAS.
- Z. Sun, M. Hu, Z. Sun, N. Zhu, J. Yang, G. Ma and X. Xu, Pyrrole Alkaloids from the Edible Mushroom Phlebopus portentosus with Their Bioactive Activities, Molecules, 2018, 23, 1198 CrossRef.
- M. C. Chambers, B. Maclean, R. Burke, D. Amodei, D. L. Ruderman, S. Neumann, L. Gatto, B. Fischer, B. Pratt, J. Egertson, K. Hoff, D. Kessner, N. Tasman, N. Shulman, B. Frewen, T. A. Baker, M.-Y. Brusniak, C. Paulse, D. Creasy, L. Flashner, K. Kani, C. Moulding, S. L. Seymour, L. M. Nuwaysir, B. Lefebvre, F. Kuhlmann, J. Roark, P. Rainer, S. Detlev, T. Hemenway, A. Huhmer, J. Langridge, B. Connolly, T. Chadick, K. Holly, J. Eckels, E. W. Deutsch, R. L. Moritz, J. E. Katz, D. B. Agus, M. MacCoss, D. L. Tabb and P. Mallick, A cross-platform toolkit for mass spectrometry and proteomics, Nat. Biotechnol., 2012, 30, 918–920 CrossRef CAS.
- P. Shannon, A. Markiel, O. Ozier, N. S. Baliga, J. T. Wang, D. Ramage, N. Amin, B. Schwikowski and T. Ideker, Cytoscape: A Software Environment for Integrated Models of Biomolecular Interaction Networks, Genome Res., 2003, 13, 2498–2504 CrossRef CAS.
- P. Hohenberg and W. Kohn, Inhomogeneous Electron Gas, Phys. Rev., 1964, 136, B864–B871 CrossRef.
- W. Kohn and L. J. Sham, Self-Consistent Equations Including Exchange and Correlation Effects, Phys. Rev. [Sect.] B, 1965, 140, A1133–A1138 CrossRef.
- A. D. Becke, Density–functional thermochemistry. III. The role of exact exchange, J. Chem. Phys., 1993, 98, 5648–5652 CrossRef CAS.
- C. Lee, W. Yang and R. G. Parr, Development of the Colle-Salvetti correlation-energy formula into a functional of the electron density, Phys. Rev. B: Condens. Matter Mater. Phys., 1988, 37, 785–789 CrossRef CAS.
-
W. J. R. Hehre, L. Radom, P. V. R. Schleyer and J. A. Pople, AB Initio Molecular Orbital Theory, Wiley, New York, 1986 Search PubMed.
-
M. J. Frisch, H. B. Trucks, G. W. Schlegel, G. E. Scuseria, M. A. Robb, J. R. Cheeseman, G. Scalmani, V. Barone, G. A. Petersson and H. Nakatsuji, et al. Gaussian 16 Revision B.01, Gaussian Inc., Wallingford, CT, USA, 2016 Search PubMed.
- E. F. Pettersen, T. D. Goddard, C. C. Huang, G. S. Couch, D. M. Greenblatt, E. C. Meng and T. E. Ferrin, UCSF Chimera—A visualization system for exploratory research and analysis, J. Comput. Chem., 2004, 25, 1605–1612 CrossRef CAS PubMed.
- T. Bruhn, A. Schaumlöffel, Y. Hemberger and G. Bringmann, SpecDis: Quantifying the Comparison of Calculated and Experimental Electronic Circular Dichroism Spectra, Chirality, 2013, 25, 243–249 CrossRef CAS.
-
H. B. Bröker, J. Campbell, R. Cunningham, D. Denholm, G. Elber, R. Fearick, C. Grammes, L. Hart, L. Heckingu and P. Juhász, Gnuplot 4.6: an Interactive Plotting Program, http://gnuplot.sourceforge.net/ Search PubMed.
- S. Oliveira Ribeiro, V. Fontaine, V. Mathieu, A. Zhiri, D. Baudoux, C. Stévigny and F. Souard, Antibacterial and Cytotoxic Activities of Ten Commercially Available Essential Oils, Antibiotics, 2020, 9, 717 CrossRef.
- C. Rens, F. Laval, M. Daffé, O. Denis, R. Frita, A. Baulard, R. Wattiez, P. Lefèvre and V. Fontaine, Effects of Lipid-Lowering Drugs on Vancomycin Susceptibility of Mycobacteria, Antimicrob. Agents Chemother., 2016, 60, 6193–6199 CrossRef CAS.
Footnote |
† Electronic supplementary information (ESI) available: Cartesian coordinates, HRMS, and NMR data of 1. See DOI: 10.1039/d1ob01791h |
|
This journal is © The Royal Society of Chemistry 2022 |