DOI:
10.1039/D1NJ04934H
(Paper)
New J. Chem., 2022,
46, 419-425
Dibenzo[f,h]furo[2,3-b]quinoxaline-based molecular scaffolds as deep blue fluorescence materials for organic light-emitting diodes†
Received
16th October 2021
, Accepted 21st November 2021
First published on 22nd November 2021
Abstract
A novel molecular scaffold, dibenzo[f,h]furo[2,3-b]quinoxaline (diBFQ), and its derivatives, 3,6-bis(4-isopropylphenyl)-11-phenyldibenzo[f,h]furo[2,3-b]quinoxaline (dP-diBFQ) and furo[2′,3′:5,6]pyrazino[2,3-f][1,10]phenanthroline (FPPhen), were designed and synthesized, showing highly efficient pure and deep blue emission. The unsubstituted molecular scaffolds diBFQ and FPPhen exhibit emission from 424–445 nm with PLQYs of ∼70%, and the disubstituted derivative of diBFQ, dP-diBFQ, shows a PLQY of 79.1%. Our experimental and theoretical investigations revealed that the additional N atoms in FPPhen facilitate the electron distribution of the HOMO orbital localizing on a smaller π-conjugate fragment compared with diBFQ, which resulted in the emission peak blue-shifting from 445 nm for diBFQ to 424 nm for FPPhen. Furthermore, introducing the steric substituent isopropylphenyl into the diBFQ scaffold would help to suppress aggregates of dP-diBFQ molecules and improve the fluorescence efficiency. OLEDs using dP-diBFQ as dopants show pure blue emission with CIE coordinates of (0.154, 0.102) and an EQE of 2.28%. Upon increasing the dopant concentration, the EQE and current efficiency could be improved to 2.63% and 4.7 cd A−1, but aggregate emission with a peak from 472–476 nm was clearly observed due to molecular aggregation. Our studies clearly suggested that diBFQ and FPPhen scaffolds are promising fluorescence cores for deep and pure-blue emission.
1. Introduction
In the past three decades, organic light-emitting diodes (OLEDs) have achieved great progress and been widely applied to varied commercial displays, such as cell phone, digital camera, vehicle display and so on. However, scarcity of pure-blue emitters featured with a high efficiency and stability limits the extensive application of OLEDs to larger-area display fields, such as notebooks, computer screens, TVs and so on. The research for such emitters is highly challenging. The blue phosphorescent emitters suffered the drawbacks of an inferior color purity, severe efficiency roll-off and short lifetime in devices, although the phosphorescence emitters1–6 can achieve 100% internal quantum efficiency (IQE). As a result, only green and red phosphorescent emitters are applied to commercial displays while blue emission is provided by conventional fluorescence dopants (FDs). Recently developed TADF (thermally active delayed fluorescence) emitters7–10 emerged as promising alternatives to phosphorescent emitters owing to the same 100% IQE. Unfortunately, serious efficiency roll-off at high luminance results in a short device lifetime and loss of energy. Especially for blue TADF emitters, this kind of phenomenon is more serious.11 To overcome these defects, the strategy of TADF-sensitized fluorescent (TSF) OLEDs12–15 was proposed by using materials with TADF as sensitizers and FDs as the final emitters, offering the possibility of a low efficiency roll-off, high color-purity, and long term-stabilities with all pure organic compounds. So, the TSF strategy has been considered as one of the most promising technologies for next-generation OLEDs. However, the performances of TSF-OLEDs highly depend on the interplay of the components in EML. In this sense, developing efficient blue FDs to match the TADF sensitizer or host is essential. On the other hand, for commercial displays, it is highly expected new molecule scaffolds for blue emission could be achieved to the improve efficiency and stability of pure blue fluorescence OLEDs.
In recent work, we have reported that the 2-phenylfuro[2,3-b]quinoxaline (FQ) scaffold could provide deep blue emission with comparable efficiency to that of anthracene-based derivatives, and found substituting at the 7-position of FQ had more perturbation of the electronic properties of the FQ than that at the 6-position of FQ. Furthermore, we found that the compound (BFQ) formed by using the 6 and 7-positions of FQ fused with a benzene ring showed green emission.16 Our previous works clearly revealed that the 5,6-position of the FQ scaffold exhibits a significantly different electron effect from that of the 7,8-position of FQ. In this manuscript, we incorporated two benzene rings or two pyridine rings to the 5,6-position and 7,8-position of the FQ scaffold by a fusing strategy to produce two new molecular scaffolds for efficient pure and deep blue emission, dibenzo[f,h]furo[2,3-b]quinoxaline (diBFQ) and furo[2′,3′:5,6]pyrazino[2,3-f][1,10]phenanthroline (FPPhen). Furthermore, the steric substituent, 4-isopropylphenyl, was introduced into the 3 and 6-positions of diBFQ to produce a highly efficient pure blue emitter, 3,6-bis(4-isopropylphenyls)-11-phenyldibenzo[f,h]furo[2,3-b]quinoxaline (dP-diBFQ), for application to OLEDs. The effects of the substituent, fusing position and N atoms on the photophysical properties of the resulting compounds were investigated. Both molecule scaffolds present deep and pure blue emission with about a ∼70% absolute photoluminescence quantum yield (PLQY) in solution, and the diBFQ gives a PL peak of 445 nm while the additional N atoms in FPPhen facilitate a hypsochromic shift in emission to 424 nm. The disubstituted compound, dP-diBFQ, gives a 79.1% PLQY, and using it as a dopant, the OLEDs shows a pure blue emission with an EL peak of 444 nm, CIE coordinates (0.154, 0.102) and a 2.28% external quantum efficiency (EQE). The high efficiency and stability of the device indicates that diBFQ is a promising fluorescence molecule scaffold for deep and pure-blue OLEDs.
2. Results and discussion
2.1. Synthesis
The diBFQ, FPPhen and dP-diBFQ were synthesized following our reported route,16 as shown in Scheme S1. According to our previously reported procedures, the 5-phenyl-2,3-dihydrofuran-2,3-dione firstly reacted with types of aryldiamine in acetic acid to generate the intermediates, 2a–c, which were not separated, and cyclization reaction was directly performed in polyphosphoric acid to afford the compounds diBFQ, FPPhen, and intermediate 3c. The 3c was further reacted with isopropylphenylboronic acid via a Suzuki coupling reaction to produce the final compound dP-diBFQ.
2.2. Photophysical properties
Fig. 1 shows the ultraviolet-visible absorption and PL fluorescence spectra of diBFQ, FPPhen and dP-diBFQ in a toluene solution, and their photophysical data are listed in Table 1. The diBFQ shows absorption bands with a pronounced vibronic splitting, and the dominant absorption peak appearing at 412 nm is ascribed to the S0 → S1 (HOMO → LUMO) transition according to the calculated results. The FPPhen exhibits a similar absorption behavior to that of diBFQ, but its HOMO → LUMO transition is shifted to 396 nm, about a 15 nm blue-shift. The disubstituted compound of diBFQ with two isopropylphenyls, dP-diBFQ, shows a significantly broadened and red-shifted absorption as compared with that of the unsubstituted diBFQ, obviously implying a produced conjugation between the isopropylphenyl and diBFQ. The optical gaps were identified to be 2.95.0, 3.05 and 2.84 eV for diBFQ, FPPhen and dP-diBFQ from violet-visible absorption spectra in toluene solution.
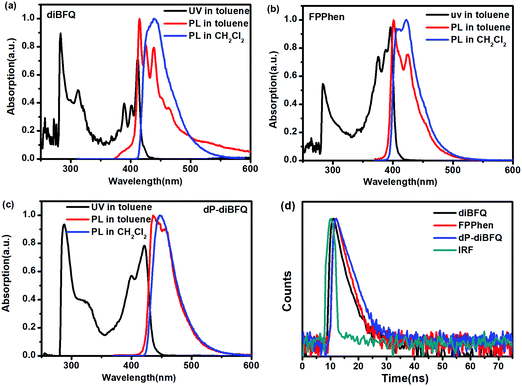 |
| Fig. 1 The UV and PL spectra in toluene and CH2Cl2 solution for diBFQ (a), FPPhen (b), and dP-diBFQ (c), and the transient PL spectra in toluene solution (d). | |
Table 1 The photophysical properties of diBFQ, FPPhen, dP-diBFQ, 26dPFQ, 27dPFQ, and BFQ
Compound |
λ
abs [nm] |
λ
em [nm] |
PLQY [%] |
t
p [ns] |
HOMO [eV] |
LUMO [eV] |
Measured in CH2Cl2 solution (10−5 M).
|
diBFQ, |
312, 389, 400, 412 |
445a |
72.0 |
1.05 |
−5.95 |
−3.01 |
FPPhen |
375, 388, 396 |
424a |
70.0 |
1.26 |
−6.09 |
−2.95 |
dP-diBFQ |
287, 399, 421 |
457a |
79.1 |
1.76 |
−6.07 |
−3.23 |
26dPFQ |
283, 387 |
422 |
58.9 |
3.07 |
−5.96 |
−3.08 |
27dPFQ |
275, 382 |
422 |
48.7 |
1.80 |
−5.88 |
−2.97 |
BFQ |
313, 280, 396 |
515 |
9.2 |
3.58 |
−5.57 |
−3.01 |
Under photoexcitation, the three compounds show clear vibronic splitting peaks in dilute toluene solutions. The significant vibronic splitting of the emission peaks suggests they own a rigid molecular structure. The diBFQ shows triple splitting peaks with values of 414, 425 and 439 nm, respectively, and a peak width at half-height (FWHM) of 36 nm. The calculated LUMO → HOMO (S1) emission energy for diBFQ is 430.4 nm, and thus, the peak of 439 nm can be ascribed to S1 emission. The FPPhen displays two pronounced splitting peaks with blueshift values of 400 and 424 nm, respectively, and a FWHM of 40 nm, and the 424 nm emission should be from an S1 emission due to the calculated S1 energy of 420.5 nm. This indicates that additional N atoms in FPPhen would be beneficial to promote a hypsochromic shift of both the absorption and emission. The theoretical calculations showed that the additional N atoms in FPPhen made the electron distribution of the HOMO orbital tend toward localization, resulting in a shortening of the π-conjugate skeleton, and consequently a blue-shift of emission. For dP-diBFQ, a main peak of 436 nm accompanying two weak shoulder peaks of 447 and 457 nm were observed. Switching to a CH2Cl2 solution, the pronounced vibronic splitting of the emission peaks disappears and only one main peak was observed, 424 nm, 445 nm and 457 nm for diBFQ, FPPhen and dP-diBFQ, respectively.
Noteworthily, our previously reported compounds, 26dPFQ and 27dPFQ with a scaffold of diphenylfuro[2,3-b]quinoxaline, emit deep blue light with a fluorescence peak of 422 nm.16 While when one benzene ring was fused at the 6 and 7-positions of 2-phenylfuro[2,3-b]quinoxaline, the resulting compound, BFQ, shows a green emission with a fluorescence peak of 515 nm.17 The UV and PL spectra of 26dPFQ, 27dPFQ, and BFQ are provided in Fig. S1 (ESI†). Here, when two benzene rings were simultaneously fused at the 5,6-position and 7,8-position of 2-phenylfuro[2,3-b]quinoxaline, the formed compound, diBFQ, presented a pure blue emission with a peak of 445 nm. To understand the reasons, we compared the electron distribution of their HOMO and LUMO orbitals. The electron distribution of the HOMO and LUMO orbitals for 26dPFQ, 27dPFQ, and BFQ are provided in Fig. S2 (ESI†). It was found that both HOMO and LUMO wavefunctions are distributed over the whole π-conjugate scaffold in BFQ. However, in diBFQ, most of the electron distribution for the LUMO orbital is localized on the 2-phenylfuro[2,3-b]quinoxaline fragment and in addition a minor contribution from the fused benzene ring of the 7,8-position of diBFQ, while the fused benzene ring from the 5,6-position of diBFQ almost has no contribution to the LUMO wavefunction. This phenomenon was found in 26dPFQ, where the substituent at the 6-position of the furo[2,3-b]quinoxaline scaffold has little effect on its photophysical properties. Thus, the localizing property of the LUMO wavefunction in diBFQ makes it present a blue emission. Moreover, we can also infer that substitution on the 1/2/3/4-position of diBFQ (as shown in Scheme 1) may have a little effect on the optical properties of resulting compounds. This can be demonstrated from calculated HOMO and LUMO orbitals in dP-diBFQ, where the isopropylphenyl at the 3-position of diBFQ makes negligible contribution to the wavefunction of both HOMO and LUMO orbitals (see Fig. 3).
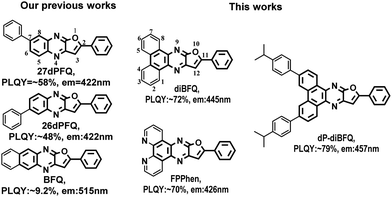 |
| Scheme 1 The molecular structures in our previous works and in this work. | |
The PLQYs of the diBFQ and FPPhen in dilute toluene solutions were determined to be 0.72 and 0.70, respectively, which are comparable to that of the well-known molecule scaffolds of anthracene18–20 and pyrene,15,21–23 such as 2,6-diphenylanthracene,24 2,6-di(2-naphthyl)anthracene,25 and other anthracene derivatives,26 as well as 1,6/2,7-trans-β-styrylpyrenes.27 Notably, the PLQYs of diBFQ and FPPhen are higher than those in our reported molecule scaffold, 2,6/7-diphenylfuro[2,3-b]quinoxaline, indicating this scaffold is more emissive. For the disubstituted compound dP-diBFQ, the PLQY increases to 0.79. This can be understood as that the diBFQ molecule tends to be aggregated in solution due to large a π-conjugate plane28 while introducing isopropylphenyl to diBFQ suppresses this aggregation, leading to an increase of fluorescence emission capability.
The transient PL spectroscopies of the three compounds in toluene solution are shown in Fig. 1d. The three compounds exhibit a single exponential decay profile of the excited state relaxation in solution. The estimated fluorescence lifetimes for diBFQ is 1.05 ns, comparable to that of FPPhen (1.26 ns). The lifetime for the disubstituted compound, dP-diBFQ (1.76 ns), is slightly longer than that of unsubstituted diBFQ. We also noticed that the lifetimes of the three compounds in this manuscript are significantly shorter than that of 26dPFQ (3.07 ns). Moreover, the shortening of the lifetime is accompanied by an increased PLQY for diBFQ, FPPhen and dP-diBFQ as compared with that of 26dPFQ.
2.3. Thermal stability and electrochemical properties
As shown in Fig. 2a, only a quasi-reversible redox wave was observed for the three compounds during positive scanning, and their onset redox potentials (vs. Fc/Fc+) are 1.15, 1.29 and 1.27 V for diBFQ, FPPhen and dP-diBFQ, respectively. Thus, the HUMO energies were calculated to be −5.95/−6.09/−6.07 eV for diBFQ/FPPhen/dP-diBFQ, respectively. Thus, their LUMO energies corresponded as −3.01 eV, −2.95 eV and −3.23 eV for diBFQ, FPPhen and dP-diBFQ, respectively, using the equation LUMO = −(Eoptg − HOMO). Obviously, FPPhen shows a slightly lower HOMO energy than diBFQ, consistent with the trend of theoretical calculations.
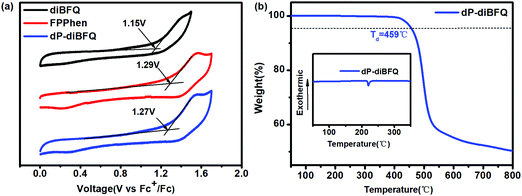 |
| Fig. 2 (a) The cyclic voltammetry curves of diBFQ, FPPhen, and dP-diBFQ in CH3CN solution and (b) the TGA curve of dP-diBFQ (the inset is the DSC curve). | |
The thermal gravimetric analysis and differential scanning calorimetry, as shown in Fig. 2b, show that the 5% weight loss temperature (Td) of dP-diBFQ is 459 °C, and the melting temperatures was at 220.7 °C, indicating it has a good thermal stability.
2.4. Theoretical calculations
The effects of the substituent in dP-diBFQ and the N atoms in FPPhen on the photophysical properties of resulting the compounds were further investigated by DFT and TD-DFT calculations at the B3LYP/6-31G(d) level of theory. Fig. 3 illustrates the configurations of the molecules, and the frontier molecular orbitals as well as their corresponding energy level values. It can be seen that both compounds, diBFQ and FPPhen, show the same electron distribution as the LUMOs orbital, where most of the electron distribution for the LUMO orbitals was localized on 2-phenylfuro[2,3-b]quinoxaline fragment with minor contribution from the fused benzene/pyridine ring of the 7,8-position of diBFQ/FPPhen. But, the wavefunction of their HOMOs displays a different electron distribution. The HOMO orbital of diBFQ is delocalized over the whole π-conjugate scaffold, whereas for FPPhen, the HOMO orbital is localized on the same fragment as its LUMO orbital, which results in a shifting of the HOMO energy level further away from the zero-point energy, increasing energy gap between the S1 and S0 states. Just for this reason, the FPPhen shows a deep-blue emission of 424 nm in CH2Cl2 solution, about a 20 nm blueshift in comparison with that of diBFQ (445 nm). When two iso-propylphenyls were incorporated the to 3 and 6-positions of diBFQ to produce a compound dP-diBFQ, the electron distribution of the LUMO orbital was not perturbed, while electron delocalization of the HOMO orbital further extended to the isopropylphenyl unit at the 6-position of dP-diBFQ in addition to the π-conjugate scaffold of diBFQ, which shifts the energy level of the HOMO orbital toward zero-point energy. Thus, the emission of dP-diBFQ shows a slight red-shift in comparison to that of diBFQ, just as the experimental observation. Theoretical calculation shows that dP-diBFQ (1.4921) has a larger oscillator strength of the S1 state over those of diBFQ (1.2232) and FPPhen (1.2910), and the enlarging of the oscillator strength for the S1 state is accompanied by an increased fluorescence efficiency, observed in experiments, indicating that the LUMO → HOMO transition mainly contributed to the light emission in the three compounds.
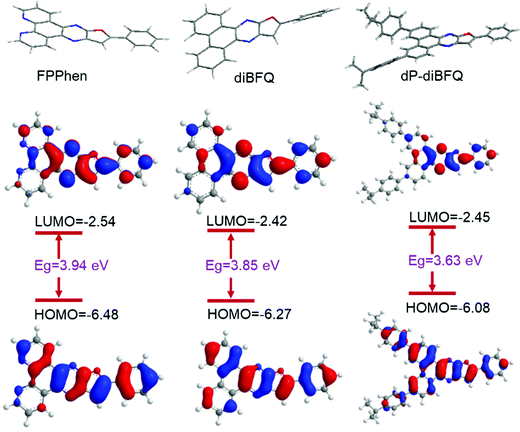 |
| Fig. 3 The calculated optimized molecular geometries and electron distributions of the HOMO and LUMO orbitals of diBFQ, FPPhen, and dP-diBFQ. | |
Table 2 EL performances of dP-diBFQ-based devices with the structure: ITO/MoO3 (7 nm)/TAPC (55 nm)/BH1140: dP-diBFQ (x%) (20 nm)/TPBi (40 nm)/LiF (1.0 nm)/Al (100 nm)
Dopant concentration (x%) |
V
on
[V] |
L
max
[cd m−2] |
EQEb [%] |
PEb [lm W−1] |
CEb [cd A−1] |
λ
EL
[nm] |
CIE(x, y)d |
Voltage values at a luminance of 1000 cd m−2.
Maximum luminance, EQE, CE and PE values.
The EL emission wavelength of devices at 10 V.
The CIE coordinates of the device at 10 V.
|
1% |
4.50 |
3407 |
2.28 |
2.21 |
2.11 |
444 |
(0.154, 0.102) |
2% |
4.50 |
5753 |
2.52 |
2.54 |
3.73 |
472 |
(0.154, 0.182) |
3% |
4.50 |
6505 |
2.36 |
2.57 |
3.85 |
476 |
(0.158, 0.208) |
4% |
4.30 |
7055 |
2.63 |
3.60 |
4.70 |
476 |
(0.161, 0.244) |
2.5. Electroluminescence properties
The molecules diBFQ and FPPhen tend to be aggregated in a solid film due to a large π-conjugate plane and, here, only dP-diBFQ was used as dopant to estimate the EL performances for this type of molecular scaffold. The device with structure, ITO (100 nm)/MoO3 (7 nm)/TAPC(55 nm)/BH1140:dP-diBFQ (x%) (20 nm)/TPBi (40 nm)/LiF (1.0 nm)/Al (100 nm) was fabricated, in which di-(4-(N,N-ditolylamino)phenyl) cyclohexane (TAPC) and 1,3,5-tri(phenyl-2-benzimidazolyl)benzene (TPBi) were used as hole and electron transporting materials, respectively. BH1144, a commercially used host with a HOMO/LUMO energy level of −5.68/−2.96 for blue emission, was selected as the host. For OLEDs, dopant concentrations that ranged from 1% to 4% were optimized, and the EL performances are shown in Fig. 4, and the data summarized in Table 2.
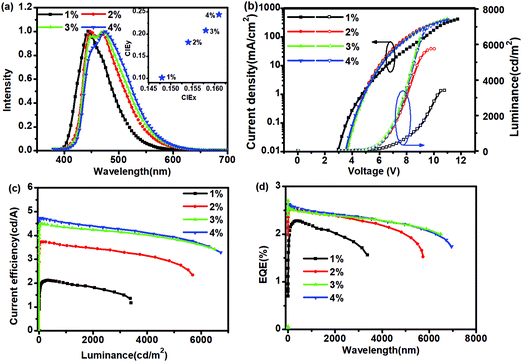 |
| Fig. 4 The EL performances of dP-diBFQ-based devices with different dopant concentrations: (a) the EL spectra (the inset shows the CIE coordinates with different doping concentrations); (b) the current density–voltage–luminance (J–V–L) curves; and the (c) current efficiency vs. luminance and (d) EQE curves. | |
The device doped with 1% dP-diBFQ displays a pure blue emission with an EL peak of 444 nm and CIE coordinates (0.154, 0.102), perfectly matching the NTSC blue standard for information displays. With increasing dopant concentration, a shoulder peak of 472–476 nm appeared and became a main peak. This shoulder peak should be ascribed to the aggregate emission of dP-diBFQ because this molecule tends to form aggregates due to its large π-conjugate plane. Correspondingly, the CIE coordinates shift to a CIExy of (0.154, 0.182)/(0.158, 0.208)/(0.161, 0.244) for the 2%/3%/4% doped devices, respectively. For the 1% doped device, the maximum luminance (Lmax) is 3407 cd m−2, and with increasing dopant concentration, the Lmax values for 2%/3%/4% doped devices increase to 5753 cd m−2/6492 cd m−2/7055 cd m−2, respectively. The 1% doped device achieves a maximum EQE and current efficiency of 2.28% and 2.11 cd A−1 at a luminance of 331.5 cd m−2. As for the 2% doped device, the EQEmax slightly improved to 2.52% while the CEmax increased to 3.73 cd A−1 at a luminance of 251.2 cd m−2. For the 3% doped device, the CEmax and EQEmax further increased to 4.47 cd A−1 and 2.53%, respectively, at a luminance of 122.8 cd m−2. The 4% doped device presents a further improvement in CEmax, 4.7d cd A−1, but little increase in EQEmax, 2.63%.
3. Conclusions
Two new molecular scaffolds showing efficient pure and deep blue emission, diBFQ and FPPhen, were synthesized and characterized. Both the compounds exhibit bright pure or deep blue emission with PLQYs of ∼70%. Introducing isopropylphenyl into the diBFQ scaffold further improved the PLQY of the resulting compound dP-diBFQ to ∼79%. The use of dP-diBFQ in OLEDs resulted in pure blue emission with CIE coordinates of (0.154, 0.102), very close to the NTSC blue standard, and an EQE of 2.28%. We found the substituent, fusing position, and N atoms in this type of molecule scaffold significantly affected the photophysical properties of the resulting compounds. The high efficiency and stability of the device indicate that diBFQ is a promising molecular scaffold for deep and pure-blue OLEDs. When the steric substituent isopropylphenyl was introduced into dP-diBFQ, the dP-diBFQ molecules in devices show a clear tendency to form aggregates in the doped film. We are sure that highly efficient pure blue emitters could be achieved via selecting suitable substituents with larger steric hindrance, and through optimizing the substituent numbers and the substitution positions.
4. Experimental
4.1. Measurements
TGA-DSC measurements were carried out on a Shimadzu DTG-60 instrument from room temperature to 500 °C under a dry nitrogen flow with a heating rate of 10 °C min−1. Cyclic voltammograms were measured on a Princeton Applied Research model 273 A electrochemical workstation at a scan rate of 100 mV s−1 using 0.1 M tetrabutylammonium perfluorinated phosphorate (TBAPF6) as the supporting electrolyte in dry CH2Cl2. A glassy carbon, Pt-sheet and Pt-wire were employed as working electrode, counter electrode and reference electrode. Fc/Fc+ was used as the internal reference. UV-visible spectra of the solution were recorded on Hitachi 3010 spectrometers. The steady state and transient fluorescence spectra were measured on a FLS 980 fluorescent spectrometer, and fluorescence quantum yields (PLQY) were measured via using the integrating sphere in combination with FLS 980 spectrofluorometer.
4.2. Device fabrication and characterization
All the organic layers were successively deposited by means of vacuum deposition onto the ITO-coated glass substrates, which were previously etched, patterned, and washed with detergent, deionized water, acetone, and ethanol in turn. For the doped layer, the dopant and host materials were co-evaporated and the doping concentrations were controlled by deposition rates. The electroluminescence spectra and CIE coordinates of the devices were measured by a spectrometer (PR655) and the current–voltage–luminescence characteristics were analyzed using a Keithley 2400 source meter with PR655.
Conflicts of interest
The authors declare no competing financial interest.
Acknowledgements
We are grateful for financial support from the National Natural Science Foundation of China (Grant No. 61675163, Grant No. 62075178), Natural Science Basic Research Plan in Shaanxi Province of China (Program No. 2016JM6024), and China Postdoctoral Science Foundation (Grant No. 2015M572542). We thank the Instrumental Analysis Center of Xi'an Jiaotong University and the High-Performance Computing Center in Xi'an Jiaotong University for providing computational resources.
References
- M. A. Baldo, D. F. O'Brien, Y. You, A. Shoustikov, S. Sibley, M. E. Thompson and S. R. Forrest, Highly efficient phosphorescent emission from organic electroluminescent devices, Nature, 1998, 395(6698), 151–154 CrossRef CAS.
- S. Lamansky, P. Djurovich, D. Murphy, F. Abdel-Razzaq, H. E. Lee, C. Adachi, P. E. Burrows, S. R. Forrest and M. E. Thompson, Highly phosphorescent bis-cyclometalated iridium complexes: Synthesis, photophysical characterization, and use in organic light emitting diodes, J. Am. Chem. Soc., 2001, 123(18), 4304–4312 CrossRef CAS PubMed.
- A. Tsuboyama, H. Iwawaki, M. Furugori, T. Mukaide, J. Kamatani, S. Igawa, T. Moriyama, S. Miura, T. Takiguchi, S. Okada, M. Hoshino and K. Ueno, Homoleptic cyclometalated iridium complexes with highly efficient red phosphorescence and application to organic light-emitting diode, J. Am. Chem. Soc., 2003, 125(42), 12971–12979 CrossRef CAS PubMed.
- L. M. Canada, J. Kolling, Z. Wen, J. I. C. Wu and T. S. Teets, Cyano-isocyanide Iridium(III) complexes with pure blue phosphorescence, Inorg. Chem., 2021, 60(9), 6391–6402 CrossRef CAS PubMed.
- B.-S. Yun, S.-Y. Kim, J.-H. Kim, H.-J. Son and S. O. Kang, Homoleptic cyclometalated dibenzothiophene-NHC-iridium(III) complexes for efficient blue phosphorescent organic light-emitting diodes, J. Mater. Chem. C, 2021, 9(11), 4062–4069 RSC.
- S. Jinwon and C. Changwoong, Blue phosphorescent organic light-emitting didoes for future displays, SID Symp. Dig. Tech. Pap., 2021, 52(1), 336 CrossRef.
- Q. Zhang, J. Li, K. Shizu, S. Huang, S. Hirata, H. Miyazaki and C. Adachi, Design of efficient thermally activated delayed fluorescence materials for pure blue organic light emitting diodes, J. Am. Chem. Soc., 2012, 134(36), 14706–14709 CrossRef CAS PubMed.
- F. B. Dias, K. N. Bourdakos, V. Jankus, K. C. Moss, K. T. Kamtekar, V. Bhalla, J. Santos, M. R. Bryce and A. P. Monkman, Triplet harvesting with 100% efficiency by way of thermally activated delayed fluorescence in charge transfer OLED emitters, Adv. Mater., 2013, 25(27), 3707–3714 CrossRef CAS PubMed.
- Y. Im and J. Y. Lee, Above 20% external quantum efficiency in thermally activated delayed fluorescence device using furodipyridine-type host materials, Chem. Mater., 2014, 26(3), 1413–1419 CrossRef CAS.
- M. K. Etherington, F. Franchello, J. Gibson, T. Northey, J. Santos, J. S. Ward, H. F. Higginbotham, P. Data, A. Kurowska, P. L. Dos Santos, D. R. Graves, A. S. Batsanov, F. B. Dias, M. R. Bryce, T. J. Penfold and A. P. Monkman, Regio- and conformational isomerization critical to design of efficient thermally-activated delayed fluorescence emitters, Nat. Commun., 2017, 8, 14987 CrossRef CAS PubMed.
- M. Cai, M. Auffray, D. Zhang, Y. Zhang, R. Nagata, Z. Lin, X. Tang, C.-Y. Chan, Y.-T. Lee, T. Huang, X. Song, Y. Tsuchiya, C. Adachi and L. Duan, Enhancing spin-orbital coupling in deep-blue/blue TADF emitters by minimizing the distance from the heteroatoms in donors to acceptors, Chem. Eng. J., 2021, 420, 127591 CrossRef CAS.
- X. Song, D. Zhang, Y. Lu, C. Yin and L. Duan, Understanding and manipulating the interplay of wide-energy-gap host and TADF sensitizer in high-performance fluorescence OLEDs, Adv. Mater., 2019, 31(35), 1901923 CrossRef PubMed.
- W. Song, I. H. Lee, S.-H. Hwang and J. Y. Lee, High efficiency fluorescent white organic light-emitting diodes having a yellow fluorescent emitter sensitized by a blue thermally activated delayed fluorescent emitter, Org. Electron., 2015, 23, 138–143 CrossRef CAS.
- H. Nakanotani, T. Higuchi, T. Furukawa, K. Masui, K. Morimoto, M. Numata, H. Tanaka, Y. Sagara, T. Yasuda and C. Adachi, High-efficiency organic light-emitting diodes with fluorescent emitters, Nat. Commun., 2014, 5, 4016 CrossRef CAS PubMed.
- D. Zhang, L. Duan, C. Li, Y. Li, H. Li, D. Zhang and Y. Qiu, High-efficiency fluorescent organic light-emitting devices using sensitizing hosts with a small singlet-triplet exchange energy, Adv. Mater., 2014, 26(29), 5050–5055 CrossRef CAS PubMed.
- Y. X. Li, Y. Wu, Z. Qin, G. Wang, Y. Wu, D. D. Wang, Y. F. Zhang, X. Y. Wang, C. M. Wu and H. L. Dong, Substitution effect on solid parking motif and luminescence of di-phenylfuro[2,3-b]quionzaline isomers, Org. Electron., 2021 Search PubMed , under review (ORGELE-D-21-00605R1).
- G. Wang, J. Li, Y. Li, D. Wang, J. Zhang, Y. Wu, Y.-G. Zhen, Q. Tang, H. Ma, W. Hu, Z. Wu and A. K. Y. Jen, A new type of solid-state luminescent 2-phenylbenzo g furo 2,3-b quinoxaline derivative: synthesis, photophysical characterization and transporting properties, J. Mater. Chem. C, 2019, 7(31), 9690–9697 RSC.
- Z. Wu, S. Song, X. Zhu, H. Chen, J. Chi, D. Ma, Z. Zhao and B. Z. Tang, Highly efficient deep-blue fluorescent OLEDs based on anthracene derivatives with a triplet-triplet annihilation mechanism, Mater. Chem. Front., 2021, 5(18), 6978–6986 RSC.
- P. Nalaoh, N. Sungworawongpana, P. Chasing, W. Waengdongbung, P. Funchien, C. Kaiyasuan, T. Sudyoadsuk and V. Promarak, A dimeric pi-stacking of anthracene inducing efficiency enhancement in solid-state fluorescence and non-doped deep-blue triplet-triplet annihilation organic light-emitting diodes, Adv. Opt. Mater., 2021, 9, 2100500 CrossRef CAS.
- J. H. Hong, S. Im, Y. J. Seo, N. Y. Kim, C. H. Ryu, M. Kim and K. M. Lee, Effects of terminal biphenyl ring geometry on the photophysical properties of closo-o-carboranyl-anthracene dyads, J. Mater. Chem. C, 2021, 9(31), 9874–9883 RSC.
- Y. Hirai, A. Wrona-Piotrowicz, J. Zakrzewski, A. Brosseau, R. Guillot, R. Metivier and C. Allain, Mechanofluorochromism of pyrene-derived amidophosphonates, Photochem. Photobiol. Sci., 2020, 19(2), 229–234 CrossRef CAS PubMed.
- S. Cho, N. Hwang, S. E. Lee, Y. K. Kim and S. S. Yoon, Highly efficient blue organic light-emitting diodes based on diarylamine-substituted pyrene derivatives, J. Nanosci. Nanotechnol., 2019, 19(2), 1056–1060 CrossRef CAS PubMed.
- C. W. Kim, S. N. Park, S. B. Lee, J. J. Kim, H. W. Lee, Y. K. Kim and S. S. Yoon, Blue emitters based on aryl end-capped pyrene groups for OLEDs, J. Nanosci. Nanotechnol., 2016, 16(3), 2912–2915 CrossRef CAS PubMed.
- J. Liu, H. Zhang, H. Dong, L. Meng, L. Jiang, L. Jiang, Y. Wang, J. Yu, Y. Sun, W. Hu and A. J. Heeger, High mobility emissive organic semiconductor, Nat. Commun., 2015, 6, 10032 CrossRef CAS PubMed.
- J. Li, K. Zhou, J. Liu, Y. Zhen, L. Liu, J. Zhang, H. Dong, X. Zhang, L. Jiang and W. Hu, Aromatic extension at 2,6-positions of
anthracene toward an elegant strategy for organic semiconductors with efficient charge transport and strong solid state emission, J. Am. Chem. Soc., 2017, 139(48), 17261–17264 CrossRef CAS PubMed.
- A. Dadvand, W.-H. Sun, A. G. Moiseev, F. Belanger-Gariepy, F. Rosei, H. Meng and D. F. Perepichka, 1,5-, 2,6-and 9,10-distyrylanthracenes as luminescent organic semiconductors, J. Mater. Chem. C, 2013, 1(16), 2817–2825 RSC.
- H. Ju, K. Wang, J. Zhang, H. Geng, Z. Liu, G. Zhang, Y. Zhao and D. Zhang, 1,6-and 2,7-trans-beta-styryl substituted pyrenes wxhibiting both emissive and semiconducting properties in the solid state, Chem. Mater., 2017, 29(8), 3580–3588 CrossRef CAS.
- Z.-Q. Liang, Y.-X. Li, J.-X. Yang, Y. Ren and X.-T. Tao, Suppression of aggregation-induced fluorescence quenching in pyrene derivatives: photophysical properties and crystal structures, Tetrahedron Lett., 2011, 52(12), 1329–1333 CrossRef CAS.
Footnotes |
† Electronic supplementary information (ESI) available: Synthesis details, H NMR spectra, and HRMS spectra of the three compounds are presented. The UV and PL spectra, and calculated HOMO and LUMO orbitals for 26dPFQ, 27dPFQ, and BFQ are also provided. See DOI: 10.1039/d1nj04934h |
‡ Xin Ye Wang and Yuan Wu contributed equally to this work. |
|
This journal is © The Royal Society of Chemistry and the Centre National de la Recherche Scientifique 2022 |
Click here to see how this site uses Cookies. View our privacy policy here.