DOI:
10.1039/D2MO00247G
(Research Article)
Mol. Omics, 2023,
19, 72-83
Lamiophlomis rotata attenuates rheumatoid arthritis by regulating sphingolipid and steroid hormone metabolism†
Received
14th September 2022
, Accepted 13th October 2022
First published on 17th October 2022
Abstract
Rheumatoid arthritis (RA) is a chronic and progressive autoimmune disease. Lamiophlomis rotata (L. rotata) (Benth.) Kudo, an essential medicinal plant in traditional Tibetan medicine, is useful in treating RA. The purpose of this study was to evaluate L. rotata's anti-RA effect and to analyze its serum metabolites and lipids to predict the possible action pathways. Female and male rats were immunized with CFA to induce arthritis. Paw volumes were measured, and arthritis index analysis and histological analysis were performed to check the effects of L. rotata. ELISA was used to measure the levels of inflammatory cytokines (IL-1β, TNF-α, IL-6, and IL-10) and oxidative stress (MDA, SOD, GSH, and CAT). UPLC/Q-Orbitrap-MS was used to identify untargeted metabolites and lipids in serum. Metabolite validation was performed using UPLC/QQQ-MS. L. rotata application significantly reduced arthritis indices and paw swelling in AIA rats, and diminished inflammation and bone fractures in joint tissues. Sphingolipid (SP) and steroid hormone biosynthesis was found to be closely related to L. rotata's intervention in RA. In addition, our experiments also confirmed that females were more likely than males to develop RA. These findings provide clues and a scientific basis for the mechanism of L. rotata in treating RA.
1. Introduction
Rheumatoid arthritis is a multisystemic inflammatory autoimmune disease characterized by synovial tissue hyperplasia, inflammatory cell infiltration, and cartilage and bone destruction, and inadequate treatment can result in joint destruction, deformity, disability, and even death.1 The incidence rate of RA in women is about 2–3 times that of men, and it is more common in menopausal women and elderly women, as well as in perimenopausal women with low estrogen levels.2 The most commonly used clinical drugs for the treatment of RA are glucocorticoids, nonsteroidal anti-inflammatory drugs, and new biological agents. Although these drugs can effectively relieve the clinical signs and symptoms caused by inflammation, they cannot cure the disease and have significant toxic side effects, mainly gastrointestinal and renal damage.3 Traditional Chinese medicine (TCM) has attracted much attention lately for its capacity to lessen RA's symptoms.4,5
Lamiophlomis rotata (L. rotata) (Benth.) Kudo belongs to Lamiophlomis genus.6 It has been mentioned in numerous masterpieces of Tibetan medicine, such as “Si Bu Yi Dian” and “Jingzhu Materia Medica” and “Chinese Materia Medica” that L. rotata was able to treat “yellow-water” illness such as skin conditions, jaundice, rheumatism, etc.7 The main active ingredients of L. rotata have been shown to be analgesic, anti-inflammatory, haemostatic, antioxidant and immunomodulatory.6 Previous studies also confirmed that L. rotata exhibits anti-rheumatoid arthritis activities.8,9 In particular, iridoid glucosides from L. rotata have demonstrated their potential in the treatment of RA.10
Although oxidative stress and lipid peroxidation have been linked to the pathogenesis of RA, the changes in the lipid metabolism in RA patients remain unknown.11 Recently, various lipid bioactive products have been proposed to play an essential role in the chronic inflammatory process, including phospholipids/sphingolipids.12 Clinical data suggest that imbalances of sphingolipids (SPs) have been observed in serum, stool and synovial fluid of patients with RA.13,14 In addition, steroid hormones are also closely associated with rheumatoid arthritis.15 Metabolomics studies the overall metabolites in biological systems, analyzing the global profile and changes of metabolites in complex samples.16 At present, no research has been conducted on the metabolite changes caused by L. rotata treatment of RA. In this paper, we have evaluated the anti-RA activities of L. rotata in an adjuvant-induced arthritis (AA) model in female and male rats, as well as analyzed its serum metabolites and lipids to predict the possible pathways of action, supplying clues and a scientific basis for the mechanism of L. rotata in RA treatment and research, and development of practical TCM components.
2. Materials and methods
2.1 Plant material
The plant was collected from Mozhugongka County, Tibet Autonomous Region and was identified by Wu Honghua, associate researcher at Tianjin University of Traditional Chinese Medicine.
2.2 Reagents and materials
The following reagents were used in the study: Freund's complete adjuvant (CFA) (Sigma, USA); chromogenic grade formic acid (ACS, USA); the chromatography solvents methanol, acetonitrile, and isopropanol obtained from Fisher Scientific (Waltham, USA); methotrexate (MTX) was supplied by the Xinyi Pharmaceutical Company (Shanghai, China); cortisol (CORT), 17α-hydroxyprogesterone (17HPS), and progesterone (PS), all with 98% purity, purchased from MACKLIN (Shanghai, China); and corticosterone (CORTS), 11-desoxycorticosterone (11DCORTS), and specnuezhenide (internal standard, IS), all with 98% purity, bought from Yuanye Bio-Technology Co., Ltd (Shanghai, China). Determination kits for MDA, SOD, CAT, and GSH were provided by Nanjing Jiancheng Bioengineering Institute (Jiangsu, China); and enzyme-linked immunosorbent assay (ELISA) kits for IL-6, IL-10, IL-1β and TNF-α were obtained from ZCIBIO (Shanghai, China).
2.3 Animals
Sprague Dawley (SD) rats (males/females, 180–220 g) were purchased from Beijing Charles River Laboratories (certificate No. SCXK 2016-0011, Beijing, China). Rats were housed under specific pathogen-free conditions. All rats had free access to standard laboratory water and food and were allowed to acclimatize to our facility for one week before the initiation of any experiments. All protocols used in this study followed National Institutes of Health Guide for the Care and Use of Laboratory Animals and approved by the Institute of Radiation Medicine, Chinese Academy of Medical Sciences (Ethics approval number IRN-DWLL-2020127).
2.4 Preparation of L. rotata extracts
L. rotata was made using the following method: whole plant of the herb was macerated in methanol (g mL−1, 1
:
15) twice for 24 h each time. The methanol extract was concentrated, and the medicinal residues were mixed with 70% ethanol for reflux extraction. Before concentration, the extraction was repeated twice (2 h each time). Finally, the total extract was low-temperature freeze-dried, and the extraction rate was 23%. A previous scheme was followed for the chromatography and mass spectrometry experiments.17
2.5 Animal experimental design
Female and male rats were inoculated with complete Freund's adjuvant (CFA) to induce arthritis.18 This emulsion was injected into the rats’ left hind paw. Except for the rats designated as the normal group, all rats received this treatment before being randomly divided into various groups. The immunized rats (males and females) were randomly assigned to five groups: the negative control group (AA) that received no drug treatment; the positive control group (MTX, 1 mg kg−1); the 0.5 g kg−1L. rotata treated group; the 1 g kg−1L. rotata treated group; and the 2 g kg−1L. rotata treated group; each group had 12 rats (males/females, respectively). The normal control group (n = 12) neither received the CFA injection nor drug treatment. All rats were treated intragastrically for 28 days.
The animals were sacrificed on the last day, and the inflamed hind limbs were harvested and placed in physiological solutions for histopathological analysis. Prior to analysis, serum samples were kept at −80 °C. The level of swelling was indicated by hind paw volume, which was measured using a plethysmometer. Every seven days after the first set of injections, the hind paws of each rat were evaluated. The AI score was calculated.19,20 Score 0: no signs of erythema or swelling; score 1: minor erythema or swelling; score 2: moderate edema and signs including the tarsals; score 3: significant edema, restricted utilization of the joint, and signs extending to the metatarsals; and score 4: serious signs and excessive edema with joint rigidity including the entire hind paw. Each rat's total arthritis score was the sum of the four limb scores.
2.6 Inflammatory cytokines and oxidative stress
Malondialdehyde (MDA), glutathione (GSH), superoxide dismutase (SOD), catalase (CAT), and inflammatory cytokines (TNF-α, IL-6, IL-10, and IL-1β) were measured in serum supernatant. The expression of oxidative stress and inflammatory cytokines was monitored according to the manufacturer's instructions.
2.7 Histopathological examinations
Each mouse's hind limbs were fixed in 10% phosphate-buffered saline (PBS)-buffered formalin at room temperature, decalcified in 5% formic acid, and embedded in paraffin. Each limb was sectioned at a thickness of 5 μm and stained with hematoxylin and eosin (H & E).
2.8 Lipidomics analysis
Serum samples (100 μL) were prepared from normal control group (C), the negative control group (AA) and the 2 g kg−1L. rotata extract treated group (H); 300 μL methanol and 1 mL tert-butyl methyl ether were added to extraction. The mixture was vortexed for 15 seconds before being centrifuged at 12
000g for 10 minutes at −4 °C. The supernatant was dried with nitrogen, reconstituted by adding 100 μL methanol, vortexed, centrifuged again, and the upper liquid was prepared for analysis. The quality control (QC) sample was an equal mixing of each re-dissolved sample. Processed serum samples were analyzed by UPLC/Q-Exactive-Orbitrap MS. Mass spectrometry and chromatography conditions are provided in the ESI.†
2.9 Untargeted metabolomic analysis
Serum samples (100 μL of C, AA and H, respectively) were centrifuged at 12
000g for 10 minutes at −4 °C in 400 μL of ice-cold acetonitrile. The top layers were nitrogen-dried before being reconstituted with 100 μL of pre-cooled 80% methanol (v
:
v), vortexed, centrifuged again, and the supernatant transferred to a sample injection vial for detection. Each redissolved sample was mixed with equal parts for the QC sample. UPLC/Q-Exactive-Orbitrap MS was used for analysis and Mass spectrometry and chromatography conditions are provided in the ESI.†
2.10 Targeted metabolomics analysis
Serum (100 μL) was mixed with 1 mL of ethyl acetate (EA), and then centrifuged for 10 minutes. The upper layers were nitrogen dried. A 100 μL solution of 50% methanol (v
:
v) was added and redissolved, centrifuged, and the supernatant was transferred to the injection vial for detection. In a 50% methanol solution, CORT, CORTS, 11-CORTS, 17HPS, PS, and IS standards were prepared (v
:
v). The experiment was carried out via UPLC/QQQ-MS in positive- and negative-ion modes with an electrospray ionization source (ESI). Molecular docking was used to determine the target for the anti-inflammatory activity of L. rotata with these five steroids as the reverse predicted phenotype. Chromatography, mass spectrometry and molecular docking conditions are provided in the ESI.†
2.11 Statistical analysis
Orthogonal Partial Least Squares Discrimination Analysis (OPLS-DA) was performed using SIMCA (Version 14.1, USA). One-way analysis of variance (ANOVA) followed by the Tukey test for statistical significance was carried out. P < 0.05 were considered statistically significant by Prism 8 (Graph Pad Software, Inc. San Diego, CA, USA). MetaboAnalyst 5.0 (https://www.metaboanalyst.ca/) was used to screen differential metabolites and determine the corresponding perturbed metabolic pathways.
3. Results
3.1 Chemical composition of L. rotata
Fifty-eight components from L. rotata were identified using a combination of standard comparison and literature comparison approaches, including flavonoids, iridoid glycosides, and phenylethanoids.17Fig. 1 depicts the UPLC-HRMS profiles of L. rotata, and Table 1 lists the 58 chemical components identified by mass spectrometry.
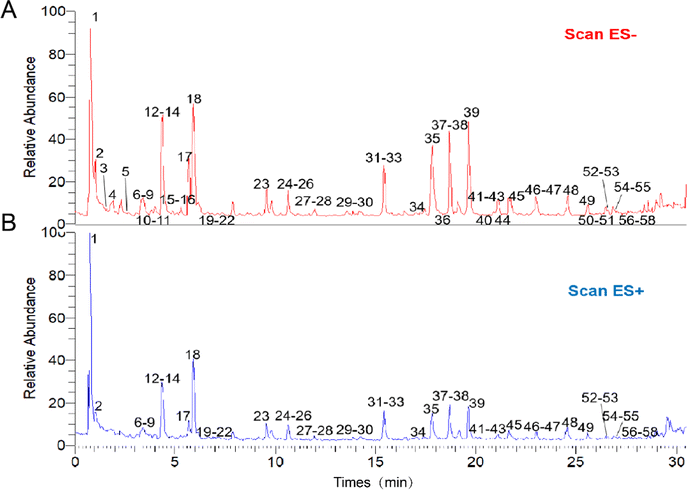 |
| Fig. 1 The UPLC-HRMS profiles of Lamiophlomis rotata. (A) The sample under negative ion mode. (B) The sample under positive ion mode. | |
Table 1 Chemical components of Lamiophlomis rotata identified by mass spectrometry
Peak number |
RT (min) |
Compound |
Formula |
Calculated (Da) |
Selected ion |
Precursor ion (Da) |
Error (ppm) |
1 |
0.77 |
Glucose |
C6H12O6 |
180.156 |
[M + Cl]− |
215.0321 |
−3.204 |
2 |
0.9 |
Sucrose |
C12H22O11 |
342.297 |
[M + Cl]− |
377.0855 |
−0.298 |
3 |
1.54 |
Quinic acid |
C9H12O6 |
192.167 |
[M − H]− |
191.0551 |
−5.293 |
4 |
1.91 |
Phlomiol |
C17H26O13 |
438.1373 |
[M + COOH]− |
483.1359 |
0.738 |
5 |
2.62 |
Vanillyl-β-D-glucopyranside |
C14H20O8 |
316.1158 |
[M − H]− |
315.1089 |
1.140 |
6 |
3.25 |
3,4-Dihydroxybenzaldehyde |
C7H6O3 |
138.0317 |
[M + H]+ |
139.0388 |
−1.227 |
7 |
3.33 |
Lamalbid |
C17H26O12 |
422.1424 |
[M + H]+ |
423.1487 |
−2.369 |
8 |
3.33 |
Schismoside |
C17H26O12 |
422.1337 |
[M + Na]+ |
445.1304 |
−5.266 |
9 |
3.36 |
Phlorigidoside C |
C17H24O11 |
404.1319 |
[M + H]+ |
405.1381 |
−2.562 |
10 |
3.76 |
Verbasoside |
C20H30O12 |
462.1723 |
[M − H]− |
461.1669 |
0.977 |
11 |
3.79 |
Decaffeoylverbascoside |
C20H30O12 |
462.1737 |
[M − H]− |
461.1668 |
1.487 |
12 |
3.94 |
Shanzhiside methyl ester |
C17H26O11 |
406.1475 |
[M + H]+ |
407.1537 |
−2.672 |
13 |
4.08 |
Salidroside |
C14H20O7 |
300.1200 |
[M − H]− |
299.1136 |
−0.087 |
14 |
4.40 |
Lamiophlomiol A/lamiophlomiol B |
C11H14O6 |
242.2253 |
[M − H]− |
241.0699 |
−7.721 |
15 |
4.40 |
Sesamoside |
C17H24O12 |
420.3653 |
[M + Na]+ |
443.1145 |
−3.379 |
16 |
4.56 |
Cistanoside F |
C21H28O13 |
488.1530 |
[M − H]− |
487.1456 |
−0.234 |
17 |
5.74 |
Chlorogenic acid or its isomers |
C16H18O9 |
354.3090 |
[M − H]− |
353.0879 |
0.268 |
18 |
5.98 |
5-Hydroxyloganin |
C17H26O11 |
406.1461 |
[M + H]+ |
407.1537 |
−2.672 |
19 |
6.32 |
Caffeic acid |
C9H8O4 |
180.0423 |
[M − H]− |
179.0343 |
−3.809 |
20 |
6.66 |
Chlorogenic acid or its isomers |
C16H18O9 |
354.3090 |
[M − H]− |
353.0880 |
0.551 |
21 |
6.83 |
Loganic acid |
C16H24O10 |
376.3560 |
[M − H]− |
375.1299 |
0.613 |
22 |
7.90 |
Phloyoside II |
C17H25ClO12 |
456.1034 |
[M + Na]+ |
479.0914 |
−4.951 |
23 |
9.42 |
7-epi-Loganin |
C17H26O10 |
390.1526 |
[M + H]+ |
391.1590 |
−2.233 |
24 |
10.60 |
Penstemoside |
C17H26O11 |
406.1475 |
[M + Na]+ |
429.1357 |
−2.406 |
25 |
10.61 |
7,8-Dehydropenstemoside |
C17H24O11 |
404.1319 |
[M + H]+ |
405.1401 |
2.375 |
26 |
10.70 |
Verbenalin |
C17H24O10 |
388.3660 |
[M + H]+ |
389.1432 |
−2.630 |
27 |
13.91 |
2,6-Dimethyl-6-hydroxyocta-2,7-dienyl-O-D-glucopyranoside or its isomers |
C16H28O9 |
332.1835 |
[M + COOH]− |
377.1817 |
−0.015 |
28 |
11.94 |
Loganin |
C17H26O10 |
390.1526 |
[M + H]+ |
391.1589 |
−2.489 |
29 |
13.86 |
Forsythoside C |
C29H36O16 |
640.2003 |
[M − H]− |
639.1934 |
0.535 |
30 |
14.23 |
Campneoside II |
C29H36O16 |
640.2003 |
[M − H]− |
639.1936 |
0.848 |
31 |
14.90 |
Rutin |
C27H30O16 |
610.5175 |
[M + H]+ |
611.1592 |
−2.391 |
32 |
15.37 |
8-O-acetyl shanzhiside methyl ester |
C18H28O12 |
448.1581 |
[M + Na]+ |
471.1462 |
−2.329 |
33 |
17.50 |
Luteolin-7-O-β-D-apiofuranosyl (1 → 6)-β-D-glucopyranoside |
C26H28O15 |
580.1428 |
[M + H]+ |
581.1488 |
−3.946 |
34 |
17.82 |
Kaempferol-3-O-D-glucopyranoside |
C21H20O11 |
448.3770 |
[M + H]+ |
449.1065 |
−2.979 |
35 |
17.84 |
Luteolin-7-O-β-D-glucopyranside |
C21H20O11 |
448.1006 |
[M − H]− |
447.0933 |
0.035 |
36 |
18.53 |
Betonyoside A/betonyoside B |
C30H38O16 |
654.2160 |
[M − H]− |
653.2094 |
1.059 |
37 |
18.68 |
6-Syringyl-deoxysesamoside |
C26H32O15 |
584.1741 |
[M − H]− |
583.1663 |
−0.932 |
38 |
18.75 |
Forsythoside B |
C34H44O19 |
756.2477 |
[M − H]− |
755.2408 |
0.527 |
39 |
19.69 |
Verbascoside/isoverbascoside |
C29H36O15 |
624.2054 |
[M − H]− |
623.1985 |
0.572 |
40 |
21.01 |
Cosmosiin |
C21H20O10 |
432.378 |
[M − H]− |
431.0985 |
−0.302 |
41 |
21.05 |
Verbascoside/isoverbascoside |
C29H36O15 |
624.2054 |
[M − H]− |
623.1983 |
0.251 |
42 |
21.11 |
Apigenin-7-β-glucopyranoside |
C21H20O10 |
432.1056 |
[M + H]+ |
433.1121 |
−1.901 |
43 |
21.37 |
Orobanchoside |
C29H34O15 |
622.1898 |
[M − H]− |
621.1831 |
0.977 |
44 |
21.73 |
Alyssonoside |
C35H46O19 |
770.7280 |
[M − H]− |
769.2567 |
0.842 |
45 |
21.77 |
Luteolin-7-O-β-D-(6′′-O-acetate)-glucopyranoside |
C23H22O12 |
490.1111 |
[M − H]− |
489.1038 |
−0.101 |
46 |
23.07 |
2′′-Acetylastragalin |
C23H22O12 |
490.1111 |
[M − H]− |
489.1039 |
0.104 |
47 |
23.16 |
Leucosceptoside A |
C30H38O15 |
638.2211 |
[M − H]− |
637.2144 |
0.952 |
48 |
23.32 |
Cistanoside C |
C30H38O15 |
638.6140 |
[M − H]− |
637.2145 |
1.109 |
49 |
24.63 |
Lamiophlomioside A |
C36H48O19 |
784.2790 |
[M − H]− |
783.2723 |
0.763 |
50 |
25.72 |
8-epi-7-Deoxyloganin |
C19H26O9 |
374.1577 |
[M + Na]+ |
397.1461 |
−0.268 |
51 |
26.46 |
6β-n-Butoxy-7,8-dehydropenstemonoside |
C21H32O10 |
444.1995 |
[M + H]+ |
445.2059 |
−2.075 |
52 |
26.88 |
Luteolin |
C15H10O6 |
286.0477 |
[M − H]− |
285.0407 |
0.838 |
53 |
27.01 |
Martynoside |
C31H40O15 |
652.6400 |
[M − H]− |
651.2302 |
1.162 |
54 |
28.18 |
α-Terpineol-β-D-O-glucopyranoside |
C16H28O6 |
316.1886 |
[M + COOH]− |
361.1873 |
1.409 |
55 |
28.58 |
Apigenin-7-O-(6′′-O-4-coumaroyl)-β-glucopyranoside |
C30H26O12 |
578.1389 |
[M − H]− |
577.1359 |
1.301 |
56 |
28.66 |
Hyperoside |
C21H20O12 |
464.3763 |
[M + H]+ |
465.1016 |
−2.478 |
57 |
28.66 |
Apigenin |
C15H10O5 |
270.0528 |
[M − H]− |
269.0460 |
1.685 |
58 |
29.24 |
Salviifoside A |
C20H22O9 |
406.1264 |
[M + Na]+ |
429.1162 |
1.390 |
3.2
L. rotata treatment prevents the progression of rheumatoid arthritis in rats
SD rats immunized with CFA were assigned to four treatment groups and administered with the indicated drug orally after the disease was established (Fig. 2A). As Fig. 2B, C show, L. rotata application significantly retarded the progression of RA in rats of different genders until the end of the experiment, and L. rotata intervention had more potent therapeutic effects in female RA rats than in male rats at the corresponding dosages. In addition, L. rotata administration markedly improved paw swelling in CFA rats (Fig. 2D–G). Stained ankle joints of AA rats contained the most osteoclast clusters and ameliorated joint pathology, including pannus formation, inflammatory cellular infiltration, synovial proliferation, and cartilage destruction. Synovial hyperplasia and inflammatory cellular infiltration were somewhat less extensive in animal groups treated with different dosages of L. rotata (Fig. 2H and I). Similarly, we found that L. rotata improved paw swelling and joint pathology in female arthritis rats induced by CFA, superior to males.
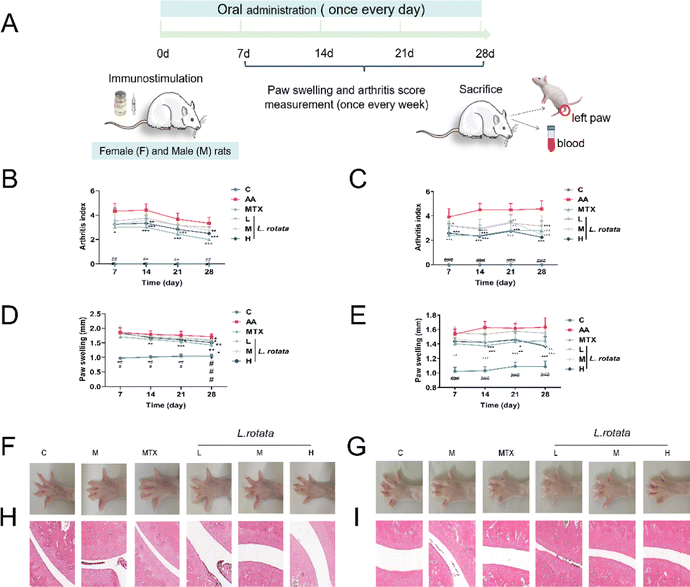 |
| Fig. 2
L. rotata prevented arthritis progression in CFA rats. (A) Experimental design of CFA induction and the treatment strategy. (B and C) Arthritis index scores were recorded every week after administration; (D and E) the thickness of left paw swelling was measured at every week; (F and G) representative photographs displaying the gross features of the left hind paws; and (H and I) representative ankle joint sections stained with H & E (magnification of ×200). B, D, F and H represent female rats, and C, E, G and I represents male rats. | |
3.3
L. rotata induced anti-inflammatory and antioxidative stress of rheumatoid arthritis rats
The serum expression levels of crucial inflammatory cytokines in RA rats treated with different dosages of L. rotata were determined for evaluation of the progression of the arthritis disease. TNF-α, IL-1β, and IL-6 expression levels were significantly inhibited in the serum of female arthritis rats treated with 2 g kg−1L. rotata extracts and MTX, whereas IL-10 expression levels were enhanced in the small dose L. rotata extracts (0.5 g kg−1 and 1 g kg−1L. rotata) treated group (Fig. 3A–D). However, in male arthritis rats, a high dose of L. rotata (2 g kg−1 L−1) had a weak effect on reducing IL-1β, IL-6, and TNF-α expression levels while significantly increasing the IL-10 levels (Fig. 3E–H).
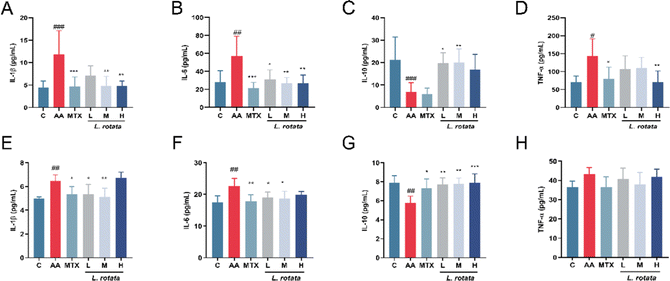 |
| Fig. 3 The serum concentration of inflammatory cytokines. (A–D) The female rat model of RA; (E–H) the male rat model of RA (n = 12, *P < 0.05, **P < 0.01, ***P < 0.001, 0.5 g kg−1L. rotate (L), 1 g kg−1L. rotate (M), 2 g kg−1L. rotate (H) and MTX treated RA rats separately compared with RA model rats, #P < 0.05, ##P < 0.01, ###P < 0.001, normal rat group compared with RA model rats). | |
To further investigate whether L. rotata affects oxidative stress in vivo, we evaluated the effects of L. rotata treatment on the activities of antioxidant enzymes SOD, lipid peroxidation product malondialdehyde (MDA), GSH peroxidase and catalase (CAT) content in serum. As illustrated in Fig. 4, in female rats, L. rotata (0.5 and 1 g kg−1) prevented GSH depletion and cleared MDA levels (Fig. 4B and C). However, the expression of endogenous antioxidants such as CAT and SOD did not increase significantly (Fig. 4A and D). In female rats, the contents of MDA, SOD, GSH, and CAT in AA rats changed but were not significant when compared to those in the healthy control group. As a result, the administration group's therapeutic effect was less than ideal (Fig. 4E–H).
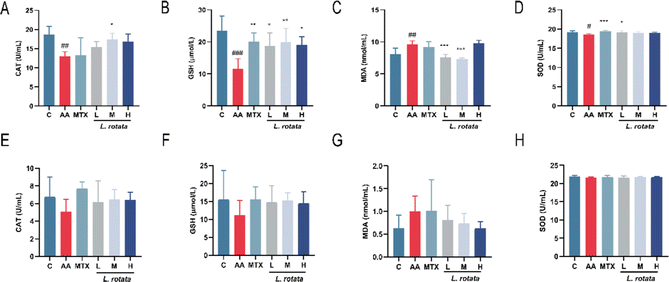 |
| Fig. 4 The serum concentration of oxidative stress factor. (A–D) The female rat model of RA; (E–H) the male rat model of RA (n = 12, *P < 0.05, **P < 0.01, ***P < 0.001, 0.5 g kg−1L. rotate (L), 1 g kg−1L. rotate (M), 2 g kg−1L. rotate (H) and MTX treated RA rats separately compared with RA model rats, #P < 0.05, ##P < 0.01, ###P < 0.001, normal rat group compared with RA model rats). | |
3.4 Lipidomics reveals the alteration in lipids in RA rats that were restored by L. rotata
3.4.1 Serum lipid profiling by Q-Exactive-Orbitrap MS.
Total ion chromatograms (TICs) from healthy control rats (C), CFA-arthritic rats (AA) and 2 g kg−1 dose L. rotata (H) reveal differences in lipids (Fig. S1, ESI†). QC sample fluctuations were within the allowable range in both positive and negative ion mode, indicating relatively stable experimental operation and instrument status (Fig. S2, ESI†). The lipid profile of C vs. AA vs. H was described using the OPLS-DA model. Results indicated a distinct separation between the three samples, indicating significant differences in their lipids (Fig. S2, ESI†).
3.4.2 Detection and identification of differential lipids.
Fold change > 2 and P < 0.05, VIP > 1.0, and lipids were considered significantly different compounds. For female rats, a total of 478 and 660 lipids were discovered in C vs. AA and AA vs. H, respectively, using the ESI+ and ESI− detection modes. Additionally, for male rats, C vs. AA and AA vs. H, respectively, revealed 516 and 425 lipids. Visualization results were displayed on a volcanic map (Fig. S3, ESI†). Venn analysis was implemented to make further efforts to determine the intervention effect of L. rotata on the lipids of RA rats. 123 compounds were screened as the differential lipids in female rats, including 52 lipids that were down-regulated in H rats, which were originally increased in AA rats, and 71 lipids were up-regulated in H rats, which were initially decreased in AA rats (Fig. 5A and Table S2, ESI†). In male rats, 58 differential lipids were identified, including 52 lipids that were down-regulated in H rats, which were originally increased in AA rats, and 6 lipids were up-regulated in H rats, which were initially decreased in AA rats (Fig. 5B and Table S3, ESI†).
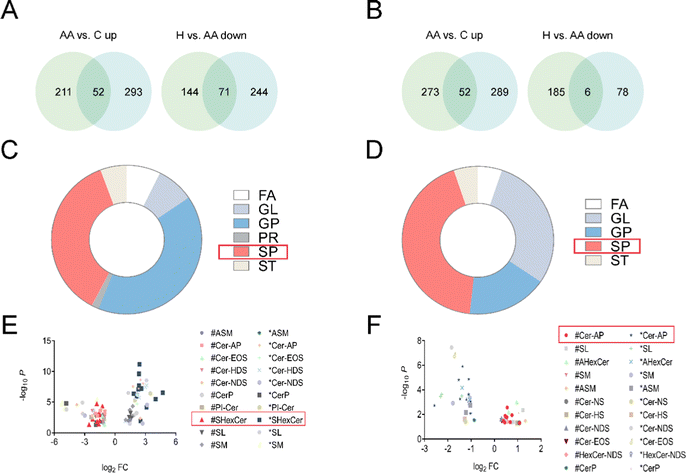 |
| Fig. 5 Lipidomics analysis of the impact of L. rotata on CFA-induced arthritis in rats. (A and B) Venn analysis for differential lipids in C vs. AA and AA vs. H; (C and D) differential lipid type analysis; (E and F) differential lipid subclass analysis. A, C and E represent female rats, and B, D and F represent male rats. | |
3.4.3 Differential lipid type and subclass analysis.
Different lipids serve different physiological functions, necessitating lipid subclass analysis. The most affected lipids after L. rotata administration in female rats were glycerophospholipids (GP), with 50 subclasses, and sphingolipids (SPs), with 45 subclasses (Fig. 5C). Furthermore, in male rats, the lipids with the greatest impact were 25 subclasses of SPs, 17 subclasses of glycerolipids (GL), and 10 subclasses of GP after L. rotata administration (Fig. 5D). A comprehensive review revealed that the differential lipids after L. rotata intervention were primarily SPs. Cer was the most abundant sphingomyelin in female and male rats, but the structure of its attached sphingosine and fatty acid differed. Statistical analysis revealed that sulfatide (SHexCer) and ceramide-hydroxy fatty acid-phytosphingosine (Cer-AP) were the most influential sphingolipids in female/male rats after L. rotata treatment. The detailed results of the sphingolipid subclass analysis are shown in Fig. 5E and F.
3.4.4
L. rotata treats RA by modulating the sphingolipid pathway.
Sphingolipid metabolites are associated with RA. Cer is an important central link in sphingolipid metabolism, and there are three main synthetic pathways, namely de novo synthesis, the sphingomyelinase pathway, and the salvage pathway. Cer reversibly generates ceryl 1-phosphate (C1P) and sphingosine (Sph), which further generates sphingosine 1-phosphate (S1P). In addition, Cer would also link with sugars to form cerebrosides and gangliosides. The sphingolipid metabolic pathway is shown in Fig. S4 (ESI†). Metabolites highlighted in red show the difference between AA rats and H rats. Sphingomyelin (SM), hexosylceramide (HexCer), SHexCer, Cer, and C1P were the main metabolites that were altered.
3.5 Metabolomics reveals metabolite perturbations in RA rats that were restored by L. rotata
3.5.1 Serum metabolic profiling by Q-Exactive-Orbitrap MS.
Representative TICs of the three groups (C, AA, and H) reveal metabolic changes (Fig. S5, ESI†). Concentrated score graphs of QC samples show strong instrument accuracy and stability throughout data collection (Fig. S6, ESI†). The OPLS-DA results revealed a clear separation between the three samples, implying that their metabolites differed significantly (Fig. S6, ESI†).
3.5.2 Detection and identification of the differential metabolites.
Metabolites with fold change > 1.2 and adjusted p-values < 0.05, VIP > 1.0 were considered significantly different. 119 and 162 metabolites were identified in female rats in C vs. AA and AA vs. H, respectively. Only 74 and 68 metabolites were identified in male rats in C vs. AA and AA vs. H, respectively. The findings were plotted on a volcanic map (Fig. S7, ESI†).
Venn analysis showed that 28 compounds were chosen as differential metabolites in female rats, with 3 metabolites down-regulated in H rats, which were previously increased in AA rats, and 25 metabolites up-regulated in H rats, which were previously decreased in AA rats (Fig. 6A and Table S4, ESI†). In male rats, 29 differential metabolites were identified, including 22 metabolites that were down-regulated in H rats, which were originally increased in AA rats, and 7 metabolites were up-regulated in H rats, which were initially decrease in AA rats (Fig. 6B and Table S5, ESI†). Heatmaps provide a global view of all the different metabolites. The heatmaps show that there are significant differences in metabolites between AA rats and H rats (Fig. 6C and D). Interestingly, female rats’ metabolites exhibited better aggregation than those of males.
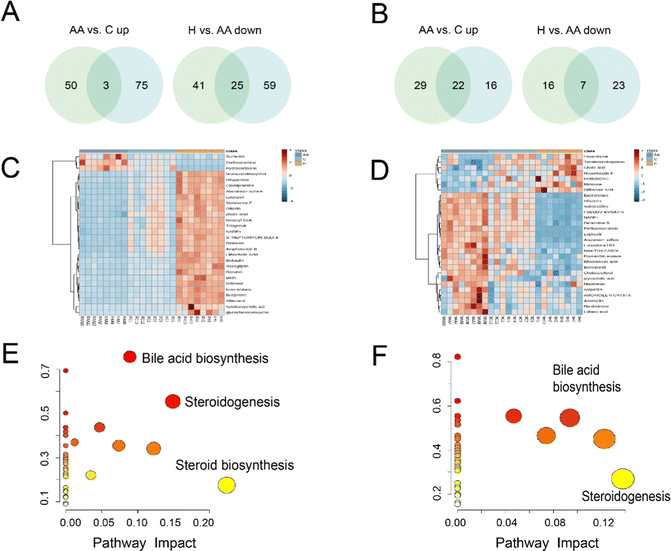 |
| Fig. 6 Metabolomic analysis of the impact of L. rotata on CFA-induced arthritis in rats. (A and B) Venn analysis for differential metabolites in C vs. AA and AA vs. H. (C and D) Heatmap representation of the levels of the differential metabolites in various groups with or without treatment with L. rotata. (E and F) Main perturbed metabolic pathways in RA salvaged by L. rotata treatment. A, C and E represent female rats, and B, D and F represent male rats. | |
3.5.3 KEGG pathway enrichment analysis.
To investigate the most relevant pathways and potential mechanisms, the KEGG (Kyoto Encyclopedia of Genes and Genomes) online database was used for pathway enrichment analysis. Based on the p values and pathway-rich factors from pathway topology analysis, pathway enrichment analysis identified all matched pathways. In our study, three KEGG pathways, including steroid hormone biosynthesis, steroid biosynthesis, and bile acid biosynthesis, were the mainly influenced by L. rotata therapy in AA female rat (Fig. 6E). In addition, steroid hormone biosynthesis and bile acid biosynthesis were also the most important pathways of L. rotata therapy in arthritic male rats (Fig. 6F). We found that steroid hormone biosynthesis was closely related to RA, so further research on the steroid hormone biosynthesis pathway was carried out.
3.5.4
L. rotata treats RA by modulating the steroid biosynthetic pathway.
Based on untargeted metabolomics, the most important pathway of L. rotata therapy in arthritic female/male rats was steroid hormone biosynthesis. Cortisol (CORT) and corticosterone (CORTS), two steroid hormones, were screened. UPLC/QQQ-MS was used to detect CORT, CORTS, and relevant steroid hormones such as progesterone (PS), 17-hydroxyprogesterone (17HPS), and 11-deoxycorticosterone (11DCORTS). The linear regression equation for the five steroid hormones were accurately established (Table S6, ESI†). Furthermore, precision (intra-day and inter-day), extraction recovery, matrix effect, and stability were investigated (Table S7, ESI†). CORT, CORTS, PS, 17HPS, and 11DCORTS levels decreased significantly after L. rotata therapy in AA female rats (Fig. 6A). However, there was no significant increase in the content of the five steroids in AA male rats, and treatment with L. rotata was not promising (Fig. 6B). This finding was consistent with previous anti-inflammatory and antioxidative stress studies, which found that the occurrence of RA was related to gender.
We used CORT, CORTS, 11DCORTS, and 17HPS, PS as phenotypes for reverse prediction to determine the target of anti-inflammatory L. rotata. Metabolite target enrichment was performed with a score of >0.7, and three targets were screened: namely glucocorticoid receptor, corticosteroid-binding globulin, and sex hormone-binding globulin. The anti-inflammatory active ingredients (apigenin, luteolin, caffeic acid, 2′′-acetylastragalin, astragalin 6-gallate and hyperoside) were screened by network pharmacology in our prior study.17 Therefore, these 6 active components were employed as small molecule ligands to dock with each of the aforementioned 3 proteins. Results revealed that the 6 compounds had strong binding affinity for the 3 receptor proteins and low conformational energy (Fig. 6C–E). It was speculatively determined that L. rotata flavonoids were the active ingredients for the treatment of RA, which may impact the steroid hormone biosynthesis pathway by acting on glucocorticoid receptors, corticosteroid-binding globulin, and sex hormone-binding globulin.
4. Discussion
4.1 Sex and rheumatoid arthritis
The majority of patients with RA are middle-aged women, usually more than 70% in any RA cohort.21 Our study established female and male rat RA models using CFA. The paw swelling, arthritis index, and ankle joint pathology indices showed that female rats had more severe arthritic lesions than males (Fig. 2). On the other hand, inflammatory cytokine and oxidative stress factor assays also suggested similar results (Fig. 3 and 4). According to the above findings, women are more likely than men to develop RA. Gender differences in RA can be explained at several levels, ranging from genetic factors to hormonal changes. Specific genes on the X and Y chromosomes are responsible for gonad differentiation and hormonal products, as well as determining an individual's phenotypic sex.22
4.2 Sphingolipids and rheumatoid arthritis
Phospholipids and glycolipids are components of SPs. Cer, which is the primary metabolite of sphingolipid metabolism, and its by-products C1P and S1P are the key active metabolites. These three sphingolipids have been extensively researched in relation to immune-dependent and vascular-related chronic inflammatory disorders.23 A number of studies suggest that SPs were involved in the pathogenesis of RA and may be a potential therapeutic target.24 Cer is a universal precursor for the synthesis of more complex sphingolipids and is central to the metabolic network of sphingolipid synthesis and degradation.25 Untargeted lipidomics analysis revealed that the most affected metabolites were SPs, with the most profound impact on SHexCer and Cer-AP (Fig. 5).
4.3 Steroid hormones and rheumatoid arthritis
Glucocorticoids (GC) are the primary steroid hormones with anti-inflammatory and immunomodulatory properties in RA. Research has shown that glucocorticoids (CORT/CORTS) suppress the Th1 cell response while activating the Th2 cell response, inhibiting the immune response and causing inflammatory damage.26 The glucocorticoid receptor (GR) and the mineralocorticoid receptor (MR) mediate glucocorticoid negative feedback regulation in the hypothalamic–pituitary–adrenal axis (HPA). Inflammatory factors can activate the HPA axis and raise endogenous glucocorticoid levels during the inflammatory process. Exogenous GC preferentially binds to GR, whereas endogenous GC preferentially binds to MR. Endogenous GC can activate MR and produce a pro-inflammatory effect in an oxidative stress state.27 RA pathogenesis is associated with increased oxidative stress, decreased antioxidant levels, and impaired antioxidant defenses.
CORT and CORTS are two important steroid metabolites derived from the 17HPS and PS metabolic pathways, respectively, via the intermediate metabolites 11DCORT and 11DCORTS. Untargeted metabolomics results showed that steroid hormone biosynthesis was the most critical pathway of L. rotata therapy in arthritic rats (Fig. 6). The steroid hormones were then validated using targeted metabolomics. Because the concentration of 11DCORT was too low to detect, the final metabolites identified were CORT, CORTS, PS, 17HPS, and 11DCORTS. The contents of the five steroids listed above decreased significantly after L. rotata therapy in female RA rats (Fig. 7). This suggests that L. rotata treatment of RA is associated with endogenous steroid hormones, possibly by reducing endogenous GC binding to MR under oxidative stress.
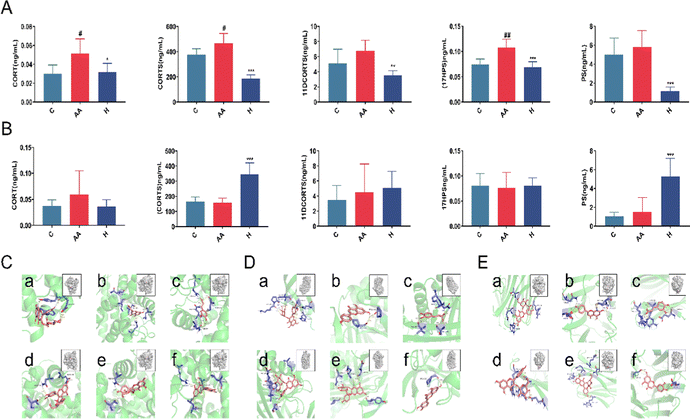 |
| Fig. 7 Targeted metabolomic analysis of steroid hormones and molecular docking. (A) The serum concentration of steroid hormones in female rats; (B) the serum concentration of steroid hormones in male rats; (C) molecular docking of glucocorticoid receptors, (D) corticosteroid-binding globulin, and (E) sex hormone binding globulin. (a) 2′′-Acetylastragalin; (b) apigenin; (c) caffeic acid; (d) hyperoside; (e) Kaempferol-3-O-D-glucopyranoside; and (f) luteolin. | |
4.4
L. rotata treats rheumatoid arthritis by regulating steroid hormones and sphingolipids
Sphingolipids like Cer, S1P, and Sph have recently been discovered to regulate steroid hormone secretion in a variety of ways.28 Cer primarily controls steroid hormones by acting as a second messenger in cytokine and growth factor signaling cascades,29 and regulates the activity of steroidogenic enzymes.30 On the other hand, sphingosine kinase (SK) can be activated by pro-inflammatory factors (TNF-α and IL-1β) to increase S1P,31 which can induce paracrine and/or autocrine cellular responses by binding to specific G protein-coupled receptors (S1PR) to perform its signaling functions intracellularly and extracellularly.32 S1P secreted in response to cAMP stimulation induces CYP17 transcription through a paracrine mechanism that requires S1PR activation and nuclear translocation of sterol regulatory element binding protein 1 (SREBP1).33 Sph binding to steroidogenic factor 1 (SF-1) decreases CYP17 expression, thereby reducing steroid hormone production.34 Sphingolipid metabolism can be quickly activated by adrenocorticotropic hormone (ACTH) in the adrenal cortex, which decreases SM, Cer, and Sph levels while increasing S1P secretion.35 Cortisol also stimulates Cer breakdown, elevates Sph concentration, and activates acidic ceramidase 1 (ASAH1).34
The relationship network of L. rotata–RA–sphingolipids–steroid hormones was discussed (Fig. 8). Internal and external factors cause RA, and immune cells and inflammatory cytokines are significantly elevated. Pro-inflammatory variables stimulate the HPA axis, which greatly raises steroid hormone concentrations and controls SMase and SK in the metabolism of sphingolipids, speeding up the buildup of Cer and S1P. In this study, differential lipids were primarily SPs, with specific SP subclasses focusing on Cer. It was speculated that L. rotata may mainly affect the sphingolipid metabolism centered on Cer to restore RA. Besides, differential metabolites were enriched into the steroid hormone biosynthesis pathway, and steroid hormone targeted validation confirmed that L. rotata administration can reduce the content of some steroid hormones. Network pharmacology and molecular docking have revealed that the active ingredients of L. rotata can act on the steroid pathway targets, primarily those related to cortisol binding and transport. Cortisol can regulate steroidogenesis via sphingolipid metabolism, and L. rotata was thought to affect both sphingolipid and steroid metabolism.
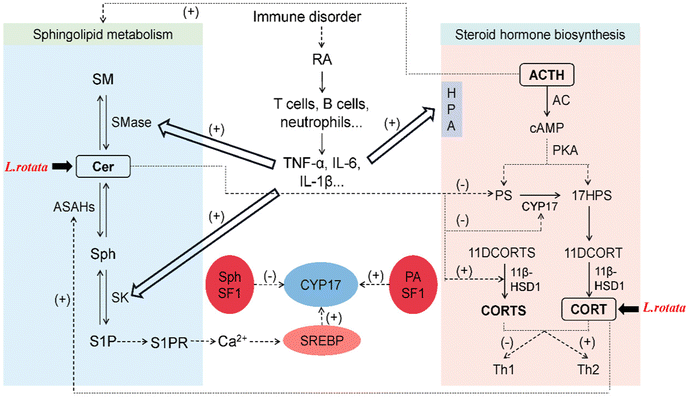 |
| Fig. 8 The relationship network of L. rotata–rheumatoid arthritis–sphingolipids–steroid hormones. | |
5. Conclusions
In general, our findings indicated that L. rotata has anti-RA properties. Sphingolipids and steroid hormones were found to be closely related to L. rotata's intervention in RA, and L. rotata may be anti-RA by regulating the content of Cer and its metabolites and decreasing steroid hormone production. The interaction of sphingolipids and steroid metabolism may become a research focus for treating RA. These findings provide hints and a scientific foundation for the mechanism of L. rotata's action in the treatment of RA.
Author's contribution
Zhirong Zhou: investigation, data curation, and writing-original draft. Tong Li: investigation, data curation, and writing-original draft. Ruijiao Du: methodology and data curation. Chengjuan Liu: formal analysis and software. Shengjie Huang: investigation and data curation. Lifeng Han: writing-review & editing. Peng Zhang: writing-review & editing. Yuefei Wang: writing-review & editing. Miaomiao Jiang: writing-review & editing and conceptualization.
Conflicts of interest
There are no conflicts to declare.
Acknowledgements
This study was supported by the Tianjin Committee of Science and Technology of China (No. 21ZYJDJC00080 and No. 20ZYJDJC00120).
References
- I. B. McInnes and G. Schett, Cytokines in the pathogenesis of rheumatoid arthritis, Nat. Rev. Immunol., 2007, 7(6), 429–442 CrossRef CAS PubMed.
- S. K. Tedeschi, B. Bermas and K. H. Costenbader, Sexual disparities in the incidence and course of SLE and RA, Clin. Immunol., 2013, 149(2), 211–218 CrossRef CAS PubMed.
- T. Watanabe, Y. Fujiwara and F. K. L. Chan, Current knowledge on non-steroidal anti-inflammatory drug-induced small-bowel damage: a comprehensive review, J. Gastroenterol., 2020, 55(5), 481–495 CrossRef CAS PubMed.
- R. Jing, Y. F. Ban, W. H. Xu, H. Nian, Y. L. Guo, Y. Y. Geng, Y. Zang and C. J. Zheng, Therapeutic effects of the total lignans from Vitex negundo seeds on collagen-induced arthritis in rats, Phytomedicine, 2019, 58, 10 CrossRef PubMed.
- S. Baig and D. D. DiRenzo, Complementary and Alternative Medicine Use in Rheumatoid Arthritis, Curr. Rheumatol. Rep., 2020, 22(10), 9 Search PubMed.
- Z. H. Cui, S. S. Qin, E. H. Zang, C. Li, L. Gao, Q. C. Li, Y. L. Wang, X. Z. Huang, Z. Y. Zhang and M. H. Li, Traditional uses, phytochemistry, pharmacology and toxicology of Lamiophlomis rotata (Benth.) Kudo: a review, RSC Adv., 2020, 10(19), 11463–11474 RSC.
- M. Li, Z. Jia, Z. Hu, R. Zhang and T. Shen, Experimental study on the hemostatic activity of the Tibetan medicinal herb Lamiophlomis rotata, Phytother. Res., 2008, 22(6), 759–765 CrossRef CAS PubMed.
- X. J. Huang, J. Wang, A. Muhammad, H. Y. Tong, D. G. Wang, J. Li, A. Ihsan and G. Z. Yang, Systems pharmacology-based dissection of mechanisms of Tibetan medicinal compound Ruteng as an effective treatment for collagen-induced arthritis rats, J. Ethnopharmacol., 2021, 272, 113953 CrossRef CAS PubMed.
- Y. Jiang, M. Zhong, F. Long, R. Yang, Y. Zhang and T. Liu, Network Pharmacology-Based Prediction of Active Ingredients and Mechanisms of Lamiophlomis rotata (Benth.) Kudo Against Rheumatoid Arthritis, Front. Pharmacol., 2019, 10, 1435 CrossRef CAS PubMed.
- X. H. Zhao, S. R. Jiang, Q. Dong, J. Dang, Z. G. Liu, H. P. Han, Y. D. Tao and H. L. Yue, Anti-rheumatoid arthritis effects of iridoid glucosides from Lamiophlomis rotata (Benth.) kudo on adjuvant-induced arthritis in rats by OPG/RANKL/NF-kappa B signaling pathways, J. Ethnopharmacol., 2021, 266, 8 CrossRef.
- H. B. Ferreira, T. Melo, A. Paiva and M. D. Domingues, Insights in the Role of Lipids, Oxidative Stress and Inflammation in Rheumatoid Arthritis Unveiled by New Trends in Lipidomic Investigations, Antioxidants, 2021, 10(1), 21 CrossRef PubMed.
-
A. Gomez-Larrauri, N. Presa, A. Dominguez-Herrera, A. Ouro, M. Trueba and A. Gomez-Munoz, Role of bioactive sphingolipids in physiology and pathology, in Lipid Mediators, ed. J. Harwood; E. LloydEvans, Portland Press Ltd, London, 2020, vol. 64, pp. 579–589 Search PubMed.
- D. Yu, J. P. Du, X. Pu, L. Y. Zheng, S. S. Chen, N. Wang, J. Li, S. Y. Chen, S. B. Pan and B. Shen, The Gut Microbiome and Metabolites Are Altered and Interrelated in Patients With Rheumatoid Arthritis, Front. Cell. Infect. Microbiol., 2022, 11, 14 Search PubMed.
- G. Miltenberger-Miltenyi, A. R. Cruz-Machado, J. Saville, V. A. Conceicao, A. Calado, I. Lopes, M. Fuller and J. E. Fonseca, Increased monohexosylceramide levels in the serum of established rheumatoid arthritis patients, Rheumatology, 2020, 59(8), 2085–2089 CrossRef PubMed.
- A. K. Carlson, R. A. Rawle, C. W. Wallace, E. Adams, M. C. Greenwood, B. Bothner and R. K. June, Global metabolomic profiling of human synovial fluid for rheumatoid arthritis biomarkers, Clin. Exp. Rheumatol., 2019, 37(3), 393–399 Search PubMed.
- W. Weckwerth and K. Morgenthal, Metabolomics: from pattern recognition to biological interpretation, Drug Discovery Today, 2005, 10(22), 1551–1558 CrossRef CAS PubMed.
- T. Li, L. Jia, R. J. Du, C. J. Liu, S. J. Huang, H. S. Yu, L. F. Han, X. P. Chen, Y. F. Wang and M. M. Jiang, Comparative investigation of aerial part and root in Lamiophlomis rotata using UPLC-Q-Orbitrap-MS coupled with chemometrics, Arab. J. Chem., 2022, 15(4), 13 Search PubMed.
- D. D. Obiri, N. Osafo, P. G. Ayande and A. O. Antwi, Xylopia aethiopica (Annonaceae) fruit extract suppresses Freund's adjuvant-induced arthritis in Sprague-Dawley rats, J. Ethnopharmacol., 2014, 152(3), 522–531 CrossRef.
- H. Z. Wang, H. H. Wang, S. S. Huang, H. Zhao, Y. G. Cao, G. Z. Wang, D. Wang, Z. G. Wang and Y. H. Liu, Inhibitory Effect of Baicalin on Collagen-Induced Arthritis in Rats through the Nuclear Factor-kappa B Pathway, J. Pharmacol. Exp. Ther., 2014, 350(2), 435–443 CrossRef.
- C. H. Li, H. M. Li, Q. Wang, M. L. Zhou, M. Li, T. Gong, Z. R. Zhang and X. Sun, pH-sensitive polymeric micelles for targeted delivery to inflamed joints, J. Controlled. Release, 2017, 246, 133–141 CrossRef CAS PubMed.
- E. Myasoedova, C. S. Crowson, H. M. Kremers, T. M. Therneau and S. E. Gabriel, Is the Incidence of Rheumatoid Arthritis Rising? Results From Olmsted County, Minnesota, 1955-2007, Arthritis Rheum., 2010, 62(6), 1576–1582 CrossRef PubMed.
- W. J. Kovacs and N. J. Olsen, Sexual dimorphism of RA manifestations: genes, hormones and behavior, Nat. Rev. Rheumatol., 2011, 7(5), 307–310 CrossRef CAS PubMed.
- M. D. Cas, G. Roda, F. Li and F. Secundo, Functional Lipids in Autoimmune Inflammatory Diseases, Int. J. Mol. Sci., 2020, 21(9), 19 Search PubMed.
-
S. Albeituni and J. Stiban, Roles of Ceramides and Other Sphingolipids in Immune Cell Function and Inflammation, in Role of Bioactive Lipids in Cancer, Inflammation and Related Diseases, ed. K. V. Honn, D. C. Zeldin, Springer International Publishing Ag, Cham, 2019, vol. 1161, pp. 169–191 Search PubMed.
- R. Loewith, H. Riezman and N. Winssinger, Sphingolipids and membrane targets for therapeutics, Curr. Opin. Chem. Biol., 2019, 50, 19–28 CrossRef CAS PubMed.
- D. A. Gonzalez, B. B. Diaz, M. D. R. Perez, A. G. Hernandez, B. N. D. Chico and A. C. de Leon, Sex hormones and autoimmunity, Immunol. Lett., 2010, 133(1), 6–13 CrossRef CAS.
- M. Nagase, N. Ayuzawa, W. Kawarazaki, K. Ishizawa, K. Ueda, S. Yoshida and T. Fujita, Oxidative Stress Causes Mineralocorticoid Receptor Activation in Rat Cardiomyocytes Role of Small GTPase Rac1, Hypertension, 2012, 59(2), 500–506 CrossRef CAS PubMed.
- N. C. Lucki and M. B. Sewer, The interplay between bioactive sphingolipids and steroid hormones, Steroids, 2010, 75(6), 390–399 CrossRef CAS PubMed.
- H. Y. Chung, J. Wickel, B. Stuhlmuller, R. W. Kinne and R. A. Claus, Acid sphingomyelinase activity is elevated in the serum of rheumatoid arthritis patients, suppressed by anti-TNF-alpha treatment, Clin. Exp. Rheumatol., 2019, 37(6), S18–S19 Search PubMed.
- S. B. Meroni, E. H. Pellizzari, D. F. Canepa and S. B. Cigorraga, Possible involvement of ceramide in the regulation of rat Leydig cell function, J. Steroid Biochem. Mol. Biol., 2000, 75(4–5), 307–313 CrossRef CAS PubMed.
- A. J. Snider, K. A. O. Gandy and L. M. Obeid, Sphingosine kinase: Role in regulation of bioactive sphingolipid mediators in inflammation, Biochimie, 2010, 92(6), 707–715 CrossRef CAS.
- A. Kihara, S. Mitsutake, Y. Mizutani and Y. Igarashi, Metabolism and biological functions of two phosphorylated sphingolipids, sphingosine 1-phosphate and ceramide 1-phosphate, Prog. Lipid Res., 2007, 46(2), 126–144 CrossRef CAS PubMed.
- T. Ozbay, A. Rowan, A. Leon, P. Patel and M. B. Sewer, Cyclic adenosine 5 '-monophosphate-dependent sphingosine-1-phosphate biosynthesis induces human CYP17 gene transcription by activating cleavage of sterol regulatory element binding protein 1, Endocrinology, 2006, 147(3), 1427–1437 CrossRef CAS PubMed.
- A. N. Urs, E. Dammer and M. B. Sewer, Sphingosine regulates the transcription of CYP17 by binding to steroidogenic factor-1, Endocrinology, 2006, 147(11), 5249–5258 CrossRef CAS PubMed.
- T. Ozbay, A. H. Merrill and M. B. Sewer, ACTH regulates steroidogenic gene expression and cortisol biosynthesis in the human adrenal cortex via sphingolipid metabolism, Endocr. Res., 2004, 30(4), 787–794 CrossRef CAS PubMed.
Footnotes |
† Electronic supplementary information (ESI) available: The raw data supporting the conclusions of this article are provided in the ESI. See DOI: https://doi.org/10.1039/d2mo00247g |
‡ These authors contributed equally to this work. |
|
This journal is © The Royal Society of Chemistry 2023 |