DOI:
10.1039/D2FO00578F
(Paper)
Food Funct., 2022,
13, 5528-5535
Development if healthy milk and yogurt products for reducing metabolic diseases using cyclodextrin and omega-3 fatty acids from fish oil†
Received
25th February 2022
, Accepted 25th April 2022
First published on 26th April 2022
Abstract
The food industry is constantly attempting to develop better products that will have a positive effect on health. Feiraco® and Clesa®, expressed their intention to create novel products using UNICLA® milk as a matrix to develop functional foods. In this respect, β-cyclodextrin (β-CD) at 1% was able to reduce the cholesterol concentration in Feiraco-UNICLA® milk products by around 87-85%. Products were fortified with omega-3 from fish oil with α- and β-CD acting as carriers. It was possible to add around 50% of eicosapentaenoic acid (EPA) and docosahexaenoic acid (DHA) Recommended Dietary Allowances (RDA), with a high diet proportion of fibre and similar organoleptic properties to commercial omega-3 products. 80% of a sensory panel found our formulations satisfactory. The final product was stable, and the bioaccessibilty of the fatty acids added to the milk was around 74%. These results as a whole satisfy the aid of Feiraco® and Clesa® to develop improved products.
1. Introduction
Society is increasingly demanding healthier food products, and one way to satisfy this demand could be to fortify foods with bioactive compounds, molecules that have demonstrated effects as antioxidants or anticancer activities, among others. Some common products, for example, milk or yogurt, are of great potential interest for improvement due to their intrinsic qualities.1 Although different strategies to improve their qualities are being studied,2,3 one strategy could be to eliminate the cholesterol fraction of milk in order to reduce its impact on cardiovascular diseases.4,5 The second one would be to fortify milk with omega-3 fatty acids, especially eicosapentaenoic acid (EPA; also eicosapentaenoic acid) and docosahexaenoic acid (DHA) are the most well-known fatty acids for their properties as cardiovascular protection agents, for preventing rheumatic arthritis, and as antioxidants, among other properties.6,7 The European Food Safety Authority (EFSA) recommends the consumption of between 250 and 500 mg per day of EPA and DHA based on cardiovascular risk considerations.8 A good source of these fatty acids (FAs) is fish oil, which also contains other interesting fatty acids such as oleic acid or linoleic, with their additional properties.6 However, the odour of the oil prevents good acceptance on the part of consumers.9 Moreover, the low solubility and stability of this family of compounds may contribute to obscuring the good organoleptic properties of the product in question.
Among many other10,11 strategies to attain both objectives, one promising strategy that can be used to increase the fish oil concentration without increasing unwanted odours and to eliminate cholesterol from milk is the complexation with cyclodextrins (CDs). These are torus-shaped oligosaccharides made up of α-(1,4) linked glucose units, the most common being α, β, and γ-CD, which contain six, seven, and eight glucose units, respectively.12,13 These types of natural CD have two GRAS statuses that appear in the lists of additives approved for alimentary use with the corresponding E-numbers: E-457, E-459, and E-458, respectively. The cavity of CDs is carpeted by hydrogen atoms and is therefore hydrophobic, unlike the outer surface of the molecule, where the primary and secondary hydroxyl groups are exposed to solvent (hydrophilic part), making the whole molecule highly water-soluble.14 Poorly water-soluble compounds and hydrophobic moieties of amphiphilic molecules interact non-covalently with the CD cavity to form the so-called inclusion complexes,15 which are also highly water-soluble. Moreover, α-CDs can be considered as alimentary fibre and contributes to reducing post-prandial glycaemic responses.16
Our research group has previously studied the use of CDs to mobilize cholesterol in Niemann Pick type C disease17,18 and the complexation process of several fatty acids and compounds with CDs recently,19–22 which have latterly been tested in a food model.23 Food models are considered an easy way to evaluate the behaviour of different molecules without interference from other metabolites.24 This information caught the attention of Feiraco® and Clesa®, two brands belonging to CLUN (Cooperativas lácteas unidas), which has been developed in a natural way by feeding cows with milk containing less saturated fats and less cholesterol. With a higher content of omega-3 fatty acids and conjugated linoleic acid, enriched in selenium25 and with less environmental impact due to the fact that the diet that these cows receive reduces enteric methane emissions.26 These enriched products were recently tested in a clinical trial in consumers with metabolic syndrome.27 This milk is as such a finished product that is marketed under the UNICLA brand (Table 1) and will be used to create novel functional foods based on CD technology. Different products could need an approach to mask the presence of a higher omega-3 concentration or to remove extra cholesterol, such as yogurts or milkshakes. Several authors have studied the capacity of CDs to remove cholesterol from dairy products for consumers advised by professionals to reduce their intake or to fortify milk with FAs.28,29 The present study uses the above to evaluate the stability and properties of several dairy food products (i) fortified with EPA + DHA from fish oil with CDs, and (ii) the ability of CDs to remove the cholesterol from milk. In addition, the bioaccessibility of EPA and DHA was evaluated30 to determine the capacity of both nutrients to be absorbed by the intestine.
Table 1 UNICLA composition (according to ref. 42 and internal measurements)
|
Comparative composition |
|
Normal |
UNICLA |
Differences |
% |
|
g per 100 g |
% Total FA |
g per 100 g |
% Total FA |
|
|
Fats |
3.6 |
100.00 |
3.60 |
100.00 |
|
|
Which are: |
|
|
|
|
|
|
Saturated |
2.52 |
70% |
2.09 |
58.00% |
−12.00 |
−17.14% |
Unsaturated |
1.08 |
30% |
1.51 |
42.00% |
12.00 |
40.00% |
Mono- |
0.90 |
25% |
1.24 |
34.50% |
9.50 |
38.00% |
Poly- |
0.18 |
5.00% |
0.27 |
7.50% |
2.50 |
50.00% |
Omega 3 |
0.014 |
0.40% |
0.06 |
1.60% |
1.20 |
300.00% |
Omega 6 |
0.058 |
1.60% |
0.13 |
3.50% |
1.90 |
118.75% |
CLA |
0.025 |
0.70% |
0.07 |
2.00% |
1.30 |
185.71% |
Omega 6/omega 3 |
4 |
|
2.19 |
|
−1.81 |
−45.31% |
Cholesterol (mg per 100 g) |
12 |
|
10 |
|
−2.00 |
−16.67% |
Selenium (μg per 100 g) |
2 |
|
5.00 |
|
3.00 |
250.00% |
Bearing in mind the above, several objectives were proposed: (1) to study the elimination of cholesterol from Feiraco-UNICLA® products using β-CD. (2) To evaluate the best CD combination to complex fish oil in food models. (3) To study the best formulation for adding fish oil to different Feiraco® and Clesa® products, creating novel fortified products with a high level of alimentary fibre. (4) To determine the organoleptic properties and possible consumer acceptance by means of a sensory panel. (5) To confirm the bioaccessibility of EPA + DHA in the final products.
2. Materials and methods
2.1 Materials
Cold extracted (Biomega Natural Nutrients), with the quantity of EPA and DHA indicated, and all milk products and derivatives were kindly supplied by Feiraco S.L., and used as received. The Free Fatty Acid Quantitation Kit was purchased from Sigma Aldrich (Madrid, Spain). The colorimetric cholesterol test was purchased from Boehringer Mannheim/r-biopharm (Roche). The honey (mil flores-Hacendado) was purchased from a local supermarket. The α-CD (alimentary grade) was purchased from Shanghai Soyoung Biotechnology (China), β-, γ-CD (alimentary grade), citric acid, vanillin, α-tocopherol, soy lecithin, ascorbyl palmitate, maltodextrins, remaining additives, reactants (alimentary grade), and the materials for sensory tests were purchased from Proquilab S.L. (Murcia, Spain).
2.2 Experimental procedures
2.2.1 Cholesterol elimination.
Milk samples containing 0, 1, or 1.5% of β-CD were incubated for 15 min at 20 °C while stirring at 500 rpm. Then, they were centrifuged at 6000g (15 min and 20 °C) and the supernatant was measured using the colorimetric cholesterol test according to specifications (Sigma-Aldrich) at 405 nm using a Jasco V-630 spectrophotometer (with Thorlabs cuvettes CV10Q1400).
2.2.2 Food model preparation.
“Milk-similar food model” samples were developed based on the pH value.24 In order to eliminate any chemical interactions between the samples and the food ingredients, an appropriate quantity of citric acid was used to create a buffer sodium citrate, 0.1 M pH = 6.51. All samples and materials were sterilized by autoclave. The initial and final concentrations of the samples were checked to prevent degradation.
2.2.3 Preparation of Feiraco® and Clesa® products based on our formulations.
The Feiraco® and Clesa® products (milk, yogurt, milkshakes, and creams) were opened in sterile conditions before adding the reactants (previously sterilized) in the appropriate order (ESI Fig. 1†). First, ascorbyl palmitate (E-304) and α-tocopherol (E-307) were added to the fish oil and vanillin to prevent lipid oxidation. The addition of soy lecithin and CDs created a powder. The addition of honey and maltodextrins increased the viscosity, density, and stability. Finally, the addition of the “Milk-similar food model” (0.1 M phosphate citrate at pH 6.51) solubilized (15 min mixing) the solution before adding it to the Feiraco® or Clesa® product.
2.2.4 Time course measurement: pH and thermal stability.
The pH was measured using a Crison pH-meter GLP 21+. The food samples were heated using a controlled temperature hot-plate.
2.2.5 Sensory test.
To evaluate the organoleptic profile, a sensory session was performed in the tasting room of the Food Technology Department, of the Faculty of Veterinary Medicine (University of Murcia), with individualized cabins using the published method.31,32
A group of 22 ± 5 random individuals smelled and tasted the different samples of different products or formulations and were asked about them, indicating the greater or lesser intensity or opinion of the odour intensity, among other questions (see ESI 1†) and their opinion. The values ranged from 0 (no) to 10 (appropriate).
At a short distance between samples, fish odour had an intense aromatic profile and the commercial product a characteristic flavour, which would have saturated the olfactory or gustatory receptors of the panellists, resulting in the same intensity being recorded for the different concentrations. To avoid this, coffee and cookies were offered between the different samples.
2.2.6
In vitro digestion and bioaccessibility.
The samples were subjected to the in vitro digestion model to assess the behaviour of the complexes.33,34 The protocol was the same as that described by Ilyasoglu (2014) with a few modifications: First, samples were prepared in saline at pH = 3 with pepsin-HCl to simulate the gastric phase before incubating in a shaking water bath at 37 °C for 1 h. The incubation was stopped by increasing the pH to 6.9. In the subsequent intestinal phase, the pH was adjusted to 6.9, and a pancreatin–bile extract–lipase mixture was added before incubating at 37 °C for 2 h. Samples were centrifuged at 16000 gx (4 °C and 35 min), and the supernatant was analysed using the Free Fatty Acid Quantitation Kit according to specifications at 570 nm in 96 well plates after 24 h at 25 °C in a Synergy HT plate reader (Bio-Tek Instruments, Winooski).
2.2.7 Data analysis.
The experiments were carried out in triplicate. Elemental statistics (SD, mean) was done using Excel 2013. Other mathematical operations were carried out using wxMaxima software (version 12.04.0).
3. Results and discussion
3.1 Cholesterol elimination from UNICLA Feiraco® milk using β-CD
Although the main objective of the work was to develop fortified products, cholesterol-free dairy products may help people who have difficulty metabolizing cholesterol, thereby reducing their dietary intake of total cholesterol.5 A wide range of milk samples was selected: skim milk with lactose (DR), skim milk without lactose (DS), low-fat milk with lactose (SV), low-fat milk without lactose (SS), and whole milk (EA). To these 0, 1, or 1.5% of β-CD was added at 20 °C mixed at 500 rpm for 15 min and centrifuged before measuring the cholesterol remaining in the milk.
The results (Table 2) showed the ability of 1% of β-CD to reduce the cholesterol content of EA by 87% and that of SS and SV by 85%. No advantage was gained by using 1.5% β-CD. These data are very close to those obtained previously,35 suggesting the possible use of this protocol in milk products.
Table 2 Cholesterol reduction in several Feiraco® (%) using β-CD
Milk |
% of β-CD |
0 |
1 |
1.5 |
Value |
SD |
Value |
SD |
Value |
SD |
EA |
100.00 |
0.20 |
13.41 |
0.25 |
12.72 |
0.30 |
SV |
100.00 |
0.29 |
15.08 |
0.10 |
14.79 |
0.49 |
SS |
100.00 |
0.48 |
15.02 |
0.10 |
14.83 |
0.29 |
DR |
100.00 |
4.05 |
98.84 |
4.62 |
100.00 |
1.73 |
DS |
100.00 |
2.29 |
98.86 |
3.43 |
99.43 |
1.71 |
3.2 Evaluation of different CD formulations for the microencapsulation of ω−3 fatty acids from fish oil in a milk food model
Before determining the effect of the CDs on the food matrixes, different milk-similar food models were prepared to establish better conditions. Although the Recommended Daily Allowance (RDA) for EPA + DHA is 15% per 100 mL of product (35 mg approximately), some products on the market contain around 20% (50 mg), so the concentration of 20% was selected as the desired quantity for our commercial formulations. Therefore, 0.25 g of fish oil per 100 mL of food product was added to 5 mL with or without combinations of 10 mM α-, β-, and γ-CD and mixed vigorously. Of all the conditions tested, the combination of α-CD
:
β-CD provided the best result. In contrast, γ-CD alone or in combination presented heterogeneous solutions and an intense fish odour. In light of these findings, we focused the study on different α-CD
:
β-CD combinations, finding that 35 mM α-CD with 10 mM β-CD (4
:
1 proportion) provided a homogeneous solution and a considerable reduction of the fish odour.
However, 50 mL of Milk-similar food model with 35 mM α-CD and 10 mM β-CD (4
:
1 proportion) was not able to reduce the odour (although the sample was homogeneous). Dimethylamine (DMA) is responsible for fish odour,36,37 and so became the natural target for the following steps. Two strategies were proposed: (i) forming ammonium salts, which are more stable, reducing the pH (e.g., by adding citric acid), (ii) adding different fragrances and coadjutants such as vanilla (using vanillin) or gum guar in combination with CDs.
The following were added to the sample consisting of 20% (EPA + DHA) and 35 mM α-CD with 10 mM β-CD (4:1): (i) vanillin (0.32, 0.72, or 1.44%), (ii) 0.5% citric acid, (iii) 1% gum guar and (iv) 1% maltodextrins, alone or combined between them. Vanillin imparted a very pale-yellow colour to the formulation, which was noted in the sensory analysis. Only vanillin was able to reduce the fish odour, with a concentration of 1.44% performing best. This formulation (20% EPA + DHA from fish oil, 35 mM α-CD with 10 mM β-CD and 1.44% vanillin) in the Milk-similar food model remained stable for 20 days at 4 °C, and also kept its homogeneous appearance (Fig. 1), although the microbial CFU's were not evaluated. The panellists in the sensory analysis considered this the best formulation in terms of aroma and appearance.
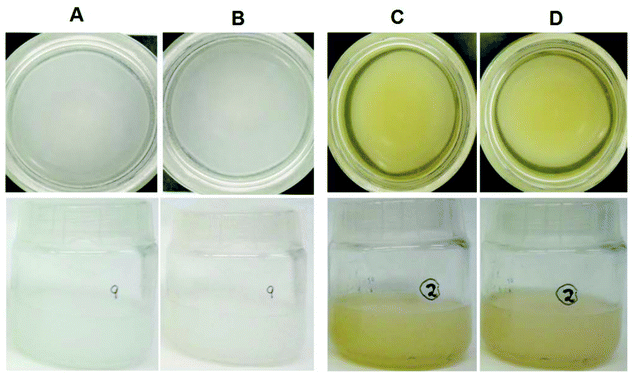 |
| Fig. 1 Appearance of the 20% of the RDA (EPA + DHA) from fish oil with 35 mM α-CD and 10 mM β-CD in a Milk-similar food model (pH 6.51) with (A) and without (C) 1.44% vanillin at day 0 (A and C) and 20 days (B and D) at 4 °C. | |
3.3 Application and perfection of the above formulation in CLUN (products: UNICLA® milk, Feiraco® and Clesa® Yogurt, and Feiraco® milkshake
3.3.1 UNICLA® milk.
The following milk samples were used: skim milk with lactose (DR), skim milk without lactose (DS), low-fat milk with lactose (SV), low-fat milk without lactose (SS), and whole milk (EA). The proportions established in the previous section were maintained but the reagents were added in a different order at 20 °C to make the emulsion more easily.38 Neither by adding CDs or fish oil was an acceptable appearance obtained, and the bad odour remained, so a previous emulsion of CDs and fish oil (with/without vanillin) in the minimum quantity of Milk-similar food model (0.1 M sodium phosphate pH 6.51) was achieved and added to the milk slowly at 20 °C and mixed.
The sensory analysis of the five milk derivatives without vanillin showed that only DS and DR presented a slight fish odour (2/10 being 10 the maximum odour), while SV (1/10), SS (0.5/10), and EA (0/10) did not. By adding 1% vanillin, the fish odour in DS, DR, SV, and SS was 0.5/10. Both formulations in the five milk samples presented a similar appearance to the control, and all the volunteers said they would drink all the milk derivatives prepared without any problem. Similar results were found by increasing the EDA + DHA to 40% (Fig. 2).
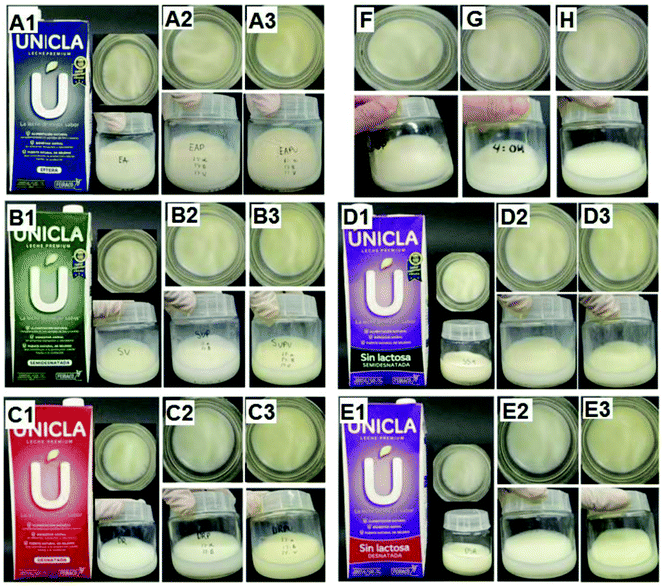 |
| Fig. 2 Appearance of different emulsions made with the 40% of the RDA (EPA + DHA) from fish oil with 10 mM α-CD and 9 mM β-CD in the five milk samples tested: whole milk (EA, letter A), low-fat milk with lactose (SV, letter B), skim milk with lactose (DR, letter C), low-fat milk without lactose (SS, letter D), and skim milk without lactose (DS, letter E) with (A2–D2) or without (A3–D3) 1% vanillin. | |
In order to reduce the quantity of CD, the proportions of CDs were varied, and once again, it was found that 10 mM α-CD with 9 mM of β-CD added to the milk samples in the same way was enough to obtain an appearance similar to that of the first formulation although sensory analysis concerning the fish odour was slightly worse DS (2/10), DR (2.5/10), SV (2/10), SS (1.5/10), and EA (0/10) than the previous formulation, although 80% of the participants expressed they would drink them. With 1% vanillin, the differences disappeared, and so this last formulation was selected as the most effective. All samples were stable for 4 days at 4 °C.
Even without vanillin, EA did not present a problem with odour, perhaps because it was reduced by the high level of fat. It is possible that using lecithin would have the same effect.39
3.3.2 Feiraco® milkshake and Feiraco® and Clesa® yogurts.
The above procedure established for milk was followed in order to fortify milkshakes and yogurts. First, an emulsion was made with both compounds (20% EPA + DHA from fish oil and 10 mM α-CD with 9 mM β-CD but without vanillin), by adding the minimum quantity of Milk-similar food model (0.1 M sodium phosphate pH 6.51). Then, it was added to different yogurts (Natural, YN; skim sugary natural, YNR) and milkshakes.
In none of the products did the appearance differ from that of the control products and neither did the sensory analysis detect any fish odour (0/10) (Fig. 3), and the volunteers also expressed they would drink all the milk products prepared without problems. All the samples were stable for 20 days at 4 °C. Even natural products did not present a fish odour, perhaps because of the density and viscosity of the products in combination with gum guar (E-412) and pectins (E-440) of the yogurt or milkshake helped encapsulation and stabilization.
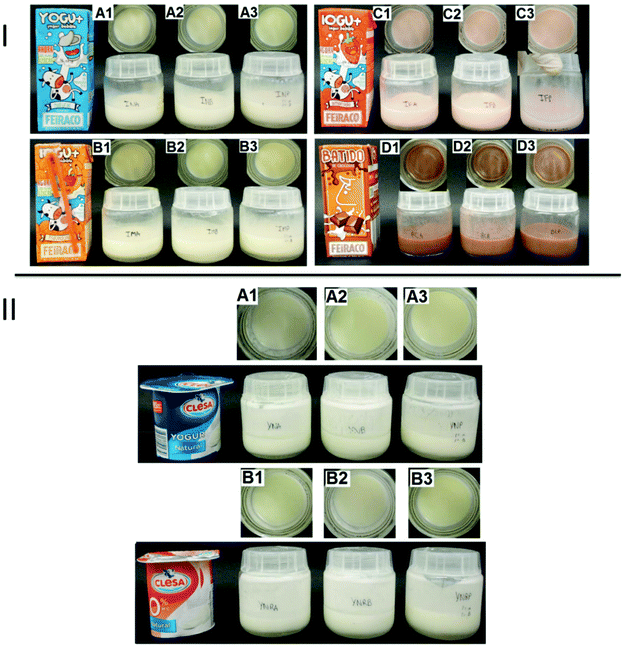 |
| Fig. 3 Different emulsion appearances of the 40% of the RDA (EPA + DHA) from fish oil with 10 mM α-CD and 9 mM β-CD in the product tested. I-A natural milkshake, I-B fruit salad milkshake, I-C strawberry milkshake, I-D chocolate milkshake whole milk, II-A natural yogurt, and II-B 0% natural Yogurt; control (A1–D1), 20% EPA + DHA from fish oil (A2–D2) and the 40% of the RDA (EPA + DHA) from fish oil plus 1% vanillin (A3–D3). | |
3.4 Development, stability, and use of the final formulation
The previous section demonstrated how we achieved a good formulation and how it was administrated on a low scale; however, a better way of administration is desirable if the process is to be scaled up for use in the food industry. In commercial products, the formulations contain 20% of the RDA for (EDA + DHA); however, to improve the results but with the minimum uptake of products, we established a target of 50% of the RDA in the total. For that reason, five new ingredients were added to the formulation: ascorbyl palmitate (E-304), α-tocopherol (E-307), soy lecithin (E-322), honey, and maltodextrins (E-1400). The preparation process is summarized in ESI Fig. 1† and the quantities of each reactant in ESI methods (1–4)† for each product that had passed several sensory tests successfully. Firstly, ascorbyl palmitate (E-304) and α-tocopherol (E-307) were added to the fish oil and also vanillin to prevent lipid oxidation. The addition of soy lecithin and CDs created a powder. The addition of honey and maltodextrins increased the viscosity, density, and stability. The new product remains stable for 3 months at 4 °C. Finally, the addition of the Milk-similar food model (0.1 M phosphate citrate at pH 6.51) mixing for 15 min solubilized the previous aggregation and the solution remained stable for 1.5 months at 4 °C. The sensory panel generally approved and liked the products (8/10).
The pH of the resultant solutions was very close to the control, and no relevant changes were observed over 20 days (ESI Table 1†), suggesting that the addition of this formulation did not affect the initial pH.23 The final milk derivatives did not produce any sedimentation, and even a thermal stability test (20, 50, and 75 °C for 2 min) did not lead to sedimentation (ESI Fig. 2–6†), although the samples heated at 75 °C presented a slight fish odour. The CFU's quantity was not evaluated. The complete sensory analysis results can be consulted in ESI 1.†
3.5
In vitro bioaccessibility of the omega-3 fatty acids in the final products
In order to check the bioaccessibility of the fatty acids in the final formulations, an in vitro digestion23,40 was carried out. This parameter is crucial to measure the stability of the fatty acids and the proportion that will be absorbed in the intestine. All the products were prepared in an in vitro stomach and intestine, after which the sample was centrifuged, and the quantity of fatty acids was measured using a colorimetric kit. The average value for milk was 75.4 ± 2.6% (ESI Fig. 7† and Table 3), which is very close to the value of 73.6 ± 1.70% obtained after an analysis of commercial omega-3 milk samples and higher than the value reported,30,34 using the same in vitro strategy. These data suggest that UNICLA® could be used as an interesting starting material for the development of novel functional foods with higher EPA + DHA quantity.
Table 3 Fatty acid concentration (g per 100 mL) obtained for each food product fortified with our formulations, and the bioaccessibility for each product (normalized using the control product without fortification)
Product |
FA (g per 100 mL) |
Total bioaccessibility (%) |
Fish oil bioaccessibility (%) |
Not digested |
Digested |
Value |
SD |
Value |
SD |
EA |
3.80 |
0.19 |
3.30 |
0.17 |
86.84 |
77.65 |
SV |
2.30 |
0.12 |
1.95 |
0.10 |
84.78 |
75.81 |
SS |
2.30 |
0.12 |
1.95 |
0.10 |
84.78 |
75.81 |
DR |
0.55 |
0.03 |
0.46 |
0.02 |
83.64 |
74.78 |
DS |
0.55 |
0.03 |
0.45 |
0.02 |
81.82 |
73.16 |
BC |
1.04 |
0.05 |
0.85 |
0.04 |
81.73 |
73.08 |
IN |
1.17 |
0.06 |
0.90 |
0.05 |
76.92 |
68.78 |
IF |
1.42 |
0.07 |
0.85 |
0.04 |
59.86 |
53.52 |
IM |
1.36 |
0.07 |
0.90 |
0.05 |
66.18 |
59.17 |
YN |
3.50 |
0.18 |
2.90 |
0.15 |
82.86 |
74.08 |
YNR |
0.62 |
0.03 |
0.51 |
0.03 |
82.26 |
73.55 |
YV |
2.50 |
0.13 |
2.20 |
0.11 |
88.00 |
78.68 |
YF |
2.50 |
0.13 |
2.23 |
0.11 |
89.20 |
79.76 |
YG |
2.45 |
0.12 |
2.15 |
0.11 |
87.76 |
78.46 |
CBO |
3.40 |
0.17 |
2.75 |
0.14 |
80.88 |
72.32 |
CCNN |
1.42 |
0.07 |
1.10 |
0.06 |
77.46 |
69.26 |
The high bioaccessibility values obtained suggest the efficient intestinal absorption of EPA and DHA, even at higher EPA + DHA levels. Although the bioaccessibility of EPA and DHA is high,41 a few doses of such functional foods would provide the desired quantity of bioactive compounds to the blood because of their high quantity. These bioaccessibility values demonstrate the efficient release of omega-3 fatty acids from our formulation and suggest good absorption in the intestine.
4. Conclusions
In this study, CDs were used to create functional foods in several ways. First, β-CD at 1% was able to reduce the concentration of cholesterol in Feiraco-UNICLA® milk samples by around 87–85%, a value very close to the values reported by other authors. Secondly, fortified omega-3 products from fish oil were formulated with α- and β-CD acting as carriers. Using food models, an initial formulation was developed, which was optimized for each product. Some additives were needed to reduce the odour of fish oil and improve its appearance, in our case, vanillin and α-tocopherol. It was possible to add around 50% of RDA of (EPA + DHA), with a high proportion of dietary fibre and emulate the organoleptic properties of commercial omega-3 products; indeed, 80% of the sensory panel was satisfied with the formulation. The final product was stable at different temperatures, and no variations in pH were found. Only when the milk formulation was heated at 75 °C was there a slight odour of fish. Generally, the bioaccessibility of the fatty acids added to the milk was around 74%, which is very close to the bioaccessibility of commercial omega-3 products. These results as a whole demonstrate the potential of CDs for adding bioactive compounds to food products and satisfy the aid of Feiraco® and Clesa® to develop improved products for human consumption based on UNICLA® fortification.
Author contributions
Conceptualization, A. M., I. M.-L., I. C., F. J. V.-S., F. G.-C. and J. M. L.-N.; methodology, B. G.-F., A. M., F. G.-C. and J. M. L.-N.; validation, B. G.-F., A. M., S. N.-O., F. G.-C. and J. M. L.-N.; formal analysis, B. G.-F., A. M. and J. M. L.-N.; investigation, B. G.-F.; resources, I. M.-L., F. G. C. and J. M. L.-N.; data curation, S. N.-O. and J. M. L.-N.; writing—original draft preparation, B. G.-F. and A. M.; writing—review and editing, B. G.-F., A. M., S. N.-O., I. M.-L., F. G.-C. and J. M. L.-N.; visualization, B. G.-F. and S. N.-O.; supervision, A. M. and J. M. L.-N.; project administration, I. M.-L. and J. M. L.-N.; funding acquisition, I. M.-L., F. G.-C. and J. M. L.-N. All authors have read and agreed to the published version of the manuscript.
Conflicts of interest
Ismael Martínez-Lede is member of “Feiraco Lacteos”. The funders had no role in the design of the study, in the collection, analyses, or interpretation of data; in the writing of the manuscript, or in the decision to publish the results.
Acknowledgements
This research was funded by the Spanish Ministry of Economy and Competitiveness (MEC-FEDER, Spain, project AGL2017-86526, by CDTI project DISMETASIN, ITC-20151251) and by the “Programa de Ayudas a Grupos de Excelencia de la Región de Murcia, Fundación Séneca, Agencia de Ciencia y Tecnología de la Región de Murcia (Spain)” (Project 19893/GERM/15). This work is the result of a predoctoral contract for the training of research staff (for Silvia Navarro-Orcajada, number 21269/FPI/19) and also an aid to postdoctoral training and improvement abroad (for Adrián Matencio, number 21229/PD/19) both financed by the Fundación Séneca (Región de Murcia, Spain).
References
- J. Buttriss, Int. J. Dairy Technol., 1997, 50, 21–27 CrossRef.
- B. Pourrajab, S. Fatahi, A. Dehnad, H. Kord Varkaneh and F. Shidfar, Nutr. Metab. Cardiovasc. Dis., 2020, 30, 11–22 CrossRef CAS PubMed.
- B. Pourrajab, N. Naderi, L. Janani, V. Mofid, M. Hajahmadi, A. Dehnad and F. Shidfar, Food Funct., 2020, 11, 10000–10010 RSC.
- A. Boudreau and J. Arul, J. Dairy Sci., 1993, 76, 1772–1781 CrossRef CAS.
- B. A. Kottke, A. A. Pineda, M. T. Case, A. M. Orsuzar and K. A. Brzys, J. Clin. Apher., 1988, 4, 35–46 CrossRef CAS PubMed.
- David S. Siscovick, Thomas A. Barringer, A. M. Fretts, J. H. Y. Wu, A. H. Lichtenstein, R. B. Costello, P. M. Kris-Etherton, T. A. Jacobson, M. B. Engler, H. M. Alger, L. J. Appel and D. Mozaffarian, Circulation, 2017, 135, e867–e884 CrossRef CAS PubMed.
- D. Richard, K. Kefi, U. Barbe, P. Bausero and F. Visioli, Pharmacol. Res., 2008, 57, 451–455 CrossRef CAS PubMed.
- C. Agostoni, J.-L. Bresson, S. Fairweather-Tait, A. Flynn, I. Golly, H. Korhonen, P. Lagiou, M. Løvik, R. Marchelli, A. Martin, B. Moseley, M. Neuhäuser-Berthold, H. Przyrembel, S. Salminen, Y. Sanz, S. (J. J.) Strain, S. Strobel, I. Tetens, D. Tomé, H. van Loveren and H. Verhagen, EFSA J., 2012, 10, 2815 Search PubMed.
- M. E. Stansby, J. Am. Oil Chem. Soc., 1971, 48, 820–823 CrossRef CAS.
- W. Klaypradit and Y.-W. Huang, LWT – Food Sci. Technol., 2008, 41, 1133–1139 CrossRef CAS.
- D. G. Noseda, H. G. Gentili, M. L. Nani, A. Nusblat, A. Tiedtke, J. Florin-Christensen and C. B. Nudel, Appl. Microbiol. Biotechnol., 2007, 75, 515–520 CrossRef CAS PubMed.
- A. Matencio, S. Navarro-Orcajada, F. García-Carmona and J. M. López-Nicolás, Trends Food Sci. Technol., 2020, 104, 132–143 CrossRef CAS.
- S. V. Kurkov and T. Loftsson, Int. J. Pharm., 2013, 453, 167–180 CrossRef CAS PubMed.
- P. Jansook, N. Ogawa and T. Loftsson, Int. J. Pharm., 2018, 535, 272–284 CrossRef CAS PubMed.
- S. Navarro-Orcajada, A. Matencio, C. Vicente-Herrero, F. García-Carmona and J. M. López-Nicolás, Sci. Rep. Search PubMed.
- EFSA, EFSA J., 2012, 10, 2713 Search PubMed.
- A. Matencio, M. A. Alcaráz-Gómez, F. García-Carmona, B. Arias and J. M. López-Nicolás, J. Chromatogr. B, 2018, 1093–1094 Search PubMed 47–51.
- A. Matencio, S. Navarro-Orcajada, A. González-Ramón, F. García-Carmona and J. M. López-Nicolás, Int. J. Pharm., 2020, 584, 119440 CrossRef CAS PubMed.
- A. Matencio, C. J. G. Hernández-Gil, F. García-Carmona and J. M. López-Nicolás, Food Chem., 2017, 216, 289–295 CrossRef CAS PubMed.
- A. Matencio, F. García-Carmona and J. M. López-Nicolás, Chem. Phys. Lipids, 2017, 204, 57–64 CrossRef CAS PubMed.
- A. Matencio, M. J. Bermejo-Gimeno, F. García-Carmona and J. M. López-Nicolás, Phytochem. Anal., 2017, 28, 151–158 CrossRef CAS PubMed.
- A. Matencio, F. García-Carmona and J. M. López-Nicolás, Food Chem., 2017, 232, 177–184 CrossRef CAS PubMed.
- A. Matencio, S. Navarro-Orcajada, I. Conesa, I. Muñoz-Sánchez, L. Laveda-Cano, D. Cano-Yelo, F. García-Carmona and J. M. López-Nicolás, Food Hydrocolloids, 2020, 98, 105250 CrossRef.
- A. Babazadeh, B. Ghanbarzadeh and H. Hamishehkar, J. Funct. Foods, 2016, 26, 167–175 CrossRef CAS.
- Ó. Muñiz-Naveiro, R. Domínguez-González, A. Bermejo-Barrera, J. A. Cocho de Juan, J. M. Fraga Bermúdez, A. Goris Pereiras, A. López Santamariña, I. Martínez Lede, J. Valledor Puente, L. Fernández-Couto Gómez and P. Bermejo-Barrera, J. Agric. Food Chem., 2005, 53, 9817–9822 CrossRef PubMed.
- L. Roibás, I. Martínez, A. Goris, R. Barreiro and A. Hospido, Sci. Total Environ., 2016, 566–567 Search PubMed 685–697.
- M. R. Pérez Fernández, I. Martínez Lede, J. M. Failde Garrido, S. Varela Rodríguez, N. Calvo Ayuso and A. J. del Álamo Alonso, Nutr. Hosp., 2021, 38(5), 983–992 Search PubMed.
- L. Alonso, P. F. Fox, M. V. Calvo and J. Fontecha, Molecules, 2018, 23, 1789 CrossRef PubMed.
- H.-S. Na, J.-N. Kim, J.-M. Kim and K.-Y. Lee, Biotechnol. Bioprocess Eng., 2011, 16, 1077–1082 CrossRef CAS.
- H. Ilyasoglu and S. N. El, LWT – Food Sci. Technol., 2014, 56, 461–468 CrossRef CAS.
- C. Abril-Sánchez, A. Matencio, S. Navarro-Orcajada, F. García-Carmona and J. M. López-Nicolás, Chem. Phys. Lipids, 2019, 219, 72–78 CrossRef PubMed.
- R. L. Chitwood, R. M. Pangborn and W. Jennings, Food Chem., 1983, 11, 201–216 CrossRef CAS.
- H. Ilyasoglu and S. N. El, LWT – Food Sci. Technol., 2014, 56, 461–468 CrossRef CAS.
- H. N. Bhagavan, R. K. Chopra, N. E. Craft, C. Chitchumroonchokchai and M. L. Failla, Int. J. Pharm., 2007, 333, 112–117 CrossRef CAS PubMed.
- A. M. Maskooki, S. H. R. Beheshti, S. Valibeigi and J. Feizi, Int. J. Food Sci., 2013, 2013, e215305 Search PubMed.
- W. J. Dyer, F. E. Dyek and J. M. Snow, J. Biol. Board Can., 1952, 8c, 309–313 CrossRef.
- T. H. Wu and P. J. Bechtel, J. Aquat. Food Prod. Technol., 2008, 17, 27–38 CrossRef CAS.
- A. Ogawa and H. Cho, J. Colloid Interface Sci., 2015, 449, 198–204 CrossRef CAS PubMed.
-
S. E. Peters and C. H. Brain, Micro/Nanoencapsulation of Active Food Ingredients, American Chemical Society, 2009, vol. 1007, pp. 183–197 Search PubMed.
- A. Matencio, S. Hernández-García, F. García-Carmona and J. M. López-Nicolás, Polymers, 2019, 11(5), 801 CrossRef CAS PubMed.
- N. Domoto, M. E. Koenen, R. Havenaar, A. Mikajiri and B.-S. Chu, Food Sci. Nutr., 2013, 1, 409–415 CrossRef CAS PubMed.
- Leche Únicla Entera | Leche Premium 100% natural | Leche Únicla, https://lecheunicla.com/leche-unicla-entera/, (accessed December 16, 2021).
Footnotes |
† Electronic supplementary information (ESI) available. See DOI: https://doi.org/10.1039/d2fo00578f |
‡ These authors contributed equally. |
§ Current situation: Independent researcher. |
|
This journal is © The Royal Society of Chemistry 2022 |
Click here to see how this site uses Cookies. View our privacy policy here.