DOI:
10.1039/D1FO02583J
(Paper)
Food Funct., 2022,
13, 398-410
γ-Tocotrienol induced the proliferation and differentiation of MC3T3-E1 cells through the stimulation of the Wnt/β-catenin signaling pathway
Received
8th August 2021
, Accepted 23rd November 2021
First published on 15th December 2021
Abstract
γ-Tocotrienol (γ-T3), an isoprenoid phytochemical, has shown the promotion of osteoblast proliferation and differentiation in our previous study. In this study, its underlying mechanism was investigated through regulating the Wnt/β-catenin signaling pathway in MC3T3-E1 cells. Comparative experiment results showed that γ-T3, not α-tocopherol (α-TOC) increased more significantly the viability and differentiation in MC3T3-E1 cells. After that, the cells were incubated with 10 mM LiCl, or 4 μM γ-T3 with or without 1 μM XAV-939. γ-T3 at 4 μM stimulated the Wnt/β-catenin signaling pathway by increasing the expression and nuclear accumulation of β-catenin, and the expressions of their downstream factors, such as cyclin-D1, c-Myc, BMP2 and BMP-4 in MC3T3-E1 cells. γ-T3 not only upregulated the viability, induced G0/G1 to the S phase, and promoted the expressions of PCNA (Proliferating Cell Nuclear Antigen) and Ki-67, but also increased ALP activity and the expressions of ON, OPN and OCN. Moreover, the effects of γ-T3 on the MC3T3-E1 cells resembled the actions of LiCl, an activator of the Wnt/β-catenin signaling pathway. Notably, all these effects of γ-T3 on the MC3T3-E1 cells were completely blocked by the Wnt/β-catenin signaling pathway inhibitor XAV-939. Our data demonstrated that γ-T3 can target β-catenin to enhance the Wnt/β-catenin signaling pathway, which led to increased expressions of the downstream cell proliferation and cell cycle-associated (cyclin D1 and c-myc), and cell differentiation-associated (BMP-2 and BMP-4) target genes, and ultimately promoted MC3T3-E1 cell proliferation and differentiation. Therefore, γ-T3 may be a potential agent to prevent and reverse osteoporosis due to its safety and powerful abilities of osteogenesis.
1. Introduction
Osteoporosis is a disease characterized by bone mass reduction due to the negative balance between osteoblast-induced osteogenesis and osteoclast-induced bone resorption, which can destroy the bone tissue microstructure, increase bone fragility and easily fracture.1 Osteoporosis is a worldwide health problem, and a systemic skeletal metabolic disorder with high morbidity and mortality and considerable social costs,2–4 making its prevention and treatment an urgent need. Currently, there are many drugs for the treatment of osteoporosis; for example, odanacatib, strontium ranelate, bisphosphonates, denosumab, raloxifene and calcitonin improve osteoporosis by inhibiting bone resorption, and teriparatide and anti-sclerostin antibodies are beneficial for bone formation.5 However, these drugs all have disadvantages such as poor efficacy, severe side effects and a high price.6,7 Thus, developing cost-effective and safe drugs or phytochemical alternatives is of great significance for the prevention and improvement of osteoporosis.
Recent studies indicated that vitamin E maintains bone mineral density in ovariectomized8 or orchidectomized9 rats, strengthens bone in rats given alcohol10 and has a positive impact on bone metabolism in hindlimb unloaded mature male rats.11 Supplementation of vitamin E has been confirmed to prevent bone loss and restore bone strength in aged mice.12 Furthermore, Mata-Granados et al. (2013)13 demonstrated that vitamin E increased bone mineral density in early postmenopausal women. These results suggest that vitamin E supplementation can be a potential alternative treatment for the prevention of osteoporosis.
Vitamin E has eight natural isoforms: α, β, γ, and δ isomers of tocotrienols (T3) and tocopherols (TOC),14 of which α-TOC is the most abundant form in the nature15 and human body.16 α-TOC was reported to encourage bone formation and shorten the retention period during the early stage of mouse anterior healing;17 however, it had a negative impact on cervical bone mineral density18 and on bone formation19 of postmenopausal women, making the use of α-TOC a controversial strategy for osteoporosis treatment. Compared to TOC, T3 has been shown to better prevent bone loss caused by estrogen deficiency,20–22 promote fracture healing in postmenopausal osteoporosis rats,23 and promote bone formation in rats with metabolic syndrome-induced osteoporosis24 and testosterone-deficient rats,25 and protect rats from bone damage induced by free radicals26 or nicotine,27 despite its low content. Recent in vitro comparative studies of α, β, γ and δ-T3 isomers and α-TOC demonstrated that γ- and δ-T3 showed to exert the most prominent bone anabolic effects by increasing osteoblast differentiation and enhancing the bone microstructure.28In vivo studies demonstrated that γ-T3 significantly improved the skeletal morphological parameters in normal male rats, which is more effective than other isoforms.29 γ-T3 can also effectively preserve bone calcium of adrenalectomized rats30 and reverse the nicotine-induced osteoporosis of male rats.31 Meanwhile, γ-T3 can also be used as an HMG-CoA reductase inhibitor to prevent bone loss caused by ovariectomy through the mevalonate pathway.32 Although these previous studies revealed that γ-T3 was a potential agent for preventing and treating osteoporosis, its underlying mechanism at the cellular level has not been fully elucidated.
The Wnt/β-catenin signaling pathway cascade controls different life phenomenon such as embryonic development, stem cell metabolism33 and human diseases,34 and its role in bone metabolism has been suggested in recent studies.35 The Wnt/β-catenin signaling pathway is composed of Wnt (Wingless and Int-1), low density lipoprotein-related protein receptor (LRP5/6), β-catenin, glycogen synthase kinase (especially GSK-3β) and other related proteins. When the signaling pathway is activated, Wnt binds to frizzled receptors and LRP5/6 co-receptors, causing the cytoplasmic phosphoprotein to dishevel. This further inhibits the phosphorylation of β-catenin by GSK-3β and accumulation of β-catenin in the cytoplasm which then translocates to the nucleus and regulates the expression of downstream genes.36In vitro and in vivo studies showed that Wnt/β-catenin signaling regulates the proliferation and differentiation of osteoblasts to enhance bone formation.37–40
Our previous in vitro studies have shown that γ-T3 can promote the proliferation and differentiation of MC3T3-E1 cells.41 However, whether this effect of γ-T3 is mediated by the Wnt/β-catenin signaling pathway remains unclear. The purpose of this research was (1) to compare the effects of γ-T3 and α-TOC on the proliferation and differentiation of MC3T3-E1 cells, (2) to examine the regulatory role of γ-T3 on the Wnt/β-catenin signaling pathway in the MC3T3-E1 cells, and (3) to clarify the correlation of γ-T3-induced proliferation and differentiation and the Wnt/β-catenin signaling pathway in the MC3T3-E1 cells.
2. Materials and methods
2.1 Reagents
γ-T3 (the purity is 99%) was purchased from Cayman Chemical Co. (Ann Arbor, MI, USA) and absolute ethanol was used to prepare the stock solution and stored at −20 °C. FBS (fetal bovine serum) and α-MEM (α-minimum essential medium) were obtained from Gibco life Technologies Co. (Carlsbad, CA, USA). Dimethyl sulfoxide, MTT (3-(4,5-dimethylthiazol-2-yl)-2,5-diphenyltetrazolium bromide) and DAPI (4′,6-diamidine-2′-phenylindole) were purchased from Sigma-Aldrich (St Louis, MO, USA). Trypsin was purchased from Amresco (Washington, USA) and sodium dodecyl sulfate polyacrylamide gel electrophoresis (SDS-PAGE) gel kit, bicinchoninic acid (BCA) protein assay kit and phenylmethanesulfonyl fluoride were obtained from Beijing Applygen Gene Technology Co., Ltd (Beijing, China). A nitrocellulose membrane was purchased from Pall Corporation (New York, USA). Phosphate buffered saline (PBS) was purchased from Solarbio Life Sciences (Beijing, China). Antibodies against p-β-catenin (#9561), c-Myc (#9402), and cyclin D1 (#2978) were obtained from Cell Signaling Technology, Inc. (MA, USA). Anti-GAPDH (sc-25778), anti-β-catenin (sc-7199), anti-Ki-67 (sc-7846), anti-PCNA (sc-7907), and anti-Lamin B1 (sc-374015) antibodies were purchased from Santa Cruz Biotechnology, Inc. (CA, USA). Goat anti-rabbit IgG (4030) was obtained from Southern Biotech (Birmingham, AL, USA). The FITC-conjugated secondary antibody (ZF-0311) was purchased from Beijing Zhongshang Jinqiao Biological Co., Ltd (Beijing, China). ELISA kits for OPN (osteopontin), ON (osteonectin), OCN (osteocalcin), BMP-2 (bone morphogenetic protein-2), and BMP-4 (bone morphogenetic protein-4) were obtained from Nanjing Jiancheng Bioengineering Institute (Nanjing, China).
2.2 Cell culture
MC3T3-E1 cells (Cell Bank of Chinese Academy of Sciences, Shanghai, China) were cultured in α-MEM supplemented with 10% FBS in a humidified incubator with 5% CO2 at 37 °C. When confluence reached 80%, cells were treated with various concentrations of γ-T3 in α-MEM medium containing 5% FBS. The medium was changed every 2 or 3 days.
2.3 Cell viability assay
Cells (5 × 103 cells per well) were seeded in a 96-well plate overnight and then incubated with different concentrations (0, 2, 4, 8, 16 or 32 μM) of γ-T3 or α-TOC, each for 2, 4 and 6 days. Then 20 μL of MTT (5 mg mL−1) was added to each well and the cells were incubated for 4 h at 37 °C. The medium was replaced with 150 μL of DMSO (dimethyl sulfoxide), and then the 96-well plate was shaken for 10 min. The absorbance was measured at 490 nm using a microplate reader (Bio-Tek Instruments, Inc., VT, USA). The viability of the control cells, which were not treated, was set as 100%. The cell viability of the treated group was calculated relative to the control group (absorbance in the experimental group/absorbance in the control group × 100%).
2.4 Alkaline phosphatase (ALP) activity assay
ALP activity was detected according to the procedure previously mentioned.41 Briefly, cells were seeded at a density of 1 × 105 cells per mL in a 6-well plate and incubated overnight, and then treated with γ-T3 or α-TOC at various concentrations (0, 2, 4, 8 or 16 μM) for 0, 3, 6 or 9 days. The ALP activity was measured using the ALP ELISA kit (Shanghai Langton Biotechnology Co., Ltd, Shanghai, China). Dexamethasone (Dex, 250 μM) and vitamin C (VC, 20 nM) were used as a positive control.42,43 ALP activity was expressed as the ratio of ALP concentration in the treated group to that in the control group.
2.5 Mineralization assay
Mineralization of the MC3T3-E1 cells was investigated by alizarin red staining according to our previous study.41 Briefly, cells (3 × 104 cells per well) in a six-well plate were treated for 21 days and the medium was replaced every 3 days. The formation of mineralized nodules was determined using an alizarin red kit (GenMed Scientifics Inc., USA) and observed using an optical microscope. The content of alizarin red of the treated groups was expressed as a percentage of the control group.
2.6 Quantitative real time PCR
The mRNA expression levels were investigated by a real time PCR method according to our previous study.41,44 Primers used were as follows: GAPDH forward 5′-CCCAGAAGACTGTGGATGG-3′ and GAPDH reverse 5′-GGATGCAGGGATGATGTTCT-3′ (81 bps); APC forward 5′-TGTGAGATGTATGGGCTTACTAAT-3′ and APC reverse 5′-TGCAATAACCTGCTGTAAGTC-3′ (189 bps); GSK3β forward 5′-GACTTTGGAAGTGCAAAGC-3′ and GSK3β reverse 5′-AGGAAATATTGGTTGTCCTAGC-3′ (177 bps); LRP5 forward 5′-AAGCCAAGGATTGTGCG-3′ and LRP5 reverse 5′-CCAGCATGTTGGAAGACTC-3′ (157 bps); and LRP6 forward 5′-CATGTGGTAGAGTTTGGCTTAG-3′ and LRP6 reverse 5′-GAGGACTGTCAAGGTCTTTCCATA-3′ (160 bps).
2.7 ELISA determination
Cells were seeded in 10 cm culture dishes at 4.2 × 104 cells per well for 24 h and then treated with reagents for 3 or 6 days, respectively. The concentrations of ON, OPN, OCN, BMP-2, and BMP-4 were respectively evaluated using ELISA kits (Nanjing Jiancheng Bioengineering Institute, Nanjing, China). The absorbance at 450 nm was measured using a microplate reader.
2.8 Western blot analysis
MC3T3-E1 cells were collected, lysed in RIPA lysis buffer (C1053, Applygen Technologies Inc., Beijing, China) with 0.2 mmol L−1 of phenylmethanesulfonyl fluoride (A1100, Applygen Technologies Inc., Beijing, China) and centrifuged at 12
000 rpm at 4 °C for 10 min. To investigate the nuclear localization of β-catenin, both nuclear and cytoplasmic proteins from the MC3T3-E1 cells were extracted using a Nuclear and Cytoplasmic Protein Extraction Kit (P1200, Applygen Technologies Inc., Beijing, China). The concentration of total protein in the supernatant was measured using a BCA assay kit. Then, the western blot experiment was performed as previously described.41 Briefly, 40–80 μg of protein was resolved on 10% polyacrylamide gels and transferred to a nitrocellulose membrane. After blocking in blocking buffer (1% BSA, 1% Tween 20 in 20 mM Tris-buffered saline (TBS), pH 7.6) for 30 min at 37 °C in a hybridization oven, the membrane was incubated with a primary antibody in the blocking buffer for 2 h at 37 °C. The membrane was washed 3 × 5 times with Tris-buffered saline Tween 20 (TBST), and then incubated with a secondary antibody at 37 °C for 1 h. The membrane was washed with TBST and TBS. Then the membrane was incubated with alkaline phosphatase until an appropriate signal level was obtained. The image of the nitrocellulose membranes was captured using the FluorChem Imaging System (BioTek Instruments, Inc., VT, USA) and analyzed using Quantity one software.
2.9 Immunofluorescence
Cells were seeded at a density of 105 cells per well in a 6-well plate with a slide in each well and cultured overnight. Then, the cells were treated with different concentrations of γ-T3 (0, 2, 4 or 8 μM) or LiCl (lithium chloride, 10 mM) for 48 h, respectively. The cells were rinsed twice with PBS and fixed with 4% paraformaldehyde for 20 min, permeabilized in 0.1% Triton X-100 for 3 min, and then incubated with 1% BSA (bovine serum albumin) for 30 min. The slides were incubated with a primary anti-β-catenin (1
:
100) antibody for 1.5 h, followed by incubation with a FITC-conjugated secondary antibody (1
:
50) for 1 h, and were then stained with 4,6-diamidine-2-phenylindole (DAPI, 15 μg mL−1) for 15 min. A Zeiss LSM710 microscope (Zeiss, Jena, Germany) was used to observe the subcellular localization.
2.10 Statistical analysis
The experimental data are expressed as the mean ± SD. Statistical analysis was analyzed by one-way analysis of variance (ANOVA) using SPSS16.0 for Windows. Differences were considered significant at P < 0.05. Each experimental measurement was performed at least three times independently.
3. Results and discussion
3.1 γ-T3 was more effective than α-TOC for promoting osteoblast proliferation and differentiation
Osteoporosis can be induced by physiological states (such as age and postmenopausal), poor diet, inactivity, being an alcoholic, and even certain medications (for example, hormone abuse), of which one of the most common causes is osteoblast dysfunction.45 Stimulating osteoblast function can promote bone formation, and increase bone mass and bone strength.46 Therefore, identifying phytochemicals with osteoblast-stimulating activity to improve bone mass is one of the most promising preventive and therapeutic approaches for osteoporosis.
MC3T3-E1 cells have the advantages of a stable growth and infinite passage, thus are widely used as a pre-osteogenic cell line for studying bone metabolic diseases.47 First, the effect of γ-T3 or α-TOC on the proliferation of the MC3T3-E1 cells was measured by the MTT assay and morphological observation. The results showed that the viability of MC3T3-E1 cells treated with lower concentrations of γ-T3 (4–8 μM, especially at 4 μM) was significantly higher compared with the blank or solvent control groups (P < 0.05). However, higher concentrations of γ-T3 (16–32 μM) significantly lowered the cell viability (P < 0.05) (Fig. 1A) and showed that γ-T3 of the above dose has cytotoxicity to MC3T3-E1 cells. This is consistent with the results of our own study41 and a study by others.48 Another study also showed that γ-T3 in the dose range of 0.01–1 μM was not cytotoxic on hFOB 1.19 human osteoblast cells.28 In contrast, α-TOC had no significant effect on the viability of MC3T3-E1 cells at all tested concentrations (P > 0.05). Additionally, ethanol solution as a solvent control (the final concentration in all cultures was ≤0.16%) exerts no cytotoxicity on the MC3T3-E1 cells. Similar results have been obtained by observing the cell morphology using an inverted microscope (data not shown).
 |
| Fig. 1 The effects of γ-T3 and α-TOC on the proliferation and differentiation of MC3T3-E1 cells. (A) The viability of the MC3T3-E1 cells treated with different concentrations of γ-T3 or α-TOC for the indicated time was measured by the MTT method. (B) ALP activity of the MC3T3-E1 cells treated with different concentrations of γ-T3 or α-TOC, respectively, for 3 days was assayed using ELISA kit methods. (C) The nodules of the MC3T3-E1 cells treated with α-TOC (4 μM), γ-T3 (4 μM), and the positive control (DEX + VC) for 3, 6 and 9 days were determined by Alizarin Red staining. (D) Image of the mineralized nodes in the MC3T3-E1 cells after different treatments for 21 days (×100). (a′) Control, (b′) γ-T3 (4 μM), (c′) α-TOC (4 μM), (d′) β-glycerol phosphate disodium (20 ng mL−1), (e′) γ-T3 (4 μM) + β-glycerol phosphate disodium (20 ng mL−1) and (f′) α-TOC (4 μM) + β-glycerol phosphate disodium (20 ng mL−1). (E) Quantification of the mineralized nodes was expressed as the relative ratio to the control group. Data represent the mean ± SD of three independent experiments. Values with different letters indicate significant differences (P < 0.05), whereas values with same letters indicate that the difference is not significant (P > 0.05). | |
Then, we compared the effect of γ-T3 and α-TOC on the differentiation of MC3T3-E1 cells. In the early stage of MC3T3-E1 cell differentiation, ALP activity increases gradually and local phosphate accumulates in the cell, which is the basis for mineralization. The ALP activity and matrix mineralization are indicators of osteoblast differentiation.29,49 Our in vitro study results suggested that the ALP activity in the MC3T3-E1 cells treated by different doses (2, 4, 8 and 16 μM) of γ-T3 for 72 h was increased in a dose-dependent manner (Fig. 1B). Moreover, treatments with 2 μM–16 μM γ-T3 significantly promoted the ALP activity in the MC3T3-E1 cells compared to those treated with the concentrations of α-TOC, indicating great potential of γ-T3 in enhancing osteoblast differentiation. A current study by Jolly et al.28 reported that treatment with 0.01 μM–1 μM γ-T3 for 7 d or more significantly promoted the ALP activity of hFOB 1.19 human osteoblast cells. The reason for the difference in the treatment dose of γ-T3 and initiation time of ALP activity enhancement may be associated with the cell numbers seeded, varieties of cell lines, cell viability, or laboratory conditions. The ALP activities of the cells treated with γ-T3 in the range from 4 μM to 16 μM showed no significant difference. Meanwhile, γ-T3 had the strongest proliferative effect on the MC3T3-E1 cells at 4 μM (Fig. 1A); thus, 4 μM γ-T3 was used in the follow-up experiments. Dex induced osteoblast differentiation through the activation of Wnt/β-catenin signaling-dependent Runx2 expression.42 VC mainly accelerates the differentiation of osteoblasts by hydroxylating the two amino acids, i.e., proline and lysine in the collagen of osteoblasts and secreting the Col I protein.43 We co-treated cells with Dex and VC as a positive control and compared the induction of γ-T3 (4 μM) on ALP activity with that of the positive control group. ALP activity in cells treated with 4 μM γ-T3 for 3, 6, and 9 d was significantly increased in comparison with α-TOC and the blank and the solvent controls (P < 0.05), and there was a similar effect in the positive control group (DEX-VC group) (Fig. 1C). We further compared the effects of α-TOC and γ-T3 on bone nodule formation in the MC3T3-E1 cells by alizarin red staining, and the results are presented in Fig. 1D and E. Compared with the blank control group, the mineralized nodules of the MC3T3-E1 cells treated with 4 μM α-TOC for 21 days were not significantly changed, and the relative content of alizarin red was 0.89 (P > 0.05), indicating that at this level, α-TOC did not promote mineralization in the MC3T3-E1 cells. However, the mineralization ability of the MC3T3-E1 cells was significantly increased after treatment with 4 μM γ-T3 or 20 ng mL−1 of β-glycerol phosphate disodium (positive control). β-Glycerol phosphate disodium also showed a synergistic effect with 4 μM γ-T3, but not with α-TOC. So, we verified the effectiveness of γ-T3 in stimulating MC3T3-E1 cell differentiation, a result consistent with our previous finding.41 In another study, annatto-derived T3 (AnT3) also enhanced osteoblast differentiation by promoting gene and protein expressions related to bone formation.50 AnT3 is a mixture of about ∼90% of δ-T3 and 10% γ-T3, thus the role of each individual component was not clearly defined.
3.2 γ-T3 stimulates the Wnt/β-catenin signaling pathway in MC3T3-E1 cells
The Wnt/β-catenin signaling pathway is closely related to the maintenance of bone homeostasis.51 Some chemicals can affect the concentration and localization of β-catenin, the core protein of the Wnt/β-catenin pathway, thus modulating the effect of the pathway.52–55In vivo studies indicated that AnT3 supplementation significantly increases the mRNA expressions of CTNNB1 genes (β-catenin) in testosterone-deficient rats when compared with the sham-operated group (P < 0.05).25 It is also possible that the effect of the AnT3 mixture on CTNNB1 genes was different from that of δ- or γ-T3 isomers. However, in vivo and in vitro studies of the two isomers on the Wnt/β-catenin signaling pathway have not been reported. LiCl is an activator of the Wnt/β-catenin signaling pathway by inhibiting the negative regulatory factor GSK-3β in the signaling pathway,56 and has been confirmed to significantly increase bone mineral density and bone mass in mice with osteoporosis.57 As shown in Fig. 2, treatment with LiCl for 48 h reduced GSK3β, LRP5 and LRP6 mRNA expressions, and increased the level of APC (Fig. 2A and B). γ-T3 had the same effect on GSK3β as LiCl. The expressions of APC and LRP6 were significantly increased (P < 0.05), but that of LRP5 was not observed after treatment with 2–8 μM γ-T3 for 48 h. Furthermore, total β-catenin protein level was significantly increased, and p-β-catenin was significantly decreased in the MC3T3-E1 cells treated with 4 μM γ-T3 for 48 h compared with the other five groups (P < 0.05) (Fig. 2C). The results of indirect immunofluorescence showed that γ-T3 promoted the translocation of β-catenin into the nucleus, which is comparable to LiCl. Our results indicated that γ-T3 could upregulate the Wnt/β-catenin signaling pathway by disrupting the β-catenin destruction complex via inhibition of GSK3β expression, allowing the stabilization and nuclear translocation of β-catenin.
 |
| Fig. 2 The effect of γ-T3 on the Wnt/β-catenin signaling pathway in MC3T3-E1 cells. (A and B) The expressions of APC, GSK3β, LRP5 and LRP6 in the MC3T3-E1 cells treated with 2–16 μM γ-T3 for 48 h were analyzed by real-time PCR. (C) The levels of β-catenin and p-β-catenin protein in the MC3T3-E1 cells treated with γ-T3 for 48 h were analyzed by the western blot method (M: marker, Blank: blank control and Con: ethanol control). (D) The effect of γ-T3 on the localization of β-catenin in the MC3T3-E1 cells treated with γ-T3 (4 μM) and LiCl (10 mM) for 48 h. Cells were fixed with 4% paraformaldehyde, and then incubated with the β-catenin antibody and FITC-labeled secondary antibody (top), followed by counterstaining with DAPI (middle). Images were collected using laser confocal microscopy (×100). The merger images (bottom) are the matched together of above two images. Data represent the mean ± SD of three independent experiments. Values with different letters indicate significant differences (P < 0.05), whereas values with same letters indicate that the difference is not significant (P > 0.05). | |
To further confirm the specificity of γ-T3 on the Wnt/β-catenin signaling pathway in osteoblasts, XAV-939 and LiCl were introduced and the MC3T3-E1 cells were treated in combination with 4 μM γ-T3. XAV-939 is a specific blocker of the Wnt/β-catenin signaling pathway,58,59 which can stabilize Axin (an inhibitor of the Wnt signalling pathway that interacts with β-catenin, GSK3β and APC) by inhibiting tankyrase, so as to selectively inhibit β-catenin-mediated transcription. The results of western blotting showed that the treatment with γ-T3 or LiCl for 72 h significantly inhibited the level of p-β-catenin phosphorylated by GSK3β, and increased the expression of total, cytoplasmic and nuclear β-catenin protein, but the effect was inhibited by the blocker XAV-939 (Fig. 3A–D) (P < 0.05). Taken together, 4 μM γ-T3 may enhance the Wnt/β-catenin signaling pathway by stimulating the accumulation and subsequent nuclear translocation of β-catenin. Thus, this pathway should also be considered in the potential mechanism of γ-T3 action in stimulating osteoblastic activity.
 |
| Fig. 3 The blocking effect of XAV-939 on the γ-T3-enhanced Wnt/β-catenin signaling pathway. Cells were treated individually or in combination with γ-T3 (4 μM), XAV-939 (1 μM) or LiCl (10 mM) for 72 h, and the protein expression levels of p-β-catenin protein (A), total β-catenin protein (B), cytoplasmic β-catenin protein (C), and nucleoplasmic β-catenin protein (D) were measured by western blotting. Data represent the mean ± SD of three independent experiments. Values with different letters indicate significant differences (P < 0.05), whereas values with same letters indicate that the difference is not significant (P > 0.05). | |
3.3 Blocking β-catenin abrogates γ-T3-induced proliferation of MC3T3-E1 cells
Bone is one of the few human tissues that have the ability of self-repair and regeneration.60 Increased number of osteoblasts requires local reactivation of signal cascades that are crucial for bone formation.61,62 As shown in Fig. 4A, the proliferation rate of MC3T3-E1 cells in 4 μM γ-T3 or 10 mM LiCl treatment group was significantly increased (P < 0.05), but significantly inhibited in the XAV-939 group (survival rates were about 75%) (P < 0.05) compared with the control group. There were no significant differences in the proliferation among the groups treated with 4 μM γ-T3 and 1 μM XAV-939, or 10 mM LiCl and 1 μM XAV-939 and in the group treated with 1 μM XAV-939 alone. Results of the inverted microscopic observation are shown in Fig. 4B. The morphology of the MC3T3-E1 cells treated with XAV-939 for 72 h remained unchanged, but the cell number decreased obviously compared with the control group. Treatment with 4 μM γ-T3 or 10 mM LiCl for 72 h increased the number and the adhesion between the MC3T3-E1 cells compared with the control cells. The numbers of MC3T3-E1 cells were slightly different between the groups treated with XAV-939 combined with γ-T3 or LiCl and the group treated with XAV-939 alone. Similarly, we also observed that XAV-939 blocked the γ-T3 or LiCl-induced increase in the cell proliferation markers, i.e., PCNA and Ki-67 protein expressions in the MC3T3-E1 cells (Fig. 4C). These results are consistent with that of the MTT assay and indicated that XAV-939 seriously abrogated the enhancing effect of γ-T3 or LiCl on the proliferation. Therefore, we concluded that blocking the Wnt/β-catenin signaling pathway can abrogate the γ-T3-induced proliferation effect of the MC3T3-E1 cells.
 |
| Fig. 4 The effects of XAV-939 on γ-T3-induced proliferation in MC3T3-E1 cells. The cells were treated with γ-T3 or LiCl alone or in combination with XAV-939 for 72 h. (A) The cell viability was measured by the MTT assay. (B) The morphology of the MC3T3-E1 cells was observed under an inverted microscope (×100). a. Control; b. γ-T3; c. XAV-939; d. XAV-939 + γ-T3; e. XAV-939 + LiCl; f. LiCl. (C) The levels of PCNA and Ki67 protein expressions were measured by the western blot method. (D) The levels of cyclin D1 and c-Myc protein expressions were measured by the western blot method. Results are expressed as the percentages of the control. Data are expressed as means ± SD of three independent experiments. Values with different letters indicate significant differences (P < 0.05), whereas values with same letters indicate that the difference is not significant (P > 0.05). | |
Increased β-catenin levels in the cytosol and its nuclear translocation could modulate the transcription of Wnt/β-catenin-targeted genes, such as cyclin D1 (cell cycle protein) and c-Myc, which play a stimulative role in osteoblastic proliferation.63–66 The expressions of the two proteins of MC3T3-E1 cells treated with γ-T3 or LiCl alone or combined with XAV9-39 for 72 h were further assessed. As shown in Fig. 4D, both 4 μM γ-T3 and 10 mM LiCl significantly increased cyclin D1 and c-Myc protein expressions compared to the control group (P < 0.05). However, the expressions of both proteins induced by γ-T3 or LiCl were markedly inhibited by XAV-939 (Fig. 4D), indicating that the promotional effects of γ-T3 on the cell proliferation regulators cyclin D1 and c-Myc were due to the stimulation of the Wnt/β-catenin signaling pathway. These results showed that γ-T3-induced cell proliferation could mediate through the Wnt/β-catenin signaling pathway by promoting the expressions of proliferation-related target genes.
3.4 Blocking β-catenin repressed the γ-T3-induced cell cycle changes of MC3T3-E1 cells
Cyclin D1 plays a critical role in cell cycle regulation, and the cyclin D1-CDK (cyclin-dependent kinase) complex promotes the progression of cell cycle into the S phase.67,68 c-Myc upregulates cyclin D1 expression and cyclin D1 plays a critical role in the regulation of G1-phase progression.69 Based on our previous results, we investigated whether γ-T3-induced cell cycle changes were also related to its regulation of the Wnt/β-catenin signaling pathway. So, we next carried out a flow cytometry analysis on the MC3T3-E1 cells treated with 4 μM γ-T3 or 10 mM LiCl alone or combined with XAV9-39 for 72 h. As shown in Table 1, the cell populations in the G0/G1 phase of the MC3T3-E1 cells treated with 1 μM XAV-939 for 72 h increased by 4.74%. The result indicated that XAV-939 could arrest the MC3T3-E1 cells at the G0/G1 phase through blocking the Wnt/β-catenin signaling pathway. The percentages of γ-T3 or LiCl-treated MC3T3-E1 cells in the S phase significantly increased, while that in the G0/G1 phase significantly decreased as compared with the control group (P < 0.05). XAV-939 in combination with γ-T3 or LiCl abrogated the regulatory effects by γ-T3 or LiCl on the cell cycle. The results indicated that γ-T3 could induce cell cycle progression from the G0/G1 phase to the S phase through enhancing the Wnt/β-catenin signaling pathway, and then promote cell proliferation.
Table 1 The effect of XAV-939 on γ-T3-induced changes in the cell cycle distribution in MC3T3-E1 cells
Groups |
Cell cycle distribution (%) |
G0/G1 |
S |
G2/M |
The results shown are the mean ± S.D. from three independent experiments. Values with different letters indicate significant differences (P < 0.05), whereas values with same letters indicate that the difference is not significant (P > 0.05). |
Control |
74.45 ± 2.01a |
3 ± 0.17a |
22.56 ± 1.97a |
γ-T3 |
68.64 ± 4.13b |
5.2 ± 0.99b |
26.15 ± 1.49b |
XAV-939 |
79.19 ± 1.34a |
1.81 ± 0.28c |
19 ± 2.06c |
XAV-939 + γ-T3 |
78.44 ± 1.10a |
2.43 ± 0.46a |
19.12 ± 1.48c |
XAV-939 + LiCl |
74.73 ± 2.45a |
2.16 ± 0.39c |
23.12 ± 1.04a |
LiCl |
66.02 ± 4.11b |
7.11 ± 1.33d |
26.87 ± 2.97d |
3.5 γ-T3 stimulates a differentiation-related protein expression in MC3T3-E1 cells via the Wnt/β-catenin signaling pathway
To verify the role of Wnt/β-catenin signaling in γ-T3-induced osteoblast differentiation, the ALP activity and the expressions of bone differentiation proteins (ON, OPN, OCN, BMP-2 and BMP-4) in the MC3T3-E1 cells treated with 4 μM γ-T3 or 10 mM LiCl alone or combined with 1 μM XAV-939 for 72 h were determined. ON expressed by osteoblasts, a calcium-binding phosphorylated glycoprotein, was higher in the maturation and mineralization stages than in the proliferation stage.70 ON induced calcium and phosphorus deposition in bones to form hydroxyapatite crystals in the stage of cell differentiation and binds tightly with type I, III, and V collagen to promote bone calcification.71 OCN and OPN are considered to be more specific markers at the late stage of osteogenic differentiation.72 OCN expressed by osteoblasts is a non-collagen acidic glycoprotein that promotes bone mineralization and reflects the end of the differentiation and maturation of osteoblasts.73,74 Pre-osteoblasts or stem cells differentiate into osteoblasts that form bones by recognizing and adhering OPN, which can also facilitate the attachment of osteoblasts to the extracellular matrix.75–77 The effects of γ-T3 on the ALP activity and expressions of ON, OPN and OCN proteins were similar to that of LiCl, which were reversed by XAV-939 (Fig. 5A–D). In vitro studies showed that the Wnt/β-catenin signaling pathway was an upstream activator of BMP-2 and BMP-4 expressions and affects osteoblast differentiation and mineralization.37,40,78–80 Our results suggested that 4 μM γ-T3 significantly upregulated the expression levels of BMP-2 and BMP-4 (Fig. 5E and F), which corroborated the results we obtained previously.41 Furthermore, the effects of γ-T3 were also blocked by XAV-939 (Fig. 5E and F). These results indicated that γ-T3 could induce the differentiation of MC3T3-E1 cells by enhancing the Wnt/β-catenin signaling pathway and subsequently stimulating the BMP-2 and BMP-4 expressions.
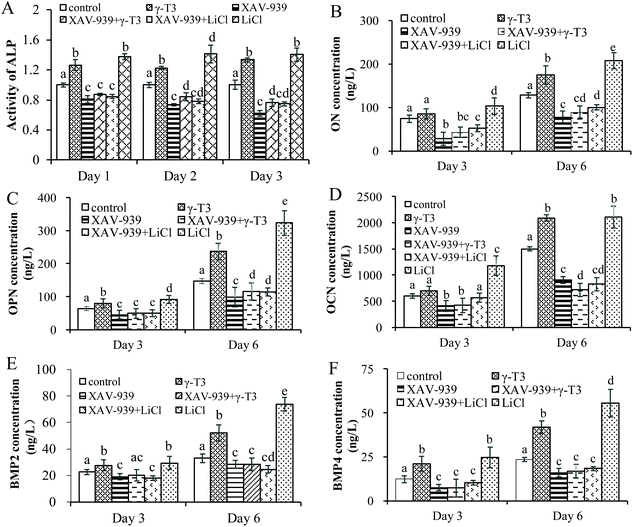 |
| Fig. 5 The effect of XAV-939 on the γ-T3-induced differentiation of MC3T3-E1 cells. The ALP activity (A) and expression levels of ON (B), OPN (C), OCN (D), BMP-2 (E) and BMP-4 (F) of the MC3T3-E1 cells treated individually or in combination with γ-T3, XAV-939 and LiCl for 3 and 6 days were detected using kits. Data are expressed as mean ± SD of three independent experiments. Values with different letters indicate significant differences (P < 0.05), whereas values with same letters indicate that the difference is not significant (P > 0.05). | |
4. Conclusions
In summary, γ-T3 promoted osteoblastic proliferation and differentiation through stimulating the Wnt/β-catenin signaling pathway by the accumulation and nuclear translocation of β-catenin, which led to increased expressions of the downstream proliferation-related target genes cyclin D1 and c-myc, and differentiation-related target genes BMP-2 and BMP-4. This study indicated that γ-T3 can be used as a natural agent for the prevention and treatment of osteoporosis induced by various factors via the Wnt/β-catenin signaling pathway. However, the present study still has some limitations. Therefore, further study should be performed to determine the effect of γ-T3 on multiple cell lines which mimic the condition of natural bone microenvironment. Moreover, γ-T3 might exert important effects on osteoblasts through multiple signaling pathways and the interaction between them,81,82 and so the molecular mechanism of γ-T3 on osteoblasts needs further research and improvement.
Author contributions
Weili Xu: conceptualization, formal analysis, funding acquisition, investigation, methodology, project administration, resources, supervision, and writing – review and editing. Yutong Li: writing – original draft. Rennan Feng: data curation and writing – original draft. Pan He: data curation, formal analysis, investigation, methodology, and writing – original draft. Yuqi Zhang: formal analysis, investigation, methodology, and writing – original draft.
Conflicts of interest
The authors declare that they have no known competing financial interests or personal relationships that could have appeared to influence the work reported in this paper.
Acknowledgements
This work was supported by the National Natural Science Foundation of China (31501481). The authors wish to thank Dr Rong Tsao at the Guelph Research and Development Centre, Agriculture and Agri-Food Canada (AAFC) for revising and improving the manuscript.
References
- T. J. Martin, J. H. Gooi and N. A. Sims, Molecular mechanisms in coupling of bone formation to resorption, Crit. Rev. Eukaryotic Gene Expression, 2009, 19(1), 73–88 CrossRef CAS PubMed.
- N. Richard, W. Tina, N. Scott, N. Jonathan and L. Joanne, The clinical epidemiology of male osteoporosis: a review of the recent literature, Clin. Epidemiol., 2015, 7(default), 65–76 Search PubMed.
- R. Burge, B. Dawson-Hughes, D. H. Solomon, J. B. Wong, A. King and A. Tosteson, Incidence and economic burden of osteoporosis-related fractures in the United States, 2005–2025, J. Bone Miner. Res., 2010, 22(3), 465–475 CrossRef PubMed.
- F. Borgström, Pos8 the societal burden of osteoporosis, Curr. Rheumatol. Rep., 2010, 12(3), 186 CrossRef PubMed.
- J. M. Spatz, E. Rachel, A. M. Cloutier, L. Leeann, V. V. Miranda, L. J. Suva, D. Denise, S. Marina, K. H. Zhu and M. L. Bouxsein, Sclerostin antibody inhibits skeletal deterioration due to reduced mechanical loading, J. Bone Miner. Res., 2013, 28(4), 865–874 CrossRef CAS PubMed.
- S. Khosla and L. C. Hofbauer, Osteoporosis treatment: recent developments and ongoing challenges, Lancet Diabetes Endocrinol., 2017, 5(11), 898–907 CrossRef PubMed.
- P. Morizio, J. I. Burkhart and S. Ozawa, Denosumab: A Unique perspective on adherence and cost-effectiveness compared with oral bisphosphonates in osteoporosis patients, Ann. Pharmacother., 2018, 52(10), 1031–1041 CrossRef CAS PubMed.
- M. Norazlina, S. Imanirwana, M. T. Gapor and B. A. K. Khalid, Palm vitamin E is comparable to α-tocopherol in maintaining bone mineral density in ovariectomised female rats, Exp. Clin. Endocrinol. Diabetes, 2000, 108(04), 305–310 CrossRef CAS PubMed.
- S. Imanirwana, A. Kiftiah, A. G. Zainal, M. Norazlina, M. T. Gapor and B. A. K. Khalid, Palm vitamin E prevents osteoporosis in orchidectomized growing male rats, Nat. Prod. Sci., 2000, 6(4), 155–160 CAS.
- S. Zakaria, S. Z. Mat-Husain, Y. H. Kong, K. Xin-Kai and N. Mohamed, Vitamin E improved bone strength and bone minerals in male rats given alcohol, Iran. J. Basic Med. Sci., 2017, 20(12), 1360–1367 Search PubMed.
- B. J. Smith, E. A. Lucas, R. T. Turner, G. L. Evans, M. R. Lerner, D. J. Brackett, B. J. Stoecker and B. H. Arjmandi, Vitamin E provides protection for bone in mature hindlimb unloaded male rats, Calcif. Tissue Int., 2005, 76(4), 272–279 CrossRef CAS PubMed.
- B. H. Arjmandi, S. Juma, A. Beharka, M. S. Bapna, M. Akhter and S. N. Meydani, Vitamin E improves bone quality in the aged but not in young adult male mice, J. Nutr. Biochem., 2002, 13(9), 543–549 CrossRef CAS PubMed.
- J. M. Mata-Granados, R. Cuenca-Acebedo, M. D. L. de Castro and J. M. Q. Gomez, Lower vitamin E serum levels are associated with osteoporosis in early postmenopausal women: a cross-sectional study, J. Bone Miner. Metab., 2013, 31(4), 455–460 CrossRef CAS PubMed.
- M. A. Iqubal, M. Khan, P. Kumar, A. Kumar and K. Ajai, Role of Vitamin E in prevention of oral cancer: a review, J. Clin. Diagn. Res., 2014, 8, 5–7 Search PubMed.
- R. Brigelius-Flohe, F. Kelly, J. Salonen, J. Neuzil, J. Zingg and A. Azzi, The european perspective on vitamin E: current knowledge and future research, Am. J. Clin. Nutr., 2002, 76, 703–716 CrossRef CAS PubMed.
- F. Galli, A. Azzi, M. Birringer, J. M. Cook-Mills, M. Eggersdorfer, J. Frank, G. Cruciani, S. Lorkowski and N. K. özer, Vitamin E: Emerging aspects and new directions, Free Radical Biol. Med., 2017, 102, 16–36 CrossRef CAS PubMed.
- T. Uysal, M. Amasyali, H. Olmez, Y. Karslioglu and O. Gunhan, Stimulation of bone formation in the expanding inter-maxillary suture by vitamin E, in rat, Korean J. Orthod., 2009, 39(5), 337–347 CrossRef.
- J. Zhang, X. Hu and J. Zhang, Associations between serum vitamin E concentration and bone mineral density in the US elderly population, Osteoporosis Int., 2017, 28(4), 1245–1253 CrossRef CAS PubMed.
- M. S. Hamidi, P. N. Corey and A. M. Cheung, Effects of vitamin E on bone turnover markers among US postmenopausal women, J. Bone Miner. Res., 2012, 27(6), 1368–1380 CrossRef CAS PubMed.
- N. Muhammad, D. A. Luke, A. N. Shuid, N. Mohamed and I. N. Soelaiman, Tocotrienol supplementation in postmenopausal osteoporosis: evidence from a laboratory study, Clinics, 2013, 68(10), 1338–1343 CrossRef PubMed.
- N. V. Mohamad, S. Ima-Nirwana and K. Y. Chin, Therapeutic potential of annatto tocotrienol with self-emulsifying drug delivery system in a rat model of postmenopausal bone loss, Biomed. Pharmacother., 2021, 137(3), 111368 CrossRef CAS PubMed.
- N. V. Mohamad, S. Ima-Nirwana and K. Y. Chin, Self- emulsified annatto tocotrienol improves bone histomorphometric parameters in a rat model of oestrogen deficiency through suppression of skeletal sclerostin level and RANKL/OPG ratio, Int. J. Med. Sci., 2021, 18(16), 3665–3673 CrossRef PubMed.
- S. Mohamad, A. N. Shuid, S. A. Mokhtar, S. Abdullah and I. N. Soelaiman, Tocotrienol supplementation improves late-phase fracture healing compared to alpha-tocopherol in a rat model of postmenopausal osteoporosis: a biomechanical evaluation, Evid. Based Complement. Alternat. Med., 2012, 2012(2039), 372878 Search PubMed.
- S. K. Wong, K. Y. Chin and S. Ima-Nirwana, The effects of tocotrienol on bone peptides in a rat model of osteoporosis induced by metabolic syndrome: the possible communication between bone cells, Int. J. Environ. Res. Public Health, 2019, 16(18), 3313 CrossRef CAS PubMed.
- K. Y. Chin and S. Ima-Nirwana, Effects of annatto-derived tocotrienol supplementation on osteoporosis induced by testosterone deficiency in rats, Clin. Interventions Aging, 2014, 9, 1247–1259 CrossRef.
- N. S. Ahmad, B. A. K. Khalid, D. A. Luke and S. I. Nirwana, Tocotrienol offers better protection than tocopherol from free radical-induced damage of rat bone, Clin. Exp. Pharmacol. Physiol., 2010, 32(9), 761–770 CrossRef PubMed.
- M. Norazlina, P. L. Lee, H. I. Lukman, A. S. Nazrun and S. Ima-Nirwana, Effects of vitamin E supplementation on bone metabolism in nicotine-treated rats, Singapore Med. J., 2007, 48(3), 195–199 CAS.
- N. F. M. Fozi, J. J. Jolly, C. K. Hui, E. Alias, C. K. Yong and I. N. Soelaiman, Comparing the effects of alpha-tocopherol and tocotrienol isomers on osteoblasts hFOB 1.19 cultured on bovine bone scaffold, Sains Malays., 2021, 50(8), 2319–2328 CrossRef.
- M. Z. Mehat, A. N. Shuid, N. Mohamed, N. Muhammad and I. N. Soelaiman, Beneficial effects of vitamin E isomer supplementation on static and dynamic bone histomorphometry parameters in normal male rats, J. Bone Miner. Metab., 2010, 28(5), 503–509 CrossRef CAS PubMed.
- S. Ima-Nirwana and S. Suhaniza, Effects of tocopherols and tocotrienols on body composition and bone calcium content in adrenalectomized rats replaced with dexamethasone, J. Med. Food, 2004, 7(1), 45–51 CrossRef CAS PubMed.
- H. Hermizi, O. Faizah, S. Ima-Nirwana, S. A. Nazrun and M. Norazlina, Beneficial effects of tocotrienol and tocopherol on bone histomorphometric parameters in sprague-dawley male rats after nicotine cessation, Calcif. Tissue Int., 2009, 84(1), 65–74 CrossRef CAS PubMed.
- L. Deng, Y. Ding, Y. Peng, Y. Wu, J. Fan, W. Li, R. Yang, M. Yang and Q. Fu, gamma-Tocotrienol protects against ovariectomy-induced bone loss via mevalonate pathway as HMG-CoA reductase inhibitor, Bone, 2014, 67, 200–207 CrossRef CAS PubMed.
- M. K. Mohammed, C. Shao, J. Wang, Q. Wei, X. Wang, Z. Collier, S. Tang, H. Liu, F. Zhang, J. Huang, D. Guo, M. Lu, F. Liu, J. Liu, C. Ma, L. L. Shi, A. Athiviraham, T. C. He and M. J. Lee, Wnt/beta-catenin signaling plays an ever-expanding role in stem cell self-renewal, tumorigenesis and cancer chemoresistance, Genes Dis., 2016, 3(1), 11–40 CrossRef PubMed.
- S. Angers and R. T. Moon, Proximal events in Wnt signal transduction, Nat. Rev. Mol. Cell Biol., 2009, 10(7), 468–477 CrossRef CAS PubMed.
- H. Clevers and R. Nusse, Wnt/beta-catenin signaling and disease, Cell, 2012, 149(6), 1192–1205 CrossRef CAS PubMed.
- V. Nardone, F. D'Asta and M. L. Brandi, Pharmacological management of osteogenesis, Clinics, 2014, 69(6), 438–446 CrossRef PubMed.
- R. R. Zhang, B. O. Oyajobi, S. E. Harris, D. Chen, C. Tsao, H. W. Deng and M. Zhao, Wnt/beta-catenin signaling activates bone morphogenetic protein 2 expression in osteoblasts, Bone, 2013, 52(1), 145–156 CrossRef CAS PubMed.
- S. L. Holmen, C. R. Zylstra, A. Mukherjee, R. E. Sigler, M. C. Faugere, M. L. Bouxsein, L. F. Deng, T. L. Clemens and B. O. Williams, Essential role of beta-catenin in postnatal bone acquisition, J. Biol. Chem., 2005, 280(22), 21162–21168 CrossRef CAS PubMed.
- V. S. Salazar, Z. Nicholas, H. Lisa, W. Marcus, K. Jacqueline, B. Sheri, N. Jin, M. Gabriel and C. Roberto, Postnatal ablation of osteoblast Smad4 enhances proliferative responses to canonical Wnt signaling through interactions with β-catenin, J. Cell Sci., 2013, 126(24), 5598–5609 CAS.
- G. Rawadi, B. Vayssiere, F. Dunn, R. Baron and S. Roman-Roman, BMP-2 controls alkaline phosphatase expression and osteoblast mineralization by a Wnt autocrine loop, J. Bone Miner. Res., 2003, 18(10), 1842–1853 CrossRef CAS PubMed.
- W. Xu, P. He, S. He, P. Cui, Y. Mi, Y. Yang, Y. Li and S. Zhou, Gamma-tocotrienol stimulates the proliferation, differentiation, and mineralization in osteoblastic MC3T3-E1 cells, J. Chem., 2018, 2018, 3805932 Search PubMed.
- Z. Hamidouche, E. Ha, P. Vaudin, P. Charbord and O. Fromigué, FHL2 mediates dexamethasone-induced mesenchymal cell differentiation into osteoblasts by activating Wnt/beta-catenin signaling-dependent Runx2 expression, FASEB J., 2008, 22(11), 3813–3822 CrossRef CAS PubMed.
- C. Vater, P. Kasten and M. Stiehler, Culture media for the differentiation of mesenchymal stromal cells, Acta Biomater., 2011, 7(2), 463–477 CrossRef CAS PubMed.
- E. Nemoto, Y. Koshikawa, S. Kanaya, M. Tsuchiya, M. Tamura, M. J. Somerman and H. Shimauchi, Wnt signaling inhibits cementoblast differentiation and promotes proliferation, Bone, 2009, 44(5), 805–812 CrossRef CAS PubMed.
- M. Kassem and P. J. Marie, Senescence-associated intrinsic mechanisms of osteoblast dysfunctions, Aging Cell, 2011, 10(2), 191–197 CrossRef CAS PubMed.
- P. J. Marie and M. Kassem, Osteoblasts in osteoporosis: past, emerging, and future anabolic targets, Eur. J. Endocrinol., 2011, 165(1), 1–10 CAS.
- H. Sudo, H. A. Kodama, Y. Amagai, S. Yamamoto and S. Kasai, In vitro differentiation and calcification in a new clonal osteogenic cell line derived from newborn mouse calvaria, J. Cell Biol., 1983, 96(1), 191–198 CrossRef CAS PubMed.
- M. N. Abd, N. Mohamed and A. N. Shuid, Effects of low-dose versus high-dose γ-tocotrienol on the bone cells exposed
to the hydrogen peroxide-induced oxidative stress and apoptosis, Evid. Based Complement. Alternat. Med., 2012, 2012(2012), 680834 Search PubMed.
- N. Fratzl-Zelman, I. Schmidt, P. Roschger, A. Roschger, F. H. Glorieux, K. Klaushofer, W. Wagermaier, F. Rauch and P. Fratzl, Unique micro- and nano-scale mineralization pattern of human osteogenesis imperfecta type VI bone, Bone, 2015, 73, 233–241 CrossRef CAS PubMed.
- W. H. W. Nuraini, A. G. Norzana, C. Kok-Yong and I. N. Soelaiman, Annatto-derived tocotrienol stimulates osteogenic activity in preosteoblastic MC3T3-E1 cells: a temporal sequential study, Drug Des. Dev. Ther., 2018, 12, 1715–1726 CrossRef PubMed.
- K. H. Park, J. W. Kang, E. M. Lee, J. S. Kim, Y. H. Rhee, M. Kim, S. J. Jeong, Y. G. Park and S. H. Kim, Melatonin promotes osteoblastic differentiation through the BMP/ERK/Wnt signaling pathways, J. Pineal Res., 2011, 51(2), 187–194 CrossRef CAS PubMed.
- W. Huang, P. Wang, T. Shen, C. Hu, Y. Han, M. Song, Y. Bian, Y. Li and Y. Zhu, Aluminum trichloride inhibited osteoblastic proliferation and downregulated the Wnt/β-catenin pathway, Biol. Trace Elem. Res., 2017, 177(2), 323–330 CrossRef CAS PubMed.
- H. Choi, J. Gwak, M. Cho, M. J. Ryu, J. H. Lee, S. K. Kim, Y. H. Kim, G. W. Lee, M. Y. Yun, M. C. Nguyen, J. G. Shin, G. Y. Song and S. Oh, Murrayafoline A attenuates the Wnt/beta-catenin pathway by promoting the degradation of intracellular beta-catenin proteins, Biochem. Biophys. Res. Commun., 2010, 391(1), 915–920 CrossRef CAS PubMed.
- S. Park, J. Gwak, M. Cho, T. Song, J. Won, D. E. Kim, J. G. Shin and S. Oh, Hexachlorophene inhibits Wnt/beta-catenin pathway by promoting Siah-mediated beta-catenin degradation, Mol. Pharmacol., 2006, 70(3), 960–966 CrossRef CAS PubMed.
- X. Zhang, Q. Chen, J. Liu, C. Fan, Q. Wei, Z. Chen and X. Mao, Parthenolide promotes differentiation of osteoblasts through the Wnt/beta-catenin signaling pathway in inflammatory environments, J. Interferon Cytokine Res., 2017, 37(9), 406–414 CrossRef CAS PubMed.
- C. M. Hedgepeth, L. J. Conrad, J. Zhang, H. C. Huang, V. M. Y. Lee and P. S. Klein, Activation of the wnt signaling pathway: A molecular mechanism for lithium action, Dev. Biol., 1997, 185(1), 82–91 CrossRef CAS PubMed.
- X. Q. Gan, J. Y. Wang, Y. Xi, Z. l. Wu, Y. P. Li and L. Li, Nuclear Dvl, c-Jun, beta-catenin, and TCF form a complex leading to stabilization of beta-catenin-TCF interaction, J. Cell Biol., 2008, 180(6), 1087–1100 CrossRef CAS PubMed.
- C. Wang, Z. W. Luo, H. M. Zhu, Z. R. Sun, Z. Xiang, Y. Y. Ge, C. Ni, W. P. Qian and X. D. Han, Inhibition of Wnt/beta-catenin signaling promotes epithelial differentiation of mesenchymal stem cells and repairs bleomycin-induced lung injury, Am. J. Physiol., 2014, 307(3), C234–C244 CrossRef CAS PubMed.
- R. T. Peterson, Drug discovery: propping up a destructive regime, Nature, 2009, 461(7264), 599–600 CrossRef CAS PubMed.
- R. Marsell and T. A. Einhorn, The biology of fracture healing, Injury, 2011, 42(6), 551–555 CrossRef PubMed.
- J. Kular, J. Tickner, S. M. Chim and J. K. Xu, An overview of the regulation of bone remodelling at the cellular level, Clin. Biochem., 2012, 45(12), 863–873 CrossRef CAS PubMed.
- N. A. Sims and C. Vrahnas, Regulation of cortical and trabecular bone mass by communication between osteoblasts, osteocytes and osteoclasts, Arch. Biochem. Biophys., 2014, 561, 22–28 CrossRef CAS PubMed.
- T. C. He, A. B. Sparks, C. Rago, H. Hermeking, L. Zawel, L. T. D. Costa, P. J. Morin, B. Vogelstein and K. W. Kinzler, Identification of c-MYC as a target of the APC pathway, Science, 1998, 281(5382), 1509 CrossRef CAS PubMed.
- O. Tetsu and F. Mccormick, Beta-Catenin regulates expression of cyclin D1 in colon carcinoma cells, Nature, 1999, 398(6726), 422–426 CrossRef CAS PubMed.
- J. F. L. Chau, W. F. Leong and B. Li, Signaling pathways governing osteoblast proliferation, differentiation and function, Histol. Histopathol., 2009, 24(12), 1593 CAS.
- M. D. Cole and V. H. Cowling, Transcription-independent functions of MYC: regulation of translation and DNA replication, Nat. Rev. Mol. Cell Biol., 2008, 9(10), 810–815 CrossRef CAS PubMed.
- Y. Chen, T. T. Jiang, L. H. Shi and K. Y. He, hcrcn81 promotes cell proliferation through Wnt signaling pathway in colorectal cancer, Med. Oncol., 2016, 33(1), 13 CAS.
- C. J. Sherr, Cancer cell cycles, Science, 1996, 274(5293), 1672–1677 CrossRef CAS PubMed.
- J. I. Daksis, R. Y. Lu, L. M. Facchini, W. W. Marhin and L. J. Z. Penn, Myc induces cyclin D1 expression in the absence of de novo protein synthesis and links mitogen-stimulated signal transduction to the cell cycle, Oncogene, 1994, 9(12), 3635–3645 CAS.
- J. Y. Choi, B. H. Lee, K. B. Song and R. W. Park, Expression pattern of bone-related proteins during osteoblastic differentiation in MC3T3-E1 cells, J. Cell. Biochem., 1996, 61(4), 609–618 CrossRef CAS PubMed.
- T. Sasaki, W. Gohring, K. Mann, P. Maurer, E. Hohenester, V. Knauper, G. Murphy and R. Timpl, Limited cleavage of extracellular matrix protein BM-40 by matrix metalloproteinases increases its affinity for collagens, J. Biol. Chem., 1997, 272(14), 9237–9243 CrossRef CAS PubMed.
- J. C. Reichert, V. M. Quent, L. J. Burke, S. H. Stansfield, J. A. Clements and D. W, Hutmacher, Mineralized human primary osteoblast matrices as a model system to analyse interactions of prostate cancer cells with the bone microenvironment, Biomaterials, 2010, 31(31), 7928–7936 CrossRef CAS PubMed.
- K. Nakashima, X. Zhou, G. Kunkel, Z. Zhang, J. M. Deng, R. R. Behringer and B. D. Crombrugghe, The novel zinc finger-containing transcription factor osterix is required for osteoblast differentiations and bone formation, Cell, 2002, 108, 17–29 CrossRef CAS PubMed.
- R. T. Franceschi, C. Ge, G. Xiao, H. Roca and D. Jiang, Transcriptional regulation of osteoblasts, Cells Tissues Organs, 2009, 189(1–4), 144–152 CrossRef CAS PubMed.
- G. R. Beck, B. Zerler and E. Moran, Phosphate is a specific signal for induction of osteopontin gene expression, Proc. Natl. Acad. Sci. U. S. A., 2000, 97(15), 8352–8357 CrossRef CAS PubMed.
- Q. Wu, D. Wang, H. Sun and L. Zhang, Effects of osteopontin in mice bone marrow-derived mesenchymal stem cells differentiating into osteoblasts in vitro, China Medical Herald, 2009, 6(36), 14–16 Search PubMed.
- T. Yabe, A. Nemoto and T. Uemura, Recognition of osteopontin by rat bone marrow derived osteoblastic primary cells, Biosci. Biotechnol. Biochem., 1997, 61(4), 754–756 CrossRef CAS PubMed.
- M. H. Lee, Y. J. Kim, H. J. Kim, H. D. Park, A. R. Kang, H. M. Kyung, J. H. Sung, J. M. Wozney, H. J. Kim and H. M. Ryoo, BMP-2-induced Runx2 expression is mediated by Dlx5, and TGF-beta 1 opposes the BMP-2-induced osteoblast differentiation by suppression of Dlx5 expression, J. Biol. Chem., 2003, 278(36), 34387–34394 CrossRef CAS PubMed.
- G. Van Der Horst, R. L. Van Bezooijen, M. M. L. Deckers, J. Hoogendam, A. Visser, C. Lowik and M. Karperien, Differenitiation of murine preosteoblastic KS483 cells depends on autocrine bone morphogenetic protein signaling during all phases of osteoblast formation, Bone, 2002, 31(6), 661–669 CrossRef CAS PubMed.
- M. Knippenberg, M. N. Helder, B. Z. Doulabi, P. I. J. M. Wuisman and J. Klein-Nulend, Osteogenesis versus chondrogenesis by bmp-2 and bmp-7 in adipose stem cells, Biochem. Biophys. Res. Commun., 2006, 342(3), 902–908 CrossRef CAS PubMed.
- K. Y. Chin, H. Mo and I. N. Soelaiman, A review of the possible mechanisms of action of tocotrienol - a potential antiosteoporotic agent, Curr. Drug Targets, 2013, 14(13), 1533–1541 CrossRef CAS PubMed.
- W. N. W. Hasan, K. Y. Chin, J. J. Jolly, N. A. Ghafar and I. N. Soelaiman, Identifying potential therapeutics for osteoporosis by exploiting the relationship between mevalonate pathway and bone metabolism, Endocr., Metab. Immune Disord.: Drug Targets, 2018, 18(5), 450–457 CrossRef CAS PubMed.
|
This journal is © The Royal Society of Chemistry 2022 |
Click here to see how this site uses Cookies. View our privacy policy here.