DOI:
10.1039/D1FO02264D
(Paper)
Food Funct., 2022,
13, 213-226
Intake of high-purity insoluble dietary fiber from Okara for the amelioration of colonic environment disturbance caused by acute ulcerative colitis
Received
14th July 2021
, Accepted 17th November 2021
First published on 19th November 2021
Abstract
High-purity insoluble dietary fiber from okara (okara-HPIDF) is a raw material with a potentially positive effect on colon health. However, the mechanisms of the effect are far from clear. In this study, okara-HPIDF and low-purity dietary fiber from okara (okara-LPDF) were fed to C57BL/6 mice with acute ulcerative colitis induced by DSS. The levels of inflammatory factors, bacterial 16S rDNA sequencing, short-chain fatty acids (SCFAs), and bioinformatics were analyzed with the colonic tissue status. The results showed that the intake of HPIDF affected the proliferation of the key bacteria Shigella, Lactobacillus, and Peptostreptococcaceae in the PWY-2941 pathway and AEROBACTINSYN-PWY pathway, and then affected the synthesis of SCFAs, providing a positive role for colon health. However, the intake of HPIDF was unable to repair colonic injury caused by DSS-induced acute ulcerative colitis mainly owing to the abundance of Shigella in the colon. This study demonstrates that the recommended intake content of HPIDF can ameliorate colonic environment disturbance caused by acute ulcerative colitis, but not enough to relieve it.
Introduction
Dietary fiber is labeled “the seventh nutrient” because of its unique function.1 Dietary fiber cannot be digested and decomposed in vivo, but can be used as a fermentation substrate by gut microbiota, which can produce short-chain fatty acids (SCFAs) and other beneficial substances.2,3 These substances affect intestinal health by changing the internal intestinal environment and intestinal permeability.4,5 In east Asia, most people's long-term dietary habits are mainly based on cereals, which are a known source of dietary fiber, and hence are expected to depict essential health functions. However, recent statistics indicate that the number of patients with colitis and colon cancer is continuously increasing year by year due to changes in dietary habits.6 Therefore, it is reasonable to believe that dietary fiber intake is closely related to intestinal diseases. Most researchers have used soluble dietary fiber (SDF), which is easier to be used by gut microbiota,7 to study the association between dietary fiber and intestinal health. However, this does not neglect the functionality of insoluble dietary fiber (IDF), such as the intake of IDF can alleviate intestinal inflammation.8–10
IDF, as a kind of food material that can improve intestinal health, has been gradually accepted and developed as a kind of functional food material. However, recent studies have shown that IDF is not necessarily beneficial to intestinal health.11–13 Owing to IDF intake, the symptoms of ulcerative colitis in experimental animals were found to be aggravated. Some researchers thus put forward a new concept: Not All Fibers Are Born Equal.14 Roughly ten subtypes of dietary fibers have been described to date, categorized as soluble or insoluble, with varying chemical structures and large differences in their fermentation profiles. Naturally, changes in the gut microbiota and intestinal environment are complex. Not only can probiotics influence the metabolism and proliferation, so can other non-probiotics, which may be the main reason for the deterioration in intestinal inflammation. In addition, during the formation of ulcerative colitis, excessive dietary fiber intake will influence nutrient absorption, whereby the body needs more nutrients. The above-mentioned aspects need to be further studied to verify the mechanism.
At the beginning of this series of research, we prepared high-purity insoluble dietary fiber from okara (okara-HPIDF) by the combined enzyme method. Its unique physicochemical properties made us realize that it has the potential to be further developed into a functional food, especially for colonic health.15 Subsequently, when exploring its intervention mechanism on acute ulcerative colitis, mainly animal experiments, it was found that the intake of HPIDF with DSS induction had little effect on the inflammation, which was beyond our expectation.
In this study, to explain why the intake of HPIDF during DSS induction reduced ulcerative colitis, 16S rDNA gene sequencing was used to qualitatively and quantitatively analyze the gut microbiota of the experimental animals, and the differences were compared. The formation of SCFAs in the colon was determined by GC-MS. Meanwhile, the metabolism of gut microbiota was explored, and the changes in the colonic environment after the intake of HPIDF were evaluated.
Materials and methods
Low-purity insoluble dietary fiber (LPDF) and HPIDF preparation
Low-purity dietary fiber (LPDF) powder (art. no. SOYFIB820) derived from okara (protein ≤ 26%, moisture ≤ 10%, ash ≤ 6%, dietary fiber ≥ 60%) was purchased from Shandong Sinoglory Health Food Co., Ltd (Liaocheng, China). High-purity insoluble dietary fiber (HPIDF) from okara was prepared by the three-step enzymatic hydrolysis of LPDF described in previous studies.15,16
Experimental animals and groups
Establishment of the DSS-induced colitis mouse model and treatment with DF
The establishment of the acute DSS colitis model was determined by the method described by Wirtz et al.17 Six- to eight-week-old C57BL/6 female mice were purchased from Beijing Vital River Laboratory Animal Technology Co. Ltd (Beijing, China). All the mice were housed under a controlled condition in individual cages at 22 ± 3 °C and 60%–70% relative humidity with a 12 h dark/light cycle in a specific pathogen-free environment and were allowed free access to food and water.
After one week of acclimatization, the mice were randomly divided into five groups: the control group (Con, water, free-feeding, n = 6), DSS group (water containing 3% DSS (w/v) (molecular weight 36
000–50
000; MP Biomedicals) for 7 days, n = 6), HPIDF group (HPIDF, 500 mg kg−1 d−1, n = 6), DSS + HPIDF group (3% DSS + HPIDF for 7 days, 500 mg kg−1 d−1, n = 6), and DSS + LPDF group (3% DSS + LPDF for 7 days, 500 mg kg−1 d−1, n = 6). All the mice were given a single oral gavage daily at 2 pm, and were fed a normal diet (Fig. 1A). The content of DF intake of the experimental animals was converted from the recommended content for the human body (30 g d−1, Food and Agricultural Organization of the United Nations).
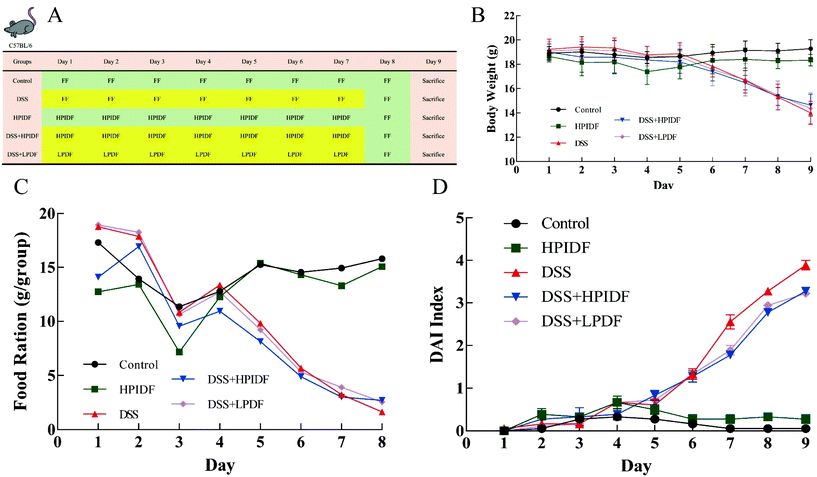 |
| Fig. 1 Animal experiment process and state changes (n = 6). (A) Process of the animal experiments (yellow backgrounds: water containing 3% DSS (w/v), drinking freely; green background: pure water, drinking freely; FF: free feeding). (B) Bodyweight changes during treatment. (C) Food ration changes during treatment. (D) DAI index changes during treatment. | |
The weight of the experimental animals in each group was measured daily during the intervention. We also observed the time and rate of modeling, including the mental state, activity, and stool shape. All the animal experiments were approved by the Institutional Animal Care and Use Committees of Northeast Agricultural University, following the National Research Council Guidelines.
Disease activity index (DAI) of the colitis mice
The DAI of the colitis mouse model was evaluated as in Liu's method18 with minor changes. Bodyweight, feces condition, and fecal blood test scores were used to evaluate DAI comprehensively according to the following formula.
DAI = (bodyweight scores + feces condition scores + fecal blood scores)/3 |
Bodyweight was scored as follows: 0 = no weight loss, 1 = 0–5% weight loss, 2 = 5%–10% weight loss, 3 = 10%–15% weight loss, 4 = 15% weight loss or more.
Feces condition was scored as follows: 0 = normal, 1 = between 0 and 2, 2 = loss of form, 3 = between 2 and 4, 4 = diarrhea.
Fecal blood was scored as follows: 0 = no blood, 1 = between 0 and 2, 2 = bloody stool recessively, 3 = between 2 and 4, 4 = bloody stool obviously.
Material sampling for the evaluation of colonic inflammation
On day 9, blood was collected before sacrificing the mice by cervical dislocation and placing them supine on a surgical pad. Using sterile forceps and scissors, each mouse was carefully opened by a ventral midline incision. The full colon was removed and its weight and length determined. The colonic solutes were collected (about 1 g per mouse) and frozen at −80 °C for 16S rDNA sequencing. The colon was rinsed with sterile PBS using a steel gavage needle attached to a 5 ml syringe to remove feces. Next, 0.5 cm long colonic fragments were cut from the proximal and distal parts of the colon for hematoxylin and eosin (H&E) staining.
ELISA analysis
Blood was drawn from the eyeball and centrifuged (3000g, 10 min, 4 °C) to obtain the serum. Then, TNF-α was measured according to the ELISA kit protocol (no. ML002095, Shanghai Enzyme-linked Biotechnology Co., Ltd, Shanghai, China). The results were presented as pg mL−1 serum.
Next, 0.1 g colon tissue was homogenized with 1 ml PBS buffer. After being fully homogenized, the samples were centrifuged at 3000 rpm for 20 min. The supernatant was collected for the measurement of myeloperoxidase (MPO), superoxide dismutase (SOD), and reactive oxygen species (ROS) according to the ELISA kits (no. ML002070, no. 643059, no. ML009876-1, Shanghai Enzyme-linked Biotechnology Co., Ltd, Shanghai, China). The results are presented as ng mL−1 homogenate.
Hematoxylin and eosin (H&E) staining
H&E staining was determined by the method described by Yang et al.19 First, 0.5 cm colonic segments were fixed in 4% paraformaldehyde solution for 12 h at room temperature and embedded in paraffin. After cutting the paraffin into 5 μm sections, the slides were dewaxed, rehydrated, and stained with 1% H&E at room temperature, as described previously.
Bacterial 16S rDNA sequencing
DNA extractions from feces were implemented using the QIAamp® DNA Stool Mini Kit (QIAGEN, Germany), according to the manufacturer's instructions. The quality of isolated DNAs was evaluated by agarose gel electrophoresis and then by precisely measuring the DNA concentration using a NanoDrop NC2000 spectrophotometer (Thermo Fisher, Waltham, MA, USA).
The V3–V4 region of the 16S rRNA gene was amplified from the purified DNA of each sample. The primers used for the PCR amplification were as follows: the forward primer 515F (5′-ACTCCTACGGGAGGCAGCA-3′) and the reverse primer 907R (5′-GGACTACHVGGGTWTCTAAT-3′). The concentrations of PCR products were measured by the Quant-iT PicoGreen dsDNA Assay Kit (Invitrogen, Carlsbad, CA, USA). Afterward, all the PCR products were pooled in equal amounts, and paired-end sequencing was performed using the Illumina MiSeq platform operated by Shanghai Personal Biotechnology Co., Ltd (Shanghai, China).
Short-chain fatty acids’ (SCFA) analysis
An appropriate amount of sample mixed with 50 μL 15% phosphoric acid, 100 mg glass beads, 125 μg mL−1 internal standard (isohexanoic acid) solution 100 μL, and 400 μL ether, before putting it into a high-throughput tissue grinder, where the sample was ground at 60 Hz for 60 s, repeated twice. After centrifugation at 4 °C and 12
000 rpm for 10 min, the supernatant was taken and tested by GC-MS (Thermo TRACE 1310-ISQ LT, Waltham, MA, USA).
Chromatographic conditions: Agilent HP-INNOWAX capillary column (30 m × 0.25 mm ID × 0.25 μm); split injection; injection volume, 1 μL; split ratio, 10
:
1; injection port temperature, 250 °C; temperature of the ion source, 230 °C; transmission line temperature, 250 °C; quadrupole temperature: 150 °C, temperature profile for the temperature ramping program: initial temperature, i90 °C. The temperature was then raised to 120 °C at 10 °C min−1; then the temperature was raised to 150 °C at 5 °C min−1; finally, the temperature was raised to 250 °C for 2 min at 25 °C min−1. Also, the carrier gas was helium with a flow rate of 1.0 mL min−1.
MS conditions: electron impact ionization (EI) source; SIM scanning mode; electron energy, 70 eV.
Bioinformatics analysis
The valid sequences were clustered into operational taxonomic units (OTUs) at 97% sequence identity using UCLUST,20 and then compared with the Greengenes 16S rRNA database. After confirming reproducibility, treatment replicate sequence runs were combined into a single file for each sample (biological replicates were not combined). Sequence data analyses were mainly performed using Quantitative In-sights in the Microbial Ecology software package (QIIME) and R packages (v3.2.0). Heatmapping was performed to present the colonic bacteria based on the relative abundance of OTUs by the gplots (https://cran.r-project.org/web/packages/gplots/index.html) R package. Within sample diversity (alpha diversity) was calculated using Shannon, Simpson, Chao1, and ACE metrics. Beta diversity analysis was performed to investigate the structural variation of microbial communities and visualized via principal component analysis (PCA) with UniFrac distance. The taxonomy compositions and abundances were visualized using MEtaGenome Analyzer (MEGAN), and Graphical Phylogenetic Analysis (GraPhlAn).21,22 Taxa abundances at the phylum and genus levels were statistically compared among samples or groups by Metastats23 and visualized as a histogram. PICRUSt2 was used to predict the abundance of sample function, and MetaCyc (https://metacyc.org/) databases were used to predict the microbial function and related pathways.
Statistical analysis
The experiments were performed in triplicate, and the results were expressed as the mean ± standard deviations (SD). Statistical analysis was performed with GraphPad Prism 6 (GraphPad Software, Inc., California, USA). Differences in experimental data, such as SCFAs produced at each time point among substrates, were analyzed by one-way analysis of variance (ANOVA) using IBM SPSS 25.0 (SPSS Inc., Chicago, USA), and the means were separated using Duncan's multiple range test (p < 0.05).
Results and discussion
Effect of okara-HPIDF on DSS-induced acute ulcerative colitis in C57BL/6 mice
The bodyweight changes of all the experimental animals are shown in Fig. 1B. The bodyweights of the DSS-treated mice (DSS, DSS + HPIDF, DSS + LPDF groups) were lower than those of the mice without DSS treatment (control, HPIDF groups) from day 6 (p < 0.05). On the last day of feeding, although the difference was not significant, the bodyweight of the DSS + HPIDF group was slightly higher than that of the DSS + LPDF group; meanwhile, both showed a minor higher bodyweight than in the DSS group. During feeding, the bodyweight of the HPIDF group was slightly lower than that of the control group, both without DSS treatment. This situation reflected the effect of HPIDF intake on bodyweight control.
The food ration changes of all the experimental animals are shown in Fig. 1C. In the first three days of treatment, the food intake of the experimental animals was not stable, which may be due to the experimental animals needing time to adapt to the environment and the gavage operation. The food ration of the DSS-treated mice (DSS, DSS + HPIDF, DSS + LPDF groups) was lower than that of the mice without DSS treatment (control, HPIDF groups) from day 5 (p < 0.05). Although there was no standard deviation in the results for the food ration, the intake of HPIDF had little effect on food ration, which was the same in the DSS-treated mice (DSS, DSS + HPIDF, DSS + LPDF groups) and the mice without DSS treatment (control, HPIDF groups). Colitis is often accompanied by rapid weight loss,24 with ulcerative colitis caused by rapid peristalsis of the colon, manifested as diarrhea, which affects the absorption of nutrients and inorganic salts, leading to weight loss.25 In addition, systemic inflammatory reactions also increase the consumption of nutrition and cause weight loss.26 The occurrence of colitis in the experimental animals in this study was completely consistent with the above.
Disease activity index (DAI) is a comprehensive score based on the percentage of weight loss, fecal status, and bloody stool to comprehensively evaluate the physical state of the experiments animals. The DAI index changes of the experimental animals during treatment are shown in Fig. 1D. On the sixth day, the index of the tested animals began to show significant differences. At the end of the experiment (day 9), the DSS-treated mice (DSS, DSS + HPIDF, DSS + LPDF groups) showed a high DAI index, but the DSS + HPIDF and DSS + LPDF groups were slightly lower than the DSS group; meanwhile, there were no differences between the DSS + HPIDF and DSS + LPDF groups. This result showed that the intake of HPIDF inhibited body damage to a certain extent, which could help the body reduce diarrhea and bloody stools occurring during ulcerative colitis.
Effect of okara-HPIDF on colitis in C57BL/6 mice
The colonic state and H&E staining of the colons of the experimental animals are shown in Fig. 2A. The colon lengths are shown in Fig. 2B. As shown, the colon length with DSS treatment (DSS, DSS + HPIDF, DSS + LPDF groups) was significantly shorter than that in the other groups (p < 0.05), but the effect of different dietary fibers on colon length was not significant under the influence of DSS (p > 0.05). From the perspective of colon length, the intake of HPIDF can enhance colon health but cannot alleviate colitis during DSS treatment.
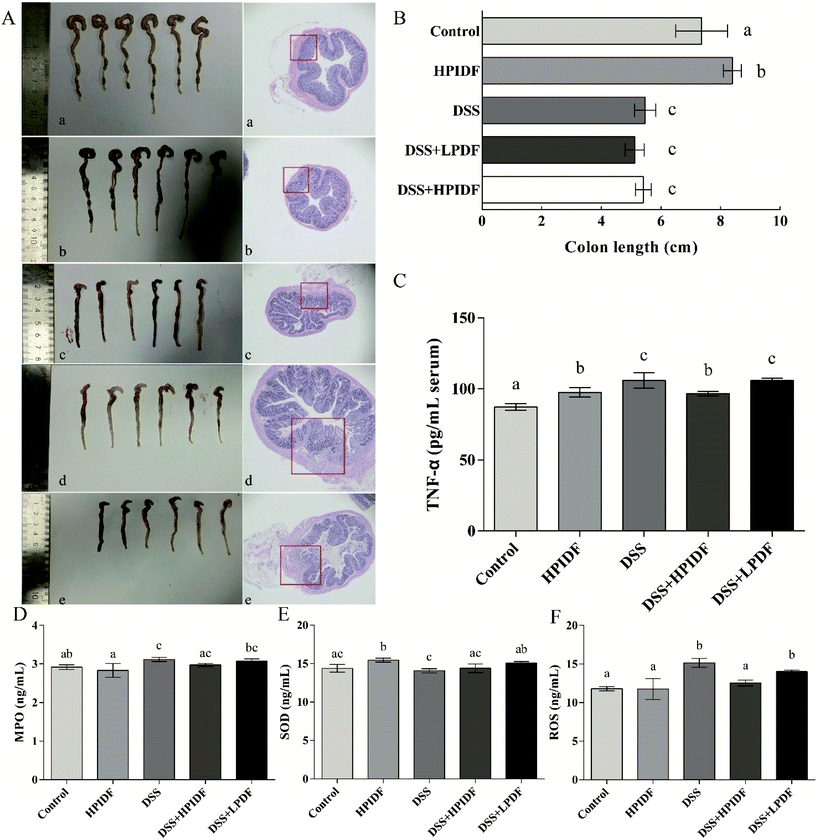 |
| Fig. 2 Colonic state and the levels of inflammation in the experimental animals. (n = 6). * (A) Colonic state and H&E (a: control group; b: HPIDF group; c: DSS group; d: DSS + HPIDF group; e: DSS + LPDF group). (B) Colon length of the experimental animals. (C) ELISA analysis of TNF-α in the experimental animals. (D) ELISA analysis of MPO in the experimental animals. (C) ELISA analysis of SOD in the experimental animals. (C) ELISA analysis of ROS in the experimental animals. Different lowercase letters mean significant difference (p < 0.05). | |
According to the colonic state and H&E staining, when assessed for tissue inflammation and damage at the experimental endpoint (Fig. 2A), dietary fiber intake (DSS + HPIDF, DSS + LPDF) seemed not to alleviate colitis. Generally, DSS treatment resulted in increased leukocyte infiltration and damage to the epithelium, where the structure of the colon was destroyed, with colonic bleeding being the most serious. The same happened in the DSS, DSS + HPIDF and DSS + LPDF (red box in Fig. 2A) groups, in which the colons were damaged to a serious degree. However, although the degree of colon damage was similar, the colonic bleeding was lighter in the DSS + HPIDF group (Fig. 2A-d vs. c). From the tissue section, we could not determine which group of colitis was the lightest.
Effect of DF intake on inflammatory factors
TNF-α is a type of tumor necrosis factor, which is usually used to measure the level of inflammation, which is also an important serological indicator to measure the severity of colitis. Because we were unable to assess the degree of colitis in each group, the content of TNF-α was used to measure the level of inflammation. As shown in Fig. 2C, the higher the content of TNF-α, the more severe the inflammation. The DSS group showed the highest level of TNF-α (105.96 pg mL−1 serum), which was significantly higher than that in the other groups (p < 0.05). The content of TNF-α in the DSS + HPIDF group (96.77 pg mL−1 serum) was also lower than that in the DSS + LPDF group (105.93 pg mL−1 serum) (p < 0.05). Meanwhile, there was no significant difference between the DSS and DSS + LPDF groups (p > 0.05).
Besides the level of TNF-α in serum, the levels of MPO, SOD, and ROS in the colonic tissues were also determined by ELISA. The results are shown in Fig. 2D–F. On the whole, there were some differences among the indicators of each group, but the ranges were minor. As shown in Fig. 2D, the intake of HPIDF (HPIDF group) decreased the level of MPO (2.83 ng mL−1) compared with the control (2.92 ng mL−1) with no significant differences (p > 0.05). With the production of inflammation, the level of MPO was also increased (DSS group: 3.12 ng mL−1, p < 0.05). In this case, the intake of HPIDF reduced MPO levels to a small extent (DSS + HPIDF group: 2.97 ng mL−1) with no significant differences (p > 0.05). In Fig. 2F, the intake of HPIDF (HPIDF group) increased the level of SOD (15.46 ng mL−1) compared with the control (14.38 ng mL−1), with significance (p < 0.05). The level of SOD in the DSS + HPIDF group showed a minor increase (14.40 ng mL−1) compared with the DSS group (14.07 ng mL−1) with no significant difference (p > 0.05). In short, the intake of DF had a minor effect on the levels of MPO and SOD in colitis tissues.
As for the level of ROS (Fig. 2F), there were significant differences among the five groups. The DSS group showed the highest level of ROS (15.14 ng mL−1), which is a typical case of inflammatory damage to the body. Meanwhile, the intake of HPIDF (DSS + HPIDF group: 12.53 ng mL−1) could decrease the ROS content to a normal level compared with the control group (11.80 ng mL−1). In contrast, the intake of LPDF (DSS + LPDF group: 14.01 ng mL−1) had less effect on the ROS level.
According to the content of inflammatory factors, the intake of HPIDF ameliorated colitis to a certain extent, which was consistent with our expected results. However, compared with other studies, the level of inflammatory factors in the tested animals recorded low changes, which might be due to the short test cycle. In future research, we will continue to observe the changes in these indicators. To further explore the effect of HPIDF intake on colon health, we comprehensively evaluated the colonic environment of the experimental animals.
Production of short-chain fatty acids (SCFAs) in the colon
The composition and content of SCFAs in the colon are shown in Fig. 3. As shown, the composition of SCFAs was significantly different in the different groups. For healthy mice (control and HPIDF groups), the intake of HPIDF significantly increased the content of propionic acid in the colon (p < 0.05); meanwhile the content of butyric acid decreased (p < 0.05). The intake of DSS reduced the content of SCFAs in the colon significantly (p < 0.05), regardless of the dietary intake. The content of the total SCFAs in the DSS + HPIDF group was the lowest, which proved that the intake of HPIDF significantly inhibited the production of SCFAs. Compared with the DSS and DSS + LPDF groups, the DSS + HPIDF group showed a significant decrease in the content of acetic acid and propionic acid (p < 0.05) with the disappearance of other SCFAs (Fig. 3B). The huge differences in SCFAs’ composition among the different groups may be the key reason for the colitis situation.
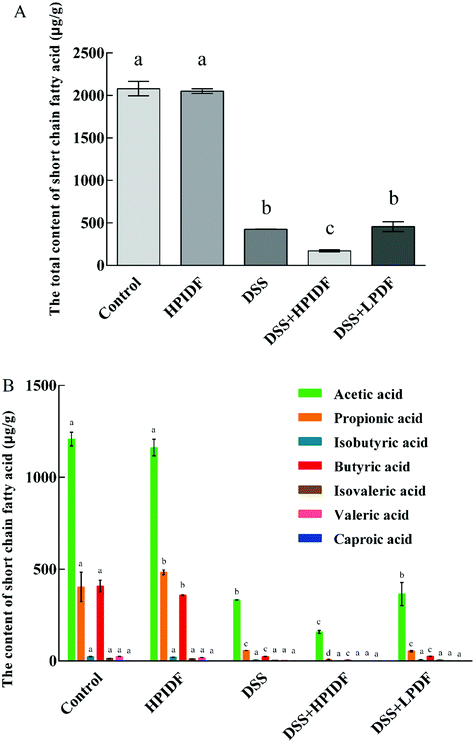 |
| Fig. 3 Content of short-chain fatty acids in the colon (n = 6). * (A) Total amount of SCFAs in the different experimental groups; (B) composition of SCFAs in the different experimental groups; different lowercase letters mean significant difference in the columns with the same color (p < 0.05). | |
Propionic acid and butyric acid are the two most reported SCFAs, which are positively related to intestinal health.27–29 Propionate significantly stimulated the release of PYY and GLP-1 from human colonic cells.30 Thus, the relationship between propionic acid, the intestinal tract, and lipid metabolism was established. In addition, propionic acid can inhibit the activity of HDACs to some extent with an anti-inflammatory or immunosuppressive function.31 Butyric acid, a typical gut bacteria metabolite, lowers arterial blood pressure via colon–vagus nerve signaling.32 Its derivatives can also accelerate the apoptosis of cancer cells,29 while it can enhance the activities of some digestive enzymes and promote the proliferation of probiotics, to enhance the body's immune and digestive function.33
The synthesis of three SCFAs with a significant difference in this study was clarified basically:34 acetic acid is produced from pyruvate by gut bacteria either via acetyl–CoA or the Wood–Ljungdahl pathway, meanwhile, acetate is synthesized via two branches: C1-body branch (the Eastern branch) and the carbon monoxide branch (the Western branch). Related microorganisms include Akkermansia muciniphila, Bacteroides spp., Bifidobacterium spp., Prevotella spp., Ruminococcus spp., Blautia hydrogenotrophica, Clostridium spp., and Streptococcus spp. Propionic acid is produced from succinate conversion to methylmalonyl–CoA via the succinate pathway. Propionate is synthesized from acrylate with lactate as a precursor through the acrylate pathway and the propanediol pathway, in which deoxyhexose sugars are substrates. Related microorganisms include Bacteroides spp., Phascolarctobacterium succinatutens, Dialister spp., Veillonella spp. Megasphaera elsdenii, Coprococcus catus, Salmonella spp., Roseburia inulinivorans, and Ruminococcus obeum. Butyric acid is formed from the condensation of two molecules of acetyl–CoA and the subsequent reduction to butyryl–CoA, which can be converted to butyrate by phosphor–transbutyrylase and butyrate kinase. Related microorganisms include Coprococcus comes, Coprococcus eutactus, Anaerostipes spp., Coprococcus catus, Eubacterium rectale, Eubacterium hallii, Faecalibacterium prausnitzii, and Roseburia spp. In addition, some microbes in the gut can use acetic acid and lactate to synthesize butyric acid to stabilize the intestinal environment.35
In summary, the change of SCFAs’ composition and content was the direct reason for the differences in colonic inflammation. However, the fundamental reason was the change in the gut bacteria after the intake of okara-IDF. The analysis of bacteria is necessary to explain the changes in the colonic environment in this work.
Effect of DF on bacterial diversity in DSS-induced C57BL/6 mice
The microbiota in the colon contents was analyzed by high-throughput sequencing spanning the 16S rDNA V3–V4 hypervariable region. The Chao1 and Observed species values showed that the richness of the gut microbiota in the DSS, DSS + HPIDF, and DSS + LPDF groups was decreased significantly (Fig. 4A). Moreover, the intake of okara-IDF did not affect the richness of the gut microbiota (control vs. HPIDF, DSS vs. DSS + HPIDF vs. DSS + LPDF, p < 0.05). The Shannon index and Simpson index showed the variety of the gut microbiota, the results are similar to the richness (Fig. 4A). These results all indicated that colitis decreased in higher gut microbiota richness. However, different from other studies,36 the intake of okara-IDF did not increase the total number of gut microbiota. We can draw an unforeseen conclusion that the intake of high-purity dietary fiber did not affect the total number of gut microbiota significantly during DSS-induced colitis, at least, okara-HPIDF/LPDF did not. Beta diversity indices showed the same (Fig. 4B), where the microbial composition of the control group and HPIDF group was similar, while the DSS, DSS + HPIDF, and DSS + LPDF groups were similar to each other, but there was a great difference between the healthy group and the treatment group.
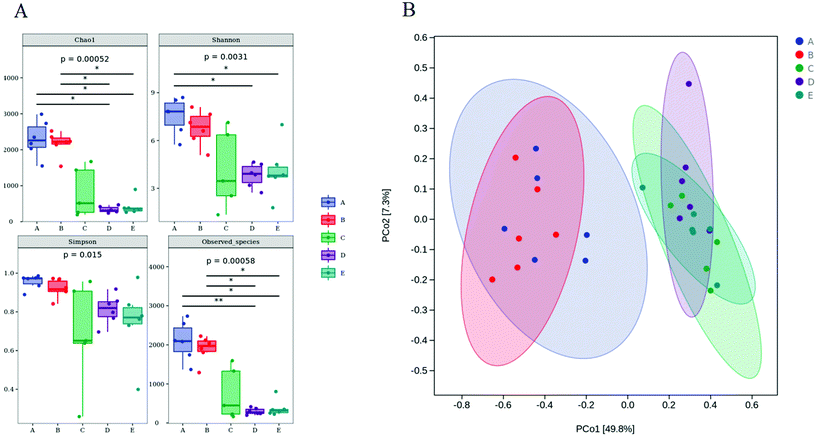 |
| Fig. 4 Species diversity analysis of each group of mice (genus level, n = 6). * (A) Boxplots of the alpha diversity indices. (B) Beta diversity indices (weighted unifrac). (A: Control group; B: HPIDF group; C: DSS group; D: DSS + HPIDF group; DSS + LPDF group). | |
Change in the gut microbiota structure caused by the intake of okara-HPIDF
To assess beta diversity, we performed principal component analysis (PCA) between the gut samples (Fig. 5A). The PCA results showed that the control and HPIDF groups were clustered together and were separate from the colitis model groups (DSS, DSS + HPIDF, and DSS + LPDF groups), thus indicating significant differences in the effects on gut microbiota in the healthy groups and colitis groups.
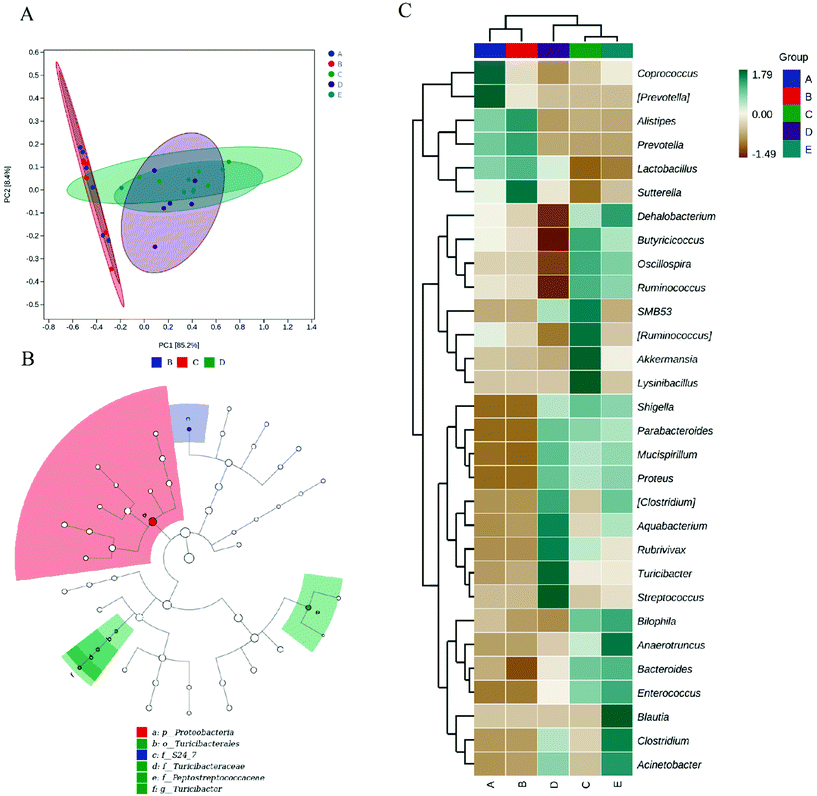 |
| Fig. 5 Species difference and characteristic species analysis from 16S rRNA gene sequencing of the gut microbiota (genus level, n = 6). * (A) PCA analysis; (B) LEfSe analysis (LDA threshold > 4); (C) Heatmap (top 30). (A: Control group; B: HPIDF group; C: DSS group; D: DSS + HPIDF group; DSS + LPDF group.) | |
The LEfSe algorithm (Fig. 5B) detected 3 genera with differential abundance as biomarkers. All of the biomarkers had high LDA scores (LDA > 4.0, alpha value according to the factorial Kruskal–Wallis test < 0.01), which reflected the differences in the abundances of bacterial communities with biological significance. The representative bacterial genera with variations in abundance included S24_7, Turicibacteraceae, and Peptostreptococcaceae. S24_7 is defined as Candidatus Homeothermaceae, which has been reported to have remarkable characteristics in the fermentation process of plant glycan (hemicellulose and pectin), host glycan, and α-glucan.37Turcicacteraceae has been reported to be associated with lipid metabolism.38Peptostreptococcaceae is an anaerobic bacterium with a fermentation metabolism,39 which was also closely related to the fermentation of DF. This phenomenon explained why the significance appears in the DSS, HPIDF, and DSS + HPIDF groups.
To further identify the microbial community changes at the OTU level after the intake of okara-HPIDF, the relative abundance of key OTUs is presented by a heatmap (Fig. 5C). Compared with the DSS group, it was obvious that the DSS + HPIDF group resulted in a promotion of the relative abundances of 10 OTUs (Lactobacillus, Parabacteroides, Aquabacterium, Rubrivivax, Acinetobacter, Turicibacter, Streptococcus, Clostridium, Mucispirillum, Proteus), and decreased the relative abundances of 14 OTUs (Coprococcus, Dehalobacterium, Butyicicoccus, SMB53, Lysinibacillus, Bilophila, Oscillospira, Ruminococcus, Akkermansia, Ruminococcus, Shigella, Anaerotruncus, Bacteroides, Enterococcus).
To elucidate the effects of different treatments on the microbiota composition, we determined the relative abundance (Fig. 6), and the ratio of microbiota at the phylum and genus levels (Table 1). Firmicutes and the Bacteroidetes are dominant in the normal gut microbiota, and the situation is the same in this study (Fig. 6A). Compared with the control group, the intake of HPIDF significantly increased the proportion of Bacteroides, with a decrease in Firmicutes. The increase in Bacteroides is beneficial to the excretion of harmful substances,40 which proved that the intake of HPIDF should not be harmful because no other bacteria proliferated excessively besides Firmicutes and Bacteroides. However in the colitis model group (DSS, DSS + HPIDF, and DSS + LPDF groups), the homeostasis of the gut environment was broken completely, showing a significant increase in Proteobacteria, and meanwhile a huge decrease in Bacteroides. It had been reported that Proteobacteria might exploit host defenses, drive proinflammatory change, and alter the intestinal microbiota in favor of dysbiosis,41 which is a typical case of colitis. In the DSS group, a large relative abundance of Verrucomicrobia appeared, while the content should be very low in the normal gut. In research on the intestinal environment, Verrucomicrobia has been rarely studied. However, we speculated that the increase in Verrucomicrobia was not related to the adverse effect of HPIDF on colitis, because, in the DSS + HPIDF group, Verrucomicrobia almost did not exist. Further study is needed on the mechanism of Verrucomicrobia in intestinal inflammation. In addition, the intake of HPIDF in the colitis model group promoted the relative abundance of Firmicutes, which is closer to that in a healthy gut, better than that in the DSS + LPDF group. From this perspective, the intake of HPIDF should positively affect acute ulcerative colitis, but the effect was not obvious or did not play a key role.
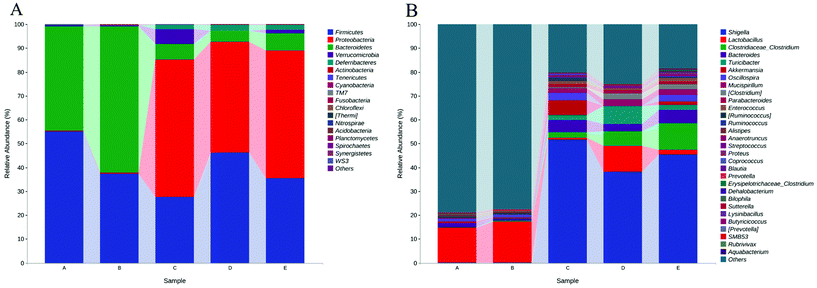 |
| Fig. 6 Relative abundances (top 30) of the gut microbiota at the bacterial (A) phylum (B) genus level. * (A) Control group; (B) HPIDF group; (C) DSS group; (D) DSS + HPIDF group; DSS + LPDF group. | |
Table 1 Ratio of microbiota (top 10) at the phylum and genus levels (%) (n = 6)
Taxonomy |
Control |
HPIDF |
DSS |
DSS + HPIDF |
DSS + LPDF |
Phylum |
Firmicutes
|
55.17 |
37.30 |
27.68 |
46.29 |
35.46 |
Proteobacteria
|
0.28 |
0.44 |
57.41 |
46.39 |
53.54 |
Bacteroidetes
|
43.62 |
61.46 |
6.76 |
4.52 |
7.09 |
Verrucomicrobia
|
0.58 |
0.43 |
6.04 |
0.05 |
1.58 |
Deferribacteres
|
0.09 |
0.02 |
1.91 |
2.63 |
2.21 |
Actinobacteria
|
0.03 |
0.06 |
0.00 |
0.02 |
0.01 |
Tenericutes
|
0.08 |
0.01 |
0.00 |
0.00 |
0.01 |
Cyanobacteria
|
0.00 |
0.00 |
0.03 |
0.00 |
0.03 |
TM7
|
0.00 |
0.02 |
0.00 |
0.00 |
0.00 |
Fusobacteria
|
0.00 |
0.01 |
0.00 |
0.01 |
0.00 |
Genus |
Shigella
|
0.03 |
0.04 |
51.79 |
38.28 |
45.43 |
Lactobacillus
|
14.71 |
17.33 |
0.57 |
10.67 |
1.80 |
Clostridiaceae/Clostridium |
0.19 |
0.29 |
2.39 |
6.16 |
11.31 |
Bacteroides
|
1.78 |
0.63 |
5.10 |
2.92 |
5.52 |
Turicibacter
|
0.00 |
0.33 |
2.09 |
7.66 |
2.06 |
Akkermansia
|
0.58 |
0.43 |
6.04 |
0.05 |
1.58 |
Oscillospira
|
1.23 |
1.21 |
3.27 |
0.01 |
2.77 |
Mucispirillum
|
0.09 |
0.02 |
1.91 |
2.63 |
2.21 |
Clostridium
|
0.00 |
0.00 |
0.47 |
2.65 |
2.25 |
Parabacteroides
|
0.04 |
0.04 |
1.30 |
1.44 |
1.12 |
At the genus level, the intake of HPIDF increased a small amount of Lactobacillus in the healthy group (control and HPIDF groups, p < 0.05). In the colitis model group, the intake of HPIDF could still maintain the existence of Lactobacillus (Fig. 6B). However, DSS resulted in a significant increase (p < 0.05) in the relative abundance of Shigella, which can produce toxins and attack colonic epithelium, leading to colon diseases.42 Compared with the DSS and DSS + LPDF groups, the intake of HPIDF significantly inhibited the relative abundance of Shigella (p < 0.05). The intake of HPIDF also led to an increase in Turiciacter, which has been reported to be important for host lipid and steroid metabolism. This may also explain the lipid-lowering mechanism of HPIDF.43 In this respect, the intake of HPIDF is still helpful to the recovery of colitis. However, the changes in the environment of the colon are complex. We also observed that for Bacteroides, a group of microorganisms involved in glucose metabolism that may be related to immune function, their relative abundance was less in the DSS + HPIDF group. Moreover, at the genus level, there are much uncertain flora, which may be associated with the changes of colitis. Fig. 7 depicts a phylogenetic classification tree. The outer ring is a heat map. Each group corresponds to a color whose intensity varies with species abundance. The abundance results were consistent with those before.
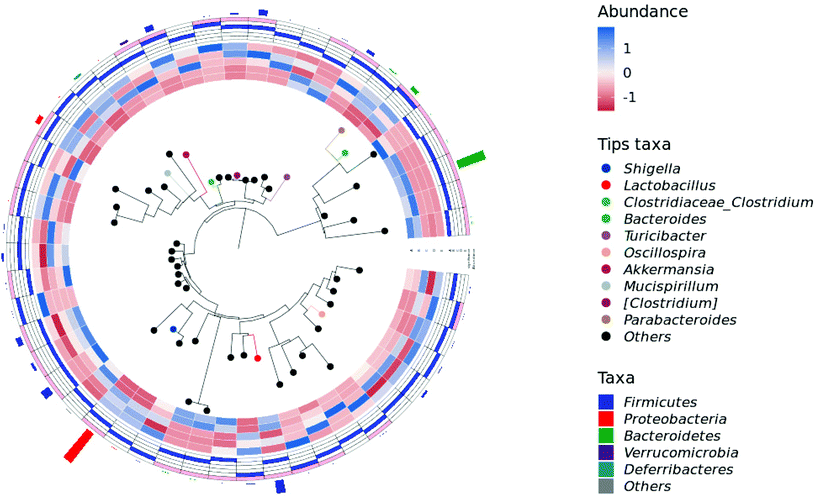 |
| Fig. 7 Phylogenetic classification tree. | |
Our previous research results showed that okara-HPIDF is a kind of insoluble dietary fiber mainly composed of hemicellulose; meanwhile, it does not change greatly with the digestive environment.15 Therefore, we speculated that the fermentation mode of okara-HPIDF in vivo should be similar to hemicellulose. Hemicelluloses are large polymeric molecules when they enter the colon, and this mode of fermentation is called the cross-feeding effect, in which one bacteria liberates smaller parts of polymers that then favor other bacteria.44 We do not rule out that HPIDF has the same fermentation mode.
Analysis of the metabolic pathway differences
After obtaining the abundance data of the metabolic pathways dependent on the PICRUSt2 (Phylogenetic Investigation of Communities by Reconstruction of Unobserved States), we tried to find metabolic pathways with significant differences between groups by comparing with the KEGG Pathway database (http://www.genome.jp/kegg/pathway.html). We did not find a metabolic pathway with a significant difference between the HPIDF and control groups, but found a significant difference between the DSS + HPIDF and DSS groups (Fig. 8A): PWY-2941 pathway and AEROBACTINSYN-PWY pathway. Both of the two pathways are lacking relevant study. The PWY-2941 pathway is related to lysine biosynthesis, and the AEROBACTINSYN-PWY pathway is related to Proteobacteria biosynthesis.45,46
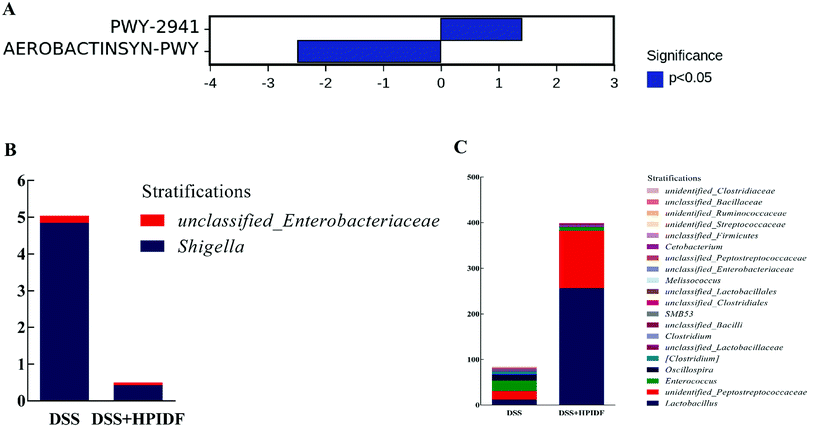 |
| Fig. 8 Analysis of the metabolic pathway differences. * (A) Metabolic pathways with significant difference between the DSS + HPIDF and DSS groups; (B) abundance of bacteria in the AEROBACTINSYN-PWY pathway with significant difference; (C) abundance of bacteria in the PWY-2941 pathway with significant difference. | |
Through the analysis of species composition in metabolic pathways (Fig. 8B and C), the abundance of Shigella in the DSS + HPIDF group was found to be significantly lower than that in the DSS group (p < 0.05); meanwhile the abundances of Lactobacillus and Peptostreptococcaceae were higher than that in the DSS group, with significance (p < 0.05). These findings were almost consistent with the previous results of the relative abundance of the gut microbiota. As reported, Shigella reduced the content of SCFAs in the colon significantly.47 Besides, we have already mentioned that Lactobacillus is an important source of propionic acid (lactic acid is the raw material of propionic acid).35 The increase in Peptostreptococcaceae is also due to the increased amount of total SCFAs.48
Since HPIDF enters the gut, all indicators indicated that it should relieve acute ulcerative colitis. Then the reason why the intake of HPIDF did not relieve the colonic injury is only possible to speculate: acute ulcerative colitis is a severe disease, and the intake of HPIDF was unable to maintain the homeostasis of the colonic environment. The intake of HPIDF could not change the main abundance of Shigella in colitis. This showed that in the process of acute ulcerative colitis, the effect of insoluble dietary fiber on the body is incidental. This is not the first time such a proposal has appeared.
Conclusion
This study focused on the changes in the colonic environment after the intake of okara-HPIDF. In summary, we put forward three viewpoints. First, the intake of okara-HPIDF reduced colitis slightly, affecting the proliferation of the key bacteria in the PWY-2941 pathway and AEROBACTINSYN-PWY pathway, again affecting the synthesis of SCFAs, which provides a positive role for colon health. Second, although okara-HPIDF has a positive effect, the recommended intake content of okara-HPIDF is still unable to repair the injury caused by DSS-induced acute ulcerative colitis. Excessive intake should not be recommended either. The intake of insoluble dietary fiber should not be recommended for patients with acute ulcerative colitis. Finally, we put forward a hypothesis: perhaps the intake of okara-HPIDF will positively affect chronic ulcerative colitis because, in chronic trials, the internal environment of the colon will not be changed so dramatically. In addition, whether the daily use of okara-HPIDF can resist the formation of acute ulcerative colitis still needs to be further explored. Finally, the differences at the protein level caused by the change of colon environment will be work for the next stage.
Author contributions
Bo Lyu: Conceptualization, software, writing-original draft. Wang Yi: Visualization, writing-original draft. Fu Hongling: Methodology. Li Jiaxin: Investigation. Yang Xiaoqing: Investigation. Shen Yue: Methodology. Mohammed Sharif Swallah: Writing-original draft. Yu Ziyue: Visualization. Li Yang: Supervision. Huan Wang: Data curation, project administration. Hansong Yu: Funding acquisition, project administration. Lianzhou Jiang: Funding acquisition, project administration.
Conflicts of interest
There are no conflicts of interest to declare.
Acknowledgements
The authors would like to thank the earmarked fund for China Agriculture Research System of MOF and MARA (CARS-04); China Postdoctoral Science Foundation (2021M690944); Major Science and Technology Innovation Projects in Shandong Province (No. 2019JZZY010722); Heilongjiang Postdoctoral General Support Project (No. LBH-Z20089); The “Young Talents” Project of Northeast Agricultural University (No. 19QC29).
References
- M. S. Swallah, H. Fan, S. Wang, H. Yu and C. Piao, Prebiotic Impacts of Soybean Residue (Okara) on Eubiosis/Dysbiosis Condition of the Gut and the Possible Effects on Liver and Kidney Functions, Molecules, 2021, 26, 326 CrossRef CAS PubMed.
- A. Andoh, T. Tsujikawa and Y. Fujiyama, Role of dietary fiber and short-chain fatty acids in the colon, Curr. Pharm. Des., 2003, 9, 347–358 CrossRef CAS PubMed.
- F. Bishehsari, P. A. Engen, N. Z. Preite, Y. E. Tuncil, A. Naqib, M. Shaikh, M. Rossi, S. Wilber, S. J. Green and B. R. Hamaker, Dietary fiber treatment corrects the composition of gut microbiota, promotes SCFA production, and suppresses colon carcinogenesis, Genes, 2018, 9, 102 CrossRef PubMed.
- S. Ötles and S. Ozgoz, Health effects of dietary fiber, Acta Sci. Pol., Technol. Aliment., 2014, 13, 191–202 CrossRef.
- M. Krawczyk, D. Maciejewska, K. Ryterska, M. Czerwińka-Rogowska, D. Jamioł-Milc, K. Skonieczna-Żydecka, P. Milkiewicz, J. Raszeja-Wyszomirska and E. Stachowska, Gut permeability might be improved by dietary fiber in individuals with nonalcoholic fatty liver disease (NAFLD) undergoing weight reduction, Nutrients, 2018, 10, 1793 CrossRef PubMed.
- M. A. Pourhoseingholi, M. Vahedi and A. R. Baghestani, Burden of gastrointestinal cancer in Asia; an overview, Gastroenterol. Hepatol. Bed Bench, 2015, 8, 19 Search PubMed.
- B. O. Schneeman, Soluble vs insoluble fiber: different physiological responses, Food Technol., 1987, 41, 81–82 Search PubMed.
- O. Kanauchi, K. Mitsuyama, T. Homma, K. Takahama, Y. Fujiyama, A. Andoh, Y. Araki, T. Suga, T. Hibi and M. Naganuma, Treatment of ulcerative colitis patients by long-term administration of germinated barley foodstuff: multi-center open trial, Int. J. Mol. Med., 2003, 12, 701–704 CAS.
- O. Kanauchi, T. Oshima, A. Andoh, M. Shioya and K. Mitsuyama, Germinated barley foodstuff ameliorates inflammation in mice with colitis through modulation of mucosal immune system, Scand. J. Gastroenterol., 2008, 43, 1346–1352 CrossRef CAS PubMed.
- O. Kanauchi, T. Suga, M. Tochihara, T. Hibi, M. Naganuma, T. Homma, H. Asakura, H. Nakano, K. Takahama and Y. Fujiyama, Treatment of ulcerative colitis by feeding with germinated barley foodstuff: first report of a multicenter open control trial, J. Gastroenterol., 2002, 37, 67–72 CrossRef CAS PubMed.
- B. L. Wilberts, P. H. Arruda, J. M. Kinyon, T. S. Frana, C. Wang, D. R. Magstadt, D. M. Madson, J. F. Patience and E. R. Burrough, Investigation of the impact of increased dietary insoluble fiber through the feeding of distillers dried grains with solubles (DDGS) on the incidence and severity of Brachyspira-associated colitis in pigs, PLoS One, 2014, 9, e114741 CrossRef PubMed.
- J. P. Miles, J. Zou, M.-V. Kumar, M. Pellizzon, E. Ulman, M. Ricci, A. T. Gewirtz and B. Chassaing, Supplementation of low-and high-fat diets with fermentable fiber exacerbates severity of DSS-induced acute colitis, Inflamm. Bowel Dis., 2017, 23, 1133–1143 CrossRef PubMed.
- V. Singh, B. San Yeoh, R. E. Walker, X. Xiao, P. Saha, R. M. Golonka, J. Cai, A. C. A. Bretin, X. Cheng and Q. Liu, Microbiota fermentation-NLRP3 axis shapes the impact of dietary fibres on intestinal inflammation, Gut, 2019, 68, 1801–1812 CrossRef CAS PubMed.
- H. Armstrong, I. Mander, Z. Zhang, D. Armstrong and E. Wine, Not All Fibers Are Born Equal; Variable Response to Dietary Fiber Subtypes in IBD, Front. Pediatr., 2021, 8, 924 Search PubMed.
- L. Bo, W. Huan, M. S. Swallah, F. Hongling, S. Yue, G. Zengwang, T. Xiaohong, L. Yang, Y. Hansong and J. Lianzhou, Structure, Properties and Potential Bioactivities of High-purity Insoluble Fibre from Soybean Dregs (Okara), Food Chem., 2021, 130402 CAS.
- S. Wang, W. Sun, M. S. Swallah, K. Amin, B. Lyu, H. Fan, Z. Zhang and H. Yu, Preparation and characterization of soybean insoluble dietary fiber and its prebiotic effect on dyslipidemia and hepatic steatosis in high fat-fed C57BL/6J mice, Food Funct., 2021, 12(18), 8760–8773 RSC.
- S. Wirtz, C. Neufert, B. Weigmann and M. F. Neurath, Chemically induced mouse models of intestinal inflammation, Nat. Protoc., 2007, 2, 541 CrossRef CAS PubMed.
- J. Liu, Y. Chen, D. Liu, W. Liu, S. Hu, N. Zhou and Y. Xie, Ectopic expression of SIGIRR in the colon ameliorates colitis in mice by downregulating TLR4/NF-κB overactivation, Immunol. Lett., 2017, 183, 52–61 CrossRef CAS PubMed.
- L. Yang, Q. Lin, L. Han, Z. Wang, M. Luo, W. Kang, J. Liu, J. Wang, T. Ma and H. Liu, Soy hull dietary fiber alleviates inflammation in BALB/C mice by modulating the gut microbiota and suppressing the TLR-4/NF-κB signaling pathway, Food Funct., 2020, 11, 5965–5975 RSC.
- R. C. Edgar, Search and clustering orders of magnitude faster than BLAST, Bioinformatics, 2010, 26, 2460–2461 CrossRef CAS PubMed.
- D. H. Huson, S. Mitra, H.-J. Ruscheweyh, N. Weber and S. C. Schuster, Integrative analysis of environmental sequences using MEGAN4, Genome Res., 2011, 21, 1552–1560 CrossRef CAS PubMed.
- F. Asnicar, G. Weingart, T. L. Tickle, C. Huttenhower and N. Segata, Compact graphical representation of phylogenetic data and metadata with GraPhlAn, PeerJ, 2015, 3, e1029 CrossRef PubMed.
- J. R. White, N. Nagarajan and M. Pop, Statistical methods for detecting differentially abundant features in clinical metagenomic samples, PLoS Comput. Biol., 2009, 5, e1000352 CrossRef PubMed.
- Y. Kim, S. W. Hwang, S. Kim, Y.-S. Lee, T.-Y. Kim, S.-H. Lee, S. J. Kim, H. J. Yoo, E. N. Kim and M.-N. Kweon, Dietary cellulose prevents gut inflammation by modulating lipid metabolism and gut microbiota, Gut Microbes, 2020, 11, 944–961 CrossRef PubMed.
- E. Greig and G. I. Sandle, Diarrhea in ulcerative colitis: the role of altered colonic sodium transport, Ann. N. Y. Acad. Sci., 2000, 915, 327–332 CrossRef CAS PubMed.
- P. C. Calder, R. Albers, J.-M. Antoine, S. Blum, R. Bourdet-Sicard, G. Ferns, G. Folkerts, P. Friedmann, G. Frost and F. Guarner, Inflammatory disease processes and interactions with nutrition, Br. J. Nutr., 2009, 101, 1–45 CrossRef CAS PubMed.
- Z. Tian, X. Zhuang, M. Luo, W. Yin and L. Xiong, The propionic acid and butyric acid in serum but not in feces are increased in patients with diarrhea-predominant irritable bowel syndrome, BMC Gastroenterol., 2020, 20, 1–8 CrossRef PubMed.
- M. J. Ormsby, S. A. Johnson, N. Carpena, L. M. Meikle, R. J. Goldstone, A. McIntosh, H. M. Wessel, H. E. Hulme, C. C. McConnachie and J. P. Connolly, Propionic acid promotes the virulent phenotype of Crohn's disease-associated adherent-invasive Escherichia coli, Cell Rep., 2020, 30, 2297–2305 CrossRef CAS PubMed.
- L. Pattayil and H.-T. Balakrishnan-Saraswathi, In vitro evaluation of apoptotic induction of butyric acid derivatives in colorectal carcinoma cells, Anticancer Res., 2019, 39, 3795–3801 CrossRef CAS PubMed.
- E. S. Chambers, A. Viardot, A. Psichas, D. J. Morrison, K. G. Murphy, S. E. Zac-Varghese, K. MacDougall, T. Preston, C. Tedford and G. S. Finlayson, Effects of targeted delivery of propionate to the human colon on appetite regulation, body weight maintenance and adiposity in overweight adults, Gut, 2015, 64, 1744–1754 CrossRef CAS PubMed.
- M. Luu, H. Monning and A. Visekruna, Exploring the molecular mechanisms underlying the protective effects of microbial SCFAs on intestinal tolerance and food allergy, Front. Immunol., 2020, 11, 1225 CrossRef CAS PubMed.
- M. Onyszkiewicz, M. Gawrys-Kopczynska, P. Konopelski, M. Aleksandrowicz, A. Sawicka, E. Koźniewska, E. Samborowska and M. Ufnal, Butyric acid, a gut bacteria metabolite, lowers arterial blood pressure via colon-vagus nerve signaling and GPR41/43 receptors, Pfluegers Arch., 2019, 471, 1441–1453 CrossRef CAS PubMed.
- H. Aalamifar, S. Soltanian, A. Vazirzadeh, M. Akhlaghi, V. Morshedi, A. Gholamhosseini and M. Torfi Mozanzadeh, Dietary butyric acid improved growth, digestive enzyme activities and humoral immune parameters in Barramundi (Lates calcarifer), Aquacult. Nutr., 2020, 26, 156–164 CrossRef CAS.
- A. Koh, F. De Vadder, P. Kovatcheva-Datchary and F. Bäckhed, From dietary fiber to host physiology: short-chain fatty acids as key bacterial metabolites, Cell, 2016, 165, 1332–1345 CrossRef CAS PubMed.
- S. H. Duncan, A. Barcenilla, C. S. Stewart, S. E. Pryde and H. J. Flint, Acetate utilization and butyryl coenzyme A (CoA): acetate-CoA transferase in butyrate-producing bacteria from the human large intestine, Appl. Environ. Microbiol., 2002, 68, 5186–5190 CrossRef CAS PubMed.
- L. Yang, Y. Zhao, J. Huang, H. Zhang, Q. Lin, L. Han, J. Liu, J. Wang and H. Liu, Insoluble dietary fiber from soy hulls regulates the gut microbiota in vitro and increases the abundance of bifidobacteriales and lactobacillales, J. Food Sci. Technol., 2020, 57, 152–162 Search PubMed.
- K. L. Ormerod, D. L. Wood, N. Lachner, S. L. Gellatly, J. N. Daly, J. D. Parsons, C. G. Dal'Molin, R. W. Palfreyman, L. K. Nielsen and M. A. Cooper, Genomic characterization of the uncultured Bacteroidales family S24-7 inhabiting the guts of homeothermic animals, Microbiome, 2016, 4, 1–17 CrossRef PubMed.
- J. Sha, J. Zhang, B. Sui and C. Zhang, Effects of Aerobic exercise and polysaccharide compounds interventions on gut microbiota of the high fat diet induced obese rats, Chin. J. Sports Med., 2018, 37, 328–336 Search PubMed.
-
A. Slobodkin, 24 The Family Peptostreptococcaceae, 2014 Search PubMed.
- H. M. Wexler, Bacteroides: the good, the bad, and the nitty-gritty, Clin. Microbiol. Rev., 2007, 20, 593–621 CrossRef CAS PubMed.
- I. Mukhopadhya, R. Hansen, E. M. El-Omar and G. L. Hold, IBD—what role do Proteobacteria play?, Nat. Rev. Gastroenterol. Hepatol., 2012, 9, 219 CrossRef CAS PubMed.
- T. L. Hale, Genetic basis of virulence in Shigella species, Microbiol. Rev., 1991, 55, 206–224 CrossRef CAS PubMed.
- B. Wang, H. Yu, Y. He, L. Wen, J. Gu, X. Wang, X. Miao, G. Qiu and H. Wang, Effect of soybean insoluble dietary fiber on prevention of obesity in high-fat diet fed mice via regulation of the gut microbiota, Food Funct., 2021, 12(17), 7923–7937 RSC.
- B. R. Hamaker and Y. E. Tuncil, A perspective on the complexity of dietary fiber structures and their potential effect on the gut microbiota, J. Mol. Biol., 2014, 426, 3838–3850 CrossRef CAS PubMed.
- C. M. O'Donovan, S. M. Madigan, I. Garcia-Perez, A. Rankin, O. O'Sullivan and P. D. Cotter, Distinct microbiome composition and metabolome exists across subgroups of elite Irish athletes, J. Sci. Med. Sport, 2020, 23, 63–68 CrossRef PubMed.
-
A. D. C. Fernandes, Characterization of microbiome in Lisbon subway, 2016 Search PubMed.
- G. Rabbani, M. J. Albert, A. H. Rahman, M. M. Isalm, K. N. Islam and K. Alam, Short-chain fatty acids improve clinical, pathologic, and microbiologic features of experimental shigellosis, J. Infect. Dis., 1999, 179, 390–397 CrossRef CAS PubMed.
- Y. Zhu, Q. Niu, C. Shi, J. Wang and W. Zhu, The role of microbiota in compensatory growth of protein–restricted rats, Microb. Biotechnol., 2017, 10, 480–491 CrossRef CAS PubMed.
|
This journal is © The Royal Society of Chemistry 2022 |
Click here to see how this site uses Cookies. View our privacy policy here.