Aerobic biotransformation of a novel highly functionalized polyfluoroether-based surfactant using activated sludge from a wastewater treatment plant†
Received
26th August 2021
, Accepted 13th December 2021
First published on 14th December 2021
Abstract
A replacement fluorosurfactant has been recently introduced to the European market as an alternative to other per- and polyfluoroalkyl substances (PFAS) that have been phased-out or banned. Here, we incubated this novel fluorosurfactant (diFESOS, [F7C3OCHFCF2SCH2CH2OC(O)]2C2H3SO3−) which contains ether and thioether insertions, and its known polyfluoroalkyl degradation products, an alcohol (FESOH) and carboxylic acid (FESCA), with activated sludge from a wastewater treatment plant under sulfur-limited conditions. Dosed chemicals and their transformation products were monitored using ultra-high performance liquid chromatography-tandem mass spectrometry, and gas chromatography-mass spectrometry. In addition to FESOH and FESCA, two smaller metabolites were identified: C3F7OCHFCOO− (2H-3:2 PFECA) and perfluoropropanoic acid (PFPrA). 2H-3:2 PFECA presumably was a result of S-dealkylation of FESCA, which then resulted in the abiotic cleavage of two C–F bonds; no S-oxygenation was observed. Overall, the terminal products of this biotransformation likely have lower bioaccumulation potential than the parent fluorosurfactant based on comparison to other similar PFAS.
Environmental significance
A main concern about many per- and polyfluoroalkyl substances (PFAS) is their environmental persistence. A replacement PFAS fluorosurfactant designed with the intent to degrade in the environment has been recently introduced to the European market. Here, we investigated the fate of this novel fluorosurfactant called diFESOS, [F7C3OCHFCF2SCH2CH2OC(O)]2C2H3SO3−, and it's known polyfluoroalkyl degradation products in activated sludge from a wastewater treatment plant. The transformation proceeds through two larger metabolites, an alcohol and a carboxylic acid. We identified smaller, less fluorinated transformation products C3F7OCHFCOO− (2H-3:2 PFECA) and perfluoropropanoic acid (PFPrA), suggesting that diFESOS degrades to less bioaccumulative molecules in the environment. This is the first report investigating the microbial biotransformation of this commercial surfactant, and gives insight on what functional groups in a polyfluoroalkyl molecule can facilitate transformation in the environment.
|
Introduction
Fluorine-containing surfactants have been widely used since the 1950s due to their high-performance capabilities,1 but there is increasing concern over their persistence, bioaccumulation, and toxicity.2,3 In fact, many organic molecules containing a perfluoroalkyl moiety, collectively known as per- and polyfluoroalkyl substances (PFAS), have been of increasing concern. PFAS have a variety of applications: some PFAS are direct-use surfactants, some are residuals or processing agents used for the synthesis of commercial products, often polymers,1 while some have no direct applications and are transformation products or byproducts of other PFAS.4–6 Perfluorooctane sulfonate (PFOS) and its related chemicals were voluntarily phased out of production by some major manufacturers7 and since 2009, have been listed under Annex B of UNEP's Stockholm Convention on Persistent Organic Pollutants, however, there are accepted uses and specific exemptions including in insect baits with sulfluramid, metal plating in closed-loop systems and some exemptions for fire-fighting foams for liquid fuel fires (i.e. aqueous film forming foams, AFFF).2 Perfluoroalkyl carboxylates with eight or more carbon atoms (i.e. perfluorooctanoic acid, PFOA or larger) have been phased out of production in some countries, such as the USA and Canada.8,9 The long-chain carboxylate regulation includes fluorosurfactants that are precursors, forming perfluoroalkyl acids in the environment via biotic and abiotic transformations (reviewed in ref. 4–6). In 2019, PFOA was added to UNEP's Stockholm Convention on Persistent Organic Pollutants under Annex A, meaning its use is to be eliminated.3 This regulation includes PFOA itself, its salts, and any PFOA-related compounds, which are identified as anything containing a linear or branched moiety containing C7F15.3
Replacements for PFOS and long-chain perfluoroalkyl carboxylates generally fall into two categories: chemistries based on shorter perfluoroalkyl chains such as perfluorobutyl sulfonyl-based chemistries and perfluorohexane-based chemistries, or ether-based replacements (e.g. GenX, C3F7OCF(CF3)COOH).10 Short-chain perfluoroalkyl-based replacements typically form perfluorohexanoic acid and smaller acids as terminal degradation products, or perfluorobutane sulfonic acid. These short-chain acids are recalcitrant in the environment, similar to the long-chain acids,4,11 but are less bioaccumulative.12,13 For the ether replacements, there has been no evidence to show that inserting an ether in a perfluoroalkyl moiety enables environmental degradation, and most replacements appear to have similar toxicities or bioaccumulation potential to the phased-out chemicals.14 Additionally, perfluoroalkyl ether acids do not appear to be transformed under oxidative chemical conditions, although polyfluoroalkyl ether acids with a –O–CHF– moiety are oxidized.15
A new fluorosurfactant with a different polyfluoroalkyl backbone came to the market in Europe in late 2018.16 The structure of this fluorosurfactant, [C3F7OCHFCF2SCH2CH2OC(O)]2C2H3SO3− is drawn in Table 1 and is referred to with the abbreviation diFESOS. It contains a three-carbon perfluoroalkyl group, and an ether linkage within the polyfluoroalkyl chain, and is advertised as a wetting and leveling agent to replace other fluorosurfactants such as the fluorotelomer-based chemistries. As a direct-use surfactant, a major entry point into the environment is expected to be through municipal wastewater treatment plants. Our recent work investigating the reaction of FESOH (Table 1), an alcohol building block of diFESOS, with atmospheric oxidants resulted in the formation of perfluoropropanoic acid (PFPrA) and other small polyfluoroalkyl acids, including a five-carbon sulfonate C3F7OCHFCF2S(O)(O)OH (2H-3:2 PFESA) and a five-carbon carboxylic acid C3F7OCHFC(O)OH (2H-3:2 PFECA).17 In another recent study from our group, metabolism of diFESOS by Sprague-Dawley rats formed PFPrA and 2H-3:2 PFESA, but not 2H-3:2 PFECA.18
Table 1 Structures and names of dosed molecules
Name |
Structure |
Molecular identifiers |
diFESOS |
|
1,1,1,2,2,3,3,5,6,6,19,19,20,22,22,23,23,24,24,24-Icosafluoro-11,14-dioxo-4,10,15,21-tetraoxa-7,18-dithiatetracosane-12-sulfonic acid |
C18H14F20O9S3 |
MW 850.45 g mol−1 |
FESOH |
|
2-((1,1,2-Trifluoro-2-(heptafluoropropyloxy)ethyl)thio)ethan-1-ol |
C7H6F10O2S |
MW 344.17 g mol−1 |
FESCA |
|
2-((1,1,2-Trifluoro-2-(heptafluoropropoxy)ethyl)thio)acetic acid |
C7H4F10O3S |
MW 358.15 g mol−1 |
Wastewater treatment plant effluents can be point sources for contamination of anthropogenic chemicals to the environment, particularly for chemicals used in residential and commercial applications that are unable to be degraded by conventional wastewater treatment processes. Thus, it is important to assess the potential degradation of a replacement chemical in aerobic sludge with diverse microbial populations. The phased-out PFOS and long-chain perfluoroalkyl carboxylates are not transformed in wastewater treatment plant sludge, or in any known environmental compartment.19,20 In fact, the concentration of PFCAs and PFSAs often increases through a wastewater treatment plant as other PFAS are biologically transformed to these acids.21 Here, as the first microbial biotransformation test of diFESOS, we chose to perform the experiments under sulfur-limited conditions which creates desulfonation conditions where sulfur metabolism is enhanced, as has been done by other researchers studying polyfluorinated compounds containing sulfur atoms.22,23
The purpose of this work was to identify biotransformation products of diFESOS formed under aerobic conditions. This was done by incubating diFESOS with wastewater treatment plant sludge inoculate under sulfur-limited conditions to determine if it is transformed to non-bioaccumulative components. A small-scale test was also performed with unaltered mixed liquor from the wastewater treatment plant to confirm the same transformation products were formed. To further probe the biotransformation pathway, the alcohol degradation product FESOH and the carboxylic acid degradation product FESCA were also incubated with the wastewater treatment sludge under sulfur-limited conditions (see structures in Table 1). Since our previous work showed that reactions of FESOH with atmospheric oxidants17 and Sprague-Dawley rats18 resulted in the formation of perfluoropropanoic acid (PFPrA) and other small polyfluoroalkyl acids, we hypothesized that this will also occur under aerobic biotransformation conditions.
Materials and methods
Chemicals
diFESOS, a solid, and FESOH and FESCA, which are liquids, were supplied by Merck kGaA (Darmstadt, Germany). Some residual FESOH was present in the diFESOS standard, as described in the results and discussion. A description of all other chemicals used is provided in the ESI,† including preparation of sulfate-free defined media, Wolfe's minerals, and Pfennig's vitamins, based on previous investigations.22,23 Acetate and glucose were provided as the carbon and energy sources for microorganisms in the microcosms.
Microcosm setup
Mixed liquor was obtained from Ashbridge's Bay Wastewater Treatment Plant in downtown Toronto, Canada with a mixed liquor suspended solids (MLSS) concentration of 3420 mg L−1. A subsample of the mixed liquor was autoclaved to act as an inactive control. To prepare the sludge, live and autoclaved mixed liquor were each transferred to 50 mL centrifuge tubes and centrifuged at 6000 rpm for 20 minutes. The supernatant was discarded, and the tubes were topped up with sulfate-free medium to wash the sludge. After vortexing, the tubes were centrifuged again, and the supernatant was removed. Then, this process was repeated to wash the sludge again. Finally, the tubes were topped up with medium and were ready to be inoculated into the experimental bottles.
diFESOS and FESOH closed-system microcosms.
Closed-system microcosms were used to study diFESOS and FESOH to prevent the loss of the semi-volatile FESOH whether it was the test chemical or was formed from diFESOS metabolism. The closed-system microcosms were constructed similar to others, using 250 mL amber glass bottles with Mininert® valve closures.24 Three types of experiments were performed with each dosed chemical: with live sludge (n = 3), with autoclaved sludge as a sterile control (n = 2) and in media only as a control (n = 2). Bottles of sludge only without a dosed chemical were also monitored (n = 2) to assess if the sludge could be a source of background chemicals. The total volume of liquid added was 150 mL, allowing for 100 mL of headspace, to help maintain aerobic conditions. Washed sludge, either live or autoclaved, was added at 10% concentration, i.e. 15 mL. Wolfe's minerals and Pfenning's vitamins were also added to the bottles, and the total volume was brought to 150 mL with the sulfate-free media. Autoclaved sterile control bottles also received 0.75 mg mL−1 Hg2Cl2 to ensure they were sterile. The test chemicals were added at a concentration of 0.6 μM diFESOS and 1.2 μM FESOH and FESCA. diFESOS was dosed at half of the concentration of FESOH and FESCA because it contains two polyfluoroalkyl chains and was expected to break down to two molar equivalents of FESOH or FESCA. In this system, the substrates were acetate, glucose, ethanol, and the test chemicals. The bottles were gently inverted 10 times daily to maintain homogeneity without disrupting the microbial community. A detailed description of the components of each bottle is provided in the ESI.†
An additional experiment was performed where diFESOS was spiked into 3 bottles of raw, undiluted mixed liquor to determine if the same transformation products were formed, compared to sulfur-limited conditions.
A stainless-steel needle (6 in, 20 gauge) attached to a 1 mL disposable plastic syringe was inserted through the Mininert® valve to sample the liquid portion of the microcosms. To analyze acids via UPLC-MS/MS, 300 μL of the sample was mixed with 300 μL methanol and vortexed for 30 seconds, then centrifuged at 10
000 g for 6 minutes to remove any suspended solids. To analyze volatile chemicals via GC-MS, 300 μL of the sample was extracted into 600 μL ethyl acetate and vortexed for 30 seconds, and the supernatant was transferred to a vial for analysis. Samples were collected from the experimental bottles prior to adding the dosed chemicals, and then at 2 hours, and at 1, 2, 4, 7, 10, 14, 28 and 38 days. Every seven days oxygen concentrations were monitored by removing 200 μL of the headspace for analysis using gas chromatography with a thermal conductivity detector (GC-TCD) and 10 mL of pure oxygen was added into the microcosms via a gas-tight syringe. Full discussion on the oxygen content is included in the ESI.†
FESCA setup using flow-through microcosms.
A flow-through setup was used to study the biotransformation of FESCA to ensure aerobic conditions over a longer experimental timeframe. Preliminary tests in our lab suggested FESCA was not readily biodegradable, and as it is not volatile and not predicted to form any volatile transformation products, we chose to flow air through the experiments. The microcosms were prepared using the same components as in the original experiment, with a few changes to the setup. Firstly, the vessels used were 250 mL two-necked round-bottom flasks. A septum was added to one neck and air was continuously passed through a long needle, resulting in slight bubbling of the solution. The remaining neck was outfitted with a condenser to prevent loss of products. As FESCA is not volatile (unlike FESOH), we believed the use of a condenser would sufficiently prevent loss of aerosols formed via bubbling. The six condensers were connected in series to a chiller set to 5 °C. Secondly, 1.2 μM of 6:2 fluorotelomer unsaturated carboxylic acid (6:2 FTUCA; C6F17CH
CHCOOH) was added into each reaction vessel as a positive control within each experiment which will be metabolized if the system is viable.23 Three flasks were live experiments and three contained autoclaved sludge. The positive control under sterile conditions also confirmed that there was no significant loss of aerosols via bubbling. After 27 days, more 6:2 FTUCA in ethanol was spiked into the live experiments, and ethanol only was added to the autoclaved control experiments, providing an additional carbon source. An additional three media controls were prepared in glass bottles to confirm the stability of FESCA in the media. Samples were collected through disposable syringes using the long needles on days 1, 3, 7, 14, 28, 35, 42, 48, 56, 70, and 84 and were prepared for analysis as described above. An image of the set-up is shown in the ESI, Fig. S1.†
Targeted mass spectrometry analysis
LC-MS/MS analysis.
Targeted analysis was performed using a Waters Acquity UPLC coupled to a Waters Xevo TQ-S triple quadrupole mass spectrometer using negative mode electrospray ionization to quantify diFESOS, FESCA, 6:2 FTUCA, and their potential metabolites (details in ESI†). A Waters Acquity UPLC BEH C18 column (2.1 mm × 50 mm, 1.7 μm) was heated to 60 °C with a flow rate of 0.25 mL min−1. Solvent composition began at 95% A (2 mM ammonium acetate in water) and 5% B (methanol) and was held at those conditions until 2 min, when it was ramped to 80% B until 2.5 min. Between 2.5 and 4.5 min, B was increased to 100% and was held there until 6.0 min. At 6.1 min, the composition was returned to 5% B, for a total run time of 8 min. Sample injection volume was 2 μL. Samples were diluted 20x (or as needed) to quantify dosed chemicals, which were at a much higher concentration than most breakdown products. Complete details of mass spectrometry parameters are in the ESI, Table S4,† and target compounds included some proposed metabolites.
GC-MS analysis.
To quantify FESOH, an Agilent 7890A gas chromatograph with a 5975C inert XL EI/CI MSD mass spectrometer was operated using positive chemical ionization with methane as the reagent gas. An Agilent DB-1701 column was used (30 m × 0.25 mm, 0.15 μm) with helium as a carrier gas (1.2 mL min−1). The GC inlet was heated to 250 °C and was set to splitless mode, with the MSD transfer line set to 280 °C. The oven began at 80 °C and was held there for four minutes, then was ramped to 260 °C until 15 minutes and held at 260 °C until 18 minutes. Selected ion mode was used to monitor FESOH with a quantitation ion of [M + H-H2O]+ at m/z 327 and a qualitative ion of [M + H-2HF]+ at m/z 305, at a retention time of 9.2 minutes.
Suspect screening of other metabolites
LC-Orbitrap.
Select samples were injected onto a Thermo Q-Exactive Hybrid Quadrupole-Orbitrap mass spectrometer coupled to a Vanquish UHPLC system (Thermo Fisher Scientific, USA). The same chromatography, including the same column, was used as described for the LC-MS/MS triple quadrupole system above. Data were acquired using negative electrospray ionization in full scan mode from m/z 100–1000 with 70
000 resolution at m/z 200 with a maximum of 3 × 106 ions collected within 100 ms. Parameters used were −3.0 kV spray voltage, 30 L h−1 sheath gas flow rate, 6 L h−1 auxiliary gas flow rate and 300 °C capillary temperature. A suspect list of proposed metabolites was used for a specific subset of samples from the biotransformation experiments (see ESI, Table S5†). Fluoride could not be monitored as a degradation product due to the low concentrations of our test analytes in the system and high limits of detection of the ion chromatography system available to us.
Results and discussion
Degradation of diFESOS to FESCA
The incubation of diFESOS with activate sludge in a sulfate-free defined medium resulted in the transformation of diFESOS to FESOH and FESCA (Fig. 1). The diFESOS starting material contained FESOH as a residual from the synthetic process and at the 2 hours time point the diFESOS bottles contained 316 ± 17 nM FESOH, compared to 613 ± 118 nM diFESOS. The biotransformation of diFESOS to FESOH occurs via ester hydrolysis yielding two FESOH molecules for each diFESOS, similar to how one molecule of polyfluoroalkyl phosphate diesters (diPAPs) yields two molecules of fluorotelomer alcohols (FTOHs).25,26 Thus, the data presented in Fig. 1 are normalized to the molar concentration of diFESOS in the bottles at the 2 hours time point. The 1
:
2 molar ratio of metabolite formation from diFESOS was accounted for in this normalization. After 38 days, the remaining fraction of diFESOS was 0.34 ± 0.04 of the dosed concentration. Compared to diFESOS incubated with autoclaved sludge or in media only controls, the concentration of diFESOS in live sludge clearly decreased throughout the 38 days experiment (ESI Fig. S2†). The concentration of diFESOS in both control experiments did decrease up to day 2 before stabilizing, possibly due to partitioning from the liquid phase of the experiment into the particulate matter, or onto interfaces, as diFESOS is an extremely effective surfactant.
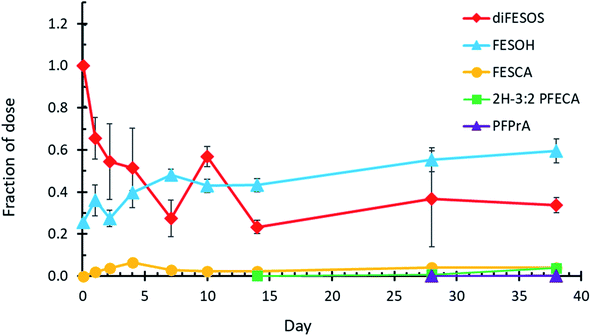 |
| Fig. 1 Biotransformation of diFESOS to FESOH and FESCA in 10% sludge over 38 days. Data points are normalized to the first measurement of diFESOS, correction for the 1 : 2 moles of polyfluoroalkyl chain present in diFESOS vs. its transformation products. Error bars represent standard deviation of the replicate experiments (n = 3). | |
FESOH concentrations in the live experiment increased from 0.26 ± 0.01 at the beginning of the experiment to 0.59 ± 0.06 of the dosed diFESOS on day 38. FESCA was first observed after one day at a molar fraction of 0.018 ± 0.005 compared to the diFESOS dose, and increased moderately throughout the experiment, resulting in 0.039 ± 0.014 on day 38. The sulfonate 2H-3:2 PFESA was not observed, nor was a larger metabolite resulting from the hydrolysis of only one FESOH equivalent (referred to as TP 524 in the ESI†). Two smaller degradation products, 2H-3:2 PFECA and PFPrA were observed in small concentrations beginning on day 14. Both control experiments formed small amounts of the lower molecular weight metabolites 2H-3:2 PFECA and PFPrA after 28 days, suggesting they may form in small amounts abiotically or the systems were no longer completely sterile (ESI Fig. S4 and S6†). These small metabolites were further investigated in the experiments where FESCA was dosed, as it is further down the biotransformation pathway and easier to elucidate their formation. The clearest difference between the live experiments versus the two controls are the big decrease in diFESOS, and large increase in FESOH formation, which is the first step of the biotransformation pathway (Fig. 1, ESI, S4 and S6†).
When the molar equivalents of diFESOS, FESOH, FESCA, 2H-3:2 PFECA and PFPrA (i.e. 1
:
2 moles diFESOS: metabolites) are summed at each time point, the mass balance of this live sludge experiment is adequate throughout the 38 days, culminating in 0.97 of the dosed diFESOS on day 38, compared to 1.26 of the dosed diFESOS at the 2 hours time point due to the FESOH impurity (see ESI Fig. S3†). Accounting for the FESOH impurity, this represents a 77% overall mass balance on day 38 of the experiment. The mass balance from the two control experiments, either autoclaved sludge or media had a similar mass balance to the live experiment over the 38 days time frame (see ESI Fig. S5 and S7†).
Three bottles were prepared by spiking diFESOS directly into raw, undiluted mixed liquor (ESI Fig. S8†). diFESOS was rapidly transformed, with nearly 80% loss after 2 days. The major difference compared to the experiment under sulfur limited conditions and with less sludge (thus lower microbial activity) is the large formation of the carboxylic acid FESCA and no buildup of the alcohol FESOH. After 38 days the fraction of FESCA formed was 0.79 ± 0.11 of the dosed diFESOS compared to 0.039 ± 0.014 after 38 days in the diluted, sulfur-limited bottles. The lower molecular weight metabolites 2H-3:2 PFECA and PFPrA also formed early compared to the other experiments. These experiments demonstrate that the same chemistry that occurs in sulfur-limited conditions appears to occur under unaltered wastewater treatment plant conditions.
Degradation of FESOH to FESCA
To confirm that FESOH is the direct precursor to FESCA, FESOH was incubated with activated sludge in a sulfate-free defined medium. After 38 days, the amount of FESOH decreased to 0.52 ± 0.10 of the FESOH concentration at the 2 hours timepoint (Fig. 2). The concentration of FESCA increased up to day 4, as the concentration of FESOH decreased, indicating that the carboxylic acid FESCA is formed from the alcohol FESOH precursor. After day 4, the concentration of FESCA decreased and was maintained at about 0.1 of the starting FESOH concentration throughout the entire experiment. On day 28, small amounts of 2H-3:2 PFECA and PFPrA were observed as well, similar to what was observed in the diFESOS experiments. The mass balance for the live experiments was 67% on day 38 (ESI Fig. S9†), which was similar to the control experiments with FESOH in autoclaved sludge (ESI Fig. S11†), likely due to the volatility of FESOH, and potential sorption to the experimental vessels.
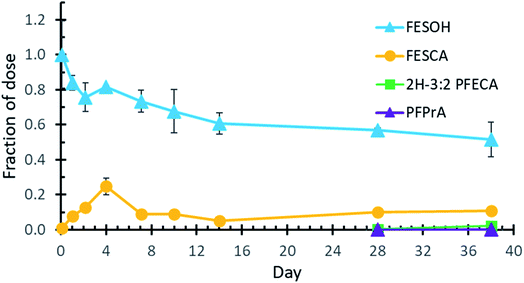 |
| Fig. 2 Biotransformation of FESOH to FESCA in 10% sludge over 38 days. Data points are normalized to the first measurement of FESOH. Error bars represent standard deviation of the replicate experiments (n = 3). | |
Degradation of FESCA to lower fluorinated molecules
The 84 days incubation of FESCA with activated sludge with a flow-through air setup showed that FESCA degrades slowly and consistently in a constantly oxygenated system. This experiment elucidates the later stages of diFESOS transformation, including over a longer time period. This system was validated using 6:2 FTUCA as a positive control (ESI Fig. S14†). It has previously been used as a positive control in separate vessels,23 but in this work, we dosed 6:2 FTUCA into the same vessels as FESCA similar to the use of 13C-monoisononylphthalate into sediment microcosms characterizing the biotransformation of other PFAS.27 Metabolites of FTUCAs have been characterized in a soil–water microcosm28 and as intermediates in the aerobic biotransformation of FTOHs using wastewater treatment plant sludge.24,29,30 The 6:2 FTUCA dosed into each of the experiments was almost completely gone after 21 days (see ESI, Fig. S14†), while FESCA had decreased about 25% within the same 21 days. On day 27, an additional spike of 6:2 FTUCA in ethanol was added to all live experiments to continue monitoring the viability of the setup and ethanol only was added to the autoclaved controls, providing a small additional carbon source to the system in addition to the original defined mineral media. The consistent concentration of 6:2 FTUCA in the autoclaved controls confirmed that they were sterile.
At the beginning of the experiment, the stock solution of FESCA contained small amounts of 2H-3:2 PFECA and PFPrA which is evident in the control experiments. The concentrations of these two molecules did not increase in the control experiments throughout the experimental time frame, remaining constant at 16.7 ± 1.3 nM PFPrA and 41.9 ± 3.4 nM 2H-3:2 PFECA. To best display the changes in chemical concentration throughout the experiment, the decrease of FESCA and the increases of 2H-3:2 PFECA and PFPrA are plotted against the autoclaved controls in Fig. 3 (Fig. S12† in the ESI for plots all three chemicals in the live experiments). FESCA decreased steadily over time with 641 ± 178 nM (47 ± 11% of the dosed FESCA) remaining after 84 days, while the concentrations in the autoclaved experiments were steady throughout the experiment, with a slight decrease by the end. 2H-3:2 PFECA was observed as a biotransformation product of FESCA in the live experiments, with a concentration of 224 ± 50 nM after 84 days, compared to the starting concentration of 45.7 ± 5.6 nM 2H-3:2 PFECA present as an impurity in the FESCA dose. Concentrations of 2H-3:2 PFECA are semi-quantitative, using perfluoropentanoic acid (PFPeA) as a surrogate standard, so the absolute concentration may be somewhat higher or lower than reported here. Small amounts of PFPrA were formed in the live incubations of FESCA, suggesting it is generated slowly from the biotransformation pathway of FESCA. After 84 days, the concentration of PFPrA was 23.9 ± 1.6 nM, compared to 17.6 ± 1.5 nM at the start of the experiment. PFPrA is a very minor product in this system, but corroborates what was observed in Sprague-Dawley rats18 and with atmospheric oxidants.17 The mass balance of FESCA, 2H-3:2 PFECA and PFPrA in the live experiments generally ranged from 75–100%, but decreased to 60% on day 84 (ESI Fig. S13†). This is lower than the mass balance of the autoclaved controls which was 80% after 84 days, suggesting there are other transformation products in addition to 2H-3:2 PFECA and PFPrA, or that the approximate quantification of 2H-3:2 PFECA using a surrogate standard was underestimating the actual concentration. Most of the lost FESCA was accounted for by the formation of 2H-3:2 PFECA.
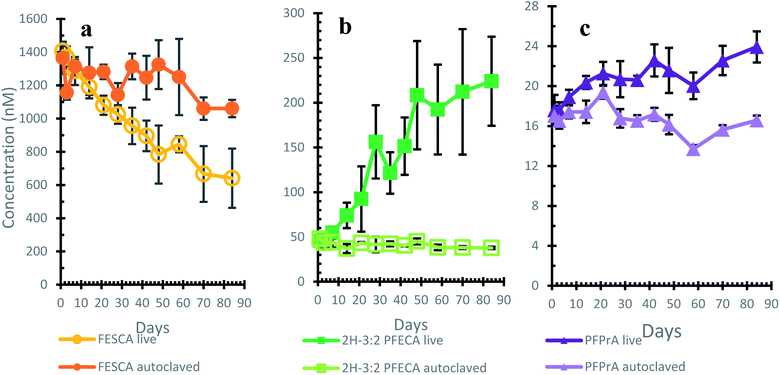 |
| Fig. 3 Biotransformation of FESCA using live sludge compared to autoclaved sludge: (a) decrease in FESCA concentration; (b) increase in 2H-3:2 PFECA concentration; (c) slight increase in PFPrA concentration. Note different scales of the y-axes (n = 3, except n = 2 for days 58, 70, 84). | |
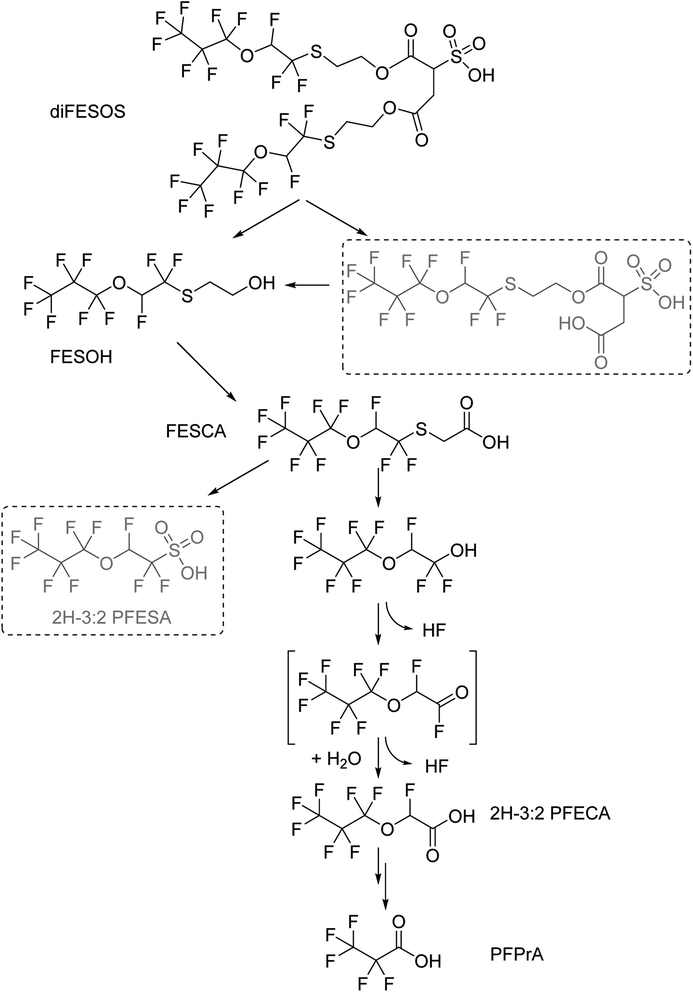 |
| Fig. 4 Proposed degradation scheme of diFESOS and its metabolites in wastewater treatment plant inoculum. Grey dashed boxes = not detected in this work. Double arrows represent multiple reaction steps. | |
Biotransformation pathway: defluorination on carbon alpha to sulfur atom
diFESOS is transformed to FESOH, then FESCA, and then further reaction appears to be the rate-limiting step in the overall degradation of diFESOS. FESCA contains multiple reaction sites: a CH2 group, a sulfur atom, and a H on an OCHF that can be oxidized (Fig. 4). When discussing this biotransformation, it is important to note that the majority of these experiments were performed under sulfur-limited conditions, and may progress at different rates under other environmental conditions, or perhaps even produce different products.
Other sulfur-containing PFAS that undergo aerobic microbial transformations can be generally categorized into three groups: (1) PFAS containing a perfluoroalkyl sulfonamide moiety, RFCF2–SO2–NR2; (2) fluorotelomer sulfonamides, RFCH2CH2–SO2–NR2; (3) fluorotelomer thioethers, RFCH2CH2–S–CH2R, where RF is a perfluoroalkyl chain. The perfluoroalkyl sulfonamide PFAS generally form a sulfonate with the corresponding perfluoroalkyl chain length, for example, PFOS, with no further degradation.27,31–34 The fluorotelomer sulfonamides 6:2 fluorotelomer alkyl betaine (FTAB) and fluorotelomer alkyl amine (FTAA) were oxidized to 6:2 fluorotelomer sulfonic acid (FTSA) in activated sludge, and then desulfonation was observed with the formation of 6:2 FTOH.23 Similarly, the formation of 6:2 FTOH was observed from the incubation of 6:2 FTSA with aerobic sediment.35 The pure culture Gordonia sp. strain NB4-1Y also degraded 6:2 FTAB and 6:2 FTSA to 6:2 FTOH under sulfur-limited conditions.36 For the fluorotelomer thioethers, only one class of PFAS has been studied under aerobic biotransformation conditions: x:2 fluorotelomer mercaptoalkylamido sulfonates (x:2 FTSAS), also known as x:2 fluorotelomer thioamido sulfonates (x:2 FtTAoS).37,38 Both papers reported direct oxidation of the sulfur atom forming the corresponding sulfoxide, but only Harding-Marjanovic et al. observed the corresponding sulfone.38 Weiner et al. used GC-MS to identify both 6:2 FTOH and 6:2 FTSH biotransformation products, which formed from either the S-dealkylation or S-oxygenation, respectively.37 FESCA differs from x:2 FTSAS as it has a CF2 group alpha to the thioether, with RCF2–S–CH2R. Here, we do not observe S-oxygenation, as the proposed sulfoxide FESCA + O (m/z 372.9598) and sulfone FESCA + O2 (m/z 388.9547) were not observed in the high-resolution mass spectrum, even when using a generous 10 ppm window (Table S4†). However, we do observe evidence of S-dealkylation of the polyfluoroalkyl chain via the formation of C3F7OCHFCF2OH, and further metabolites. To probe this hypothesis, samples from day 42 of the FESCA experiment were analyzed using our suspect list via LC-Orbitrap. This alcohol was detected in samples from the live experiment (observed m/z 282.9832, error 3.43 ppm) and was not observed in the controls. We were unable to quantify this metabolite as we did not have an available standard. The analogous S-dealkylation of 6:2 FTSAS and FESCA is drawn in the ESI Fig. S15.† In work by Key et al., desulfonation only occurred in compounds containing hydrogen atoms on carbons alpha to sulfonates,39 and to our knowledge this is the first report of desulfonation with fluorine atoms on a carbon alpha to sulfur. We also believe this is the first report of defluorination adjacent to sulfur atoms. Alcohols with adjacent CF2 groups readily eliminate HF to form an acyl fluoride,4 in this case is C3F7OCHFC(O)F, which will subsequently hydrolyze to form C3F7OCHFC(O)OH, 2H-3:2 PFECA, a major metabolite observed in this work. During these abiotic steps, two fluorine atoms are liberated. This shows that a molecule containing a –CF2SCH2– has potential for the cleavage of two C–F bonds.
The H-atom in the CHF group adjacent to the ether in diFESOS, FESOH, FESCA and 2H-3:2 PFECA is deactivated by the fluorine atoms consistent with very slow formation of PFPrA. Another PFAS with an –OCHF– moiety is ADONA, 4,8-dioxa-3H-perfluorononanoate (F3CO(CF2)3OCHFCF2C(O)OH). It was not readily biodegradable under the OECD 301B test,40 but no longer-term biotransformation experiments have been performed of which we are aware. However, under chemical oxidative conditions, ADONA is rapidly oxidized, while perfluoroalkyl ether acids such as GenX do not react.15 The biotransformation from 2H-3:2 PFECA to PFPrA liberates an additional three fluorine atoms. The entire transformation of diFESOS begins with 20 fluorine atoms in the molecule, is halved to 10 fluorine atoms in FESOH and FESCA, then two defluorination steps occur producing 2H-3:2 PFECA with 8 fluorine atoms, and finally, 3 additional fluorine atoms are liberated, resulting in PFPrA as a terminal product with 5 fluorine atoms. We note that PFPrA is formed slowly in our experiments and is a very minor product. Both 2H-3:2 PFECA and PFPrA likely have low bioaccumulation potential, based on trends summarized by Ng and Hungerbühler, which show that shorter perfluoroalkyl acids have lower bioaccumulation.41 2H-3:2 PFECA is not a perfluoroalkyl acid as it contains an ether and a hydrogen atom on one carbon, but it can be compared to PFPeA or the 4-carbon perfluoroalkyl acid PFBA. However, these two metabolites may accumulate in plants,42 and ultimately are quite persistent. Shorter chain per- and polyfluorinated carboxylic acids have increased water solubility and mobility, which may lead to more widespread contamination.14 Lastly, the biotransformation in this system differs from what was observed with the atmospheric oxidation of FESOH,17 and rat metabolism of diFESOS,18 as the sulfonate 2H-3:2 PFESA was not observed in this work. Understanding transformation products under different environmental conditions is necessary to assess overall fate.
Conflicts of interest
There are no conflicts of interest to declare.
Acknowledgements
Jessica D'eon, Andrew Folkerson, Jeremy Gauthier, Nadia Morson, Line Lomheim and Elizabeth Edwards (University of Toronto) are acknowledged for technical support and useful discussion. Hui Peng is thanked for the use of his Orbitrap (University of Toronto). We thank the staff at Ashbridge's Bay Wastewater Treatment Plant (Toronto, ON) for assistance acquiring the mixed liquor. Funding for this work was contributed by Merck kGaA (Darmstadt, Germany), and we thank Reiner Friedrich for useful discussion. S. J. acknowledges doctoral funding from an NSERC CGS D.
References
-
E. Kissa
Fluorinated Surfactants and Repellents, Mercel Dekker, New York, NY, 2nd edn, 2001 Search PubMed.
-
Stockholm Convention, SC 4/17 Listing of perfluorooctanesulfonic acid, its salts and perfluorooctane sulfonyl fluoride, UNEP-POPS-COP.4-SC-4/17, Stockholm Convention, Chatelaine, Switzerland, 2009 Search PubMed.
-
Stockholm Convention, SC 9/12 Listing of perfluorooctanoic acid (PFOA), its salts and PFOA-related compounds, UNEP-POPS-SC-9/12, Stockholm Convention, Chatelaine, Switzerland, 2019 Search PubMed.
-
C. J. Young and S. A. Mabury, Atmospheric Perfluorinated Acid Precursors: Chemistry, Occurence, and Impacts, in Reviews of Environmental Contamination and Toxicology, ed. P. de Voogt, Springer, New York, 2010, vol. 208, Perfluorinated alkyl substances, pp. 1–109 Search PubMed.
- J. C. D’eon and S. A. Mabury, Is indirect exposure a significant contributor to the burden of perfluorinated acids observed in humans?, Environ. Sci. Technol., 2011, 45(19), 7974–7984 CrossRef PubMed.
- C. M. Butt, D. C. G. Muir and S. A. Mabury, Biotransformation pathways of fluorotelomer-based polyfluoroalkyl substances: a review, Environ. Toxicol. Chem., 2014, 33(2), 243–267 CrossRef CAS PubMed.
-
U.S. EPA, Phase-out Plan for POSF-based Products, U.S. EPA Public Docket, OPPT-2002-0043, 3M Specialty Materials Markets Group, St. Paul, MN, 2000 Search PubMed.
-
U.S. EPA, PFOA Stewardship Program, U.S. EPA Public Docket OPPT-2006-0621 Search PubMed.
-
Environment Canada, Environmental Performance Agreement Respecting Perfluorinated Carboxylic Acids (PFCAs) and Their Precursors in Perfluorochemical Products Sold in Canada, 2010 Search PubMed.
- Z. Wang, I. T. Cousins, M. Scheringer and K. Hungerbühler, Fluorinated alternatives to long-chain perfluoroalkyl carboxylic acids (PFCAs), perfluoroalkane sulfonic acids (PFSAs) and their potential precursors, Environ. Int., 2013, 60, 242–248 CrossRef CAS PubMed.
- J. R. Parsons, M. Sáez, J. Dolfing and P. de Voogt, Biodegradation of perfluorinated compounds, Rev. Environ. Contam. Toxicol., 2008, 196, 53–71 CrossRef CAS PubMed.
- J. W. Martin, S. A. Mabury, K. R. Solomon and D. C. G. Muir, Dietary accumulation of perfluorinated acids in juvenile rainbow trout (Oncorhynchus mykiss), Environ. Toxicol. Chem., 2003, 22(1), 189–195 CrossRef CAS PubMed.
- K. Ohmori, N. Kudo, K. Katayama and Y. Kawashima, Comparison of the toxicokinetics between perfluorocarboxylic acids with different carbon chain length, Toxicology, 2003, 184(2–3), 135–140 CrossRef CAS PubMed.
- Z. Wang, I. T. Cousins, M. Scheringer and K. Hungerbuehler, Hazard assessment of fluorinated alternatives to long-chain perfluoroalkyl acids (PFAAs) and their precursors: Status quo, ongoing challenges and possible solutions, Environ. Int., 2015, 75, 172–179 CrossRef CAS PubMed.
- C. Zhang, Z. R. Hopkins, J. McCord, M. J. Strynar and D. R. U. Knappe, Fate of Per- And Polyfluoroalkyl Ether Acids in the Total Oxidizable Precursor Assay and Implications for the Analysis of Impacted Water, Environ. Sci. Technol. Lett., 2019, 6(11), 662–668 CrossRef CAS PubMed.
-
Tivida Fluorosurfactants
https://www.emdgroup.com/en/brands/pm/tivida.html
Search PubMed.
- S. Joudan, J. J. Orlando, G. S. Tyndall, T. Furlani, C. J. Young and S. A. Mabury, Atmospheric fate of a new polyfluoroalkyl building block, C3F7OCHFCF2SCH2CH2OH, Environ. Sci. Technol., 2021 DOI:10.1021/acs.est.0c07584.
- A. P. Folkerson, S. Joudan, S. A. Mabury and J. C. D’eon,
In Vivo Transformation of a Novel Polyfluoroether Surfactant, Environ. Toxicol. Chem., 2021, 40, 3328–3336 CrossRef CAS PubMed.
- A. Remde and R. Debus, Biodegradability of fluorinated surfactants under aerobic and anaerobic conditions, Chemosphere, 1996, 32(8), 1563–1574 CrossRef CAS PubMed.
- R. J. W. Meesters and H. F. Schröder, Perfluorooctane sulfonate - A quite mobile anionic anthropogenic surfactant, ubiquitously found in the environment, Water Sci. Technol., 2004, 50(5), 235–242 CrossRef CAS PubMed.
- E. Sinclair and K. Kannan, Mass loading and fate of perfluoroalkyl surfactants in wastewater treatment plants, Environ. Sci. Technol., 2006, 40(5), 1408–1414 CrossRef CAS PubMed.
- J. D. Van Hamme, P. M. Fedorak, J. M. Foght, M. R. Gray and H. D. Dettman, Use of a Novel Fluorinated Organosulfur Compound to Isolate Bacteria Capable of Carbon–Sulfur Bond Cleavage, Appl. Environ. Microbiol., 2004, 70(3), 1487–1493 CrossRef CAS PubMed.
- L. A. D'Agostino and S. A. Mabury, Aerobic biodegradation of 2 fluorotelomer sulfonamide-based aqueous film-forming foam components produces perfluoroalkyl carboxylates, Environ. Toxicol. Chem., 2017, 36(8), 2012–2021 CrossRef PubMed.
- M. J. A. Dinglasan, Y. Ye, E. A. Edwards and S. A. Mabury, Fluorotelomer Alcohol Biodegradation Yields Poly- and Perfluorinated Acids, Environ. Sci. Technol., 2004, 38(10), 2857–2864 CrossRef CAS PubMed.
- J. C. D'eon and S. A. Mabury, Production of perfluorinated carboxylic acids (PFCAs) from the biotransformation of polyfluoroalkyl phosphate surfactants (PAPS): Exploring routes of human contamination, Environ. Sci. Technol., 2007, 41(13), 4799–4805 CrossRef PubMed.
- H. Lee, J. C. D'eon and S. A. Mabury, Biodegradation of polyfluoroalkyl phosphates as a source of perfluorinated acids to the environment, Environ. Sci. Technol., 2010, 44(9), 3305–3310 CrossRef CAS PubMed.
- J. P. Benskin, M. G. Ikonomou, F. A. P. C. Gobas, T. H. Begley, M. B. Woudneh and J. R. Cosgrove, Biodegradation of N-ethyl perfluorooctane sulfonamido ethanol (EtFOSE) and EtFOSE-based phosphate diester (SAmPAP diester) in marine sediments, Environ. Sci. Technol., 2013, 47(3), 1381–1389 CrossRef CAS PubMed.
- A. L. Myers and S. A. Mabury, Fate of fluorotelomer acids in a soil–water microcosm, Environ. Toxicol. Chem., 2010, 29(8), 1689–1695 CAS.
- N. Wang, B. Szostek, P. W. Folsom, L. M. Sulecki, V. Capka, R. C. Buck, W. R. Berti and J. T. Gannon, Aerobic biotransformation of 14C-labeled 8-2 telomer B alcohol by activated sludge from a domestic sewage treatment plant, Environ. Sci. Technol., 2005, 39(2), 531–538 CrossRef CAS PubMed.
- N. Wang, B. Szostek, R. C. Buck, P. W. Folsom, L. M. Sulecki, V. Capka, W. R. Berti and J. T. Gannon, Fluorotelomer Alcohol Biodegradations – Direct Evidence that Perfluorinated Carbon Chains Breakdown, Environ. Sci. Technol. Lett., 2005, 39, 7516–7528 CrossRef CAS PubMed.
-
C. C. Lange, The Aerobic Biodegradation of N-EtFOSE Alcohol by the Microbial Activity Present in Municipal Wastewater Treatment Sludge, U.S. Environmental Protection Agency Docket AR-226, 2000 Search PubMed.
- K. R. Rhoads, E. M. L. Janssen, R. G. Luthy and C. S. Criddle, Aerobic biotransformation and fate of N-ethyl perfluorooctane sulfonamidoethanol (N-EtFOSE) in activated sludge, Environ. Sci. Technol., 2008, 42(8), 2873–2878 CrossRef CAS PubMed.
- S. Mejia-Avendaño and J. Liu, Production of PFOS from aerobic soil biotransformation of two perfluoroalkyl sulfonamide derivatives, Chemosphere, 2015, 119, 1084–1090 CrossRef.
- I. Zabaleta, E. Bizkarguenaga, D. B. O. Nunoo, L. Schultes, J. Leonel, A. Prieto, O. Zuloaga and J. P. Benskin, Biodegradation and Uptake of the Pesticide Sulfluramid in a Soil–Carrot Mesocosm, Environ. Sci. Technol., 2018, 52(5), 2603–2611 CrossRef CAS PubMed.
- N. Wang, J. Liu, R. C. Buck, S. H. Korzeniowski, B. W. Wolstenholme, P. W. Folsom and L. M. Sulecki, 6:2 Fluorotelomer sulfonate aerobic biotransformation in activated sludge of waste water treatment plants, Chemosphere, 2011, 82(6), 853–858 CrossRef CAS PubMed.
- D. M. J. Shaw, G. Munoz, E. M. Bottos, S. V. Duy, S. Sauvé, J. Liu and J. D. Van Hamme, Degradation and defluorination of 6:2 fluorotelomer sulfonamidoalkyl betaine and 6:2 fluorotelomer sulfonate by Gordonia sp. strain NB4-1Y under sulfur-limiting conditions, Sci. Total Environ., 2019, 647, 690–698 CrossRef CAS PubMed.
- B. Weiner, L. W. Y. Yeung, E. B. Marchington, L. A. D'Agostino and S. A. Mabury, Organic fluorine content in aqueous film forming foams (AFFFs) and biodegradation of the foam component 6:2 fluorotelomermercaptoalkylamido sulfonate (6:2 FTSAS), Environ. Chem., 2013, 10(6), 486–493 CrossRef CAS.
- K. C. Harding-Marjanovic, E. F. Houtz, S. Yi, J. A. Field, D. L. Sedlak and L. Alvarez-Cohen, Aerobic Biotransformation of Fluorotelomer Thioether Amido Sulfonate (Lodyne) in AFFF-Amended Microcosms, Environ. Sci. Technol., 2015, 49(13), 7666–7674 CrossRef CAS PubMed.
- B. D. Key, R. D. Howell and C. S. Criddle, Defluorination of Organofluorine Sulfur Compounds by Pseudomonas Sp. Strain D2, Environ. Sci. Technol., 1998, 32, 2283–2287 CrossRef CAS.
- S. C. Gordon, Toxicological evaluation of ammonium 4,8-dioxa-3H-perfluorononanoate, a new emulsifier to replace ammonium perfluorooctanoate in fluoropolymer manufacturing, Regul. Toxicol. Pharmacol., 2011, 59(1), 64–80 CrossRef CAS PubMed.
- C. A. Ng and K. Hungerbuhler, Bioaccumulation of Perfluorinated Alkyl Acids : Observations and Models, Environ. Sci. Technol., 2014, 48, 4637–4648 CrossRef CAS PubMed.
- S. Felizeter, M. S. McLachlan and P. De Voogt, Uptake of perfluorinated alkyl acids by hydroponically grown lettuce (Lactuca sativa), Environ. Sci. Technol., 2012, 46(21), 11735–11743 CrossRef CAS PubMed.
Footnote |
† Electronic supplementary information (ESI) available: Additional information on chemicals used, experimental details, mass spectrometry details and more results (PDF). See DOI: 10.1039/d1em00358e |
|
This journal is © The Royal Society of Chemistry 2022 |
Click here to see how this site uses Cookies. View our privacy policy here.