Outdoor and indoor natural background gamma radiation across Kerala, India
Received
4th May 2021
, Accepted 15th September 2021
First published on 16th December 2021
Abstract
The major share of the total radiation exposure in human beings is from natural sources and hence its measurement is vital to the fundamental understanding of radiation exposure. Natural background gamma radiation of 72 outdoor sites and 32 indoor sites covering all 14 districts of Kerala, a southern state of India, was monitored over a period of two years. Thermo Luminescent Dosimeters (TLDs) and a radiation survey meter were used for the detection. Kollam district (2.32 mGy per year) showed the maximum and Malappuram district (0.64 mGy per year) showed the minimum outdoor values. In the case of indoor radiation, a house in Palode, Thiruvanathapuram district and a house in Thumpamon in Pathanamthitta district showed maximum radiation dose levels (1.69 mGy per year and 1.64 mGy per year, respectively). Indoor environments showed higher radiation dose than the outdoor environment which may be attributed to the contribution from construction materials. The highest Annual Effective Dose (AED) was observed in Chavara area as it is located in a High Background Radiation Area (HBRA). The highest radiation values were observed in concrete houses. Since most of the studied houses were concrete except three or four, no significant difference was observed in the radiation level. In the present study, the maximum indoor to outdoor ratio (I/O) was obtained from a house in Pathanamthitta district. In certain sites it was found that the indoor dosage levels were 1.5 times higher than the outdoor levels, as almost all the sites had outdoor gamma dosage above the world average. These results have a high importance as the values are not common and very specific to this region.
Environmental significance
The southern state of Kerala (one of the southern provinces of India, on the Arabian Coast) is a region where the background radiation is among the highest reported in the world. This region is under constant research on the possible health aspects. This work was completed as part of a national mission on the assessment of background radiation in India. In this context, the work reported in this manuscript was to understand the natural background radiation in the entire state. It is also all the more important to understand the situation of background radiation within houses (indoor radiation) as the state has the highest population density in India. This is the first report covering an entire state of India which is known to have the highest background outdoor radiation. The values of both indoor and outdoor radiation have been compared with the world average and with respect to some other countries. To the best of our knowledge, this is the first report on comprehensive data on the indoor and outdoor radiation of an entire province anywhere in the world, having such a high population density. A clear cut understanding of the magnitude of background radiation is the first step towards understanding the environmental implications.
|
1. Introduction
Natural background radiation is produced from sources of terrestrial and extra-terrestrial origin. The measurement of the effects of radiation exposure from terrestrial and extra-terrestrial sources is vital to have sufficient information about the distribution of radionuclides and radiation levels existing in the environment.1 Taking into account the total radiation exposure, more than 80% of the dose has a natural origin.2 Cosmic radiation, terrestrial radiation, radon along with its decay products and radionuclides which enter into the body due to ingestion are considered to be the prime sources of natural background radiation as their dose rates are much higher when compared to the other sources.3 Various regions of the world are exposed to high dose rates of background radiation due to the uncharacteristic occurrence of thorium in monazite sands as well as the presence of uranium and its decay products in rocks, soil, and groundwater.4 We are exposed to significant amounts of radiation from radon and its decay products when compared to other sources. Uranium, thorium and potassium are the prime sources attributed to the natural radioactivity observed in soil and building materials. Activities such as mining and milling of heavy minerals and sands, their waste management plus the usage of detrimental construction materials are considered to have made the general public indoors exposed to dosage from 220Rn and 224Ra.5–10
Environmental gamma radiation levels can be measured using a variety of instruments like G. M. counters, high pressure ion chambers, portable and airborne gamma spectrometers and scintillation survey meters. However, these instruments give instantaneous readings at the time of measurement. In order to evaluate the integrated background radiation levels many types of passive detectors are being employed in environmental monitoring. The advantages of passive detectors for environmental monitoring are that they are small, cheap, and reusable, do not require in situ electronic power supply and can be used in a large dose range. Amongst them the most widely employed environmental passive systems are based on luminescence detectors such as thermoluminescence (TLD), photoluminescence (PLD) or optically stimulated luminescence detectors (OSLD). Luminescence phenomena are named after the type of radiation used to excite or to stimulate the light emission.
Kerala, which is situated in the southern part of India, is known for the localized high background radiation levels due to thorium containing monazite sand. A number of studies have been carried out in the past few decades in Kerala by many researchers all over the world with a view to assess the risks and effects of long-term exposure to natural radiation, particularly with respect to human inhabitants. Most of these studies were focused on the high background radiation areas of the state. But there are no data available in the literature on natural gamma radiation levels distinguishing various areas across the state. In addition, a state wide indoor radiation level study is also lacking which is equally important since the state has one of the highest background radiation areas in it. Therefore, comprehensive indoor as well as outdoor environmental gamma radiation monitoring was carried out in this study across the state of Kerala using TLDs. TLDs use a cumulative method which is more active than electronic measurement due to its time consuming measurement.
2. Materials and methods
2.1. Study area
Kerala is wedged between the Arabian Sea in the west and Western Ghats in the east with an area of approximately 38
863 km2 and the width varies between 35 and 120 km. The entire state of Kerala can be divided into three geographical regions, the eastern mountainous terrain, the central midlands (rolling hills), and the western coastal plains. The eastern mountainous highlands rise to an average height of 900 m, with a number of peaks well over 1800 m in height. It accounts for 48% of the total land area of Kerala. The Midlands, lying between the mountains and the lowlands, is made up of undulating hills and valleys. It is about 40% of the total land area. The western coastal plain of Kerala runs approximately 580 km in length covering 10% of the total state area. It is made up of numerous shallow lagoons known locally as kayals, river deltas, backwaters and shores of the Arabian Sea.
The entire state is divided into 14 districts. All the districts were covered in this study for the indoor as well as outdoor natural gamma radiation level measurements. In total, 72 outdoor and 32 indoor sites were monitored for two years continuously on a quarterly basis using TLDs (Fig. 1).
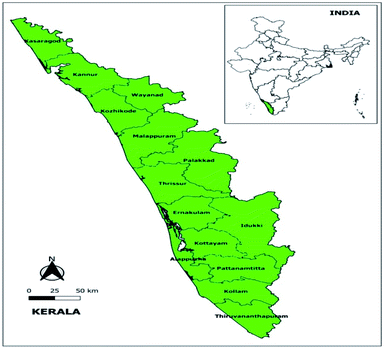 |
| Fig. 1 Study area – Kerala. | |
2.2. Description of the thermoluminescence dosimeter
Measurements of natural gamma radiation levels were carried out using TLDs based on CaSO4:Dy Teflon discs. TLD is a passive device for the measurement of gamma dose and in this the dose is acquired and stored for a long period of time until the system is stimulated by heat. The doping concentration of Dy in TLD discs was optimized to make it specifically suitable for environmental dosimetric purposes. The measured dose rates include both terrestrial and cosmic ray components. Prior to deployment; these TLDs were annealed at 250 °C for a period of 3 h in an oven with air circulation. The TLD discs were made from a homogeneous mixture of CaSO4:Dy powder and Teflon resin taken in a weight ratio of 1
:
3. Discs of this mixture weighing 280 mg each were first cold pressed and then subjected to a 400 °C h−1 heat treatment so that they become strong as well as flexible. These discs were fixed in machine identifiable aluminium cards. The cards were annealed at 250 °C for 3 hours and subjected to various degrees of exposure to a 137Cs gamma radiation source.
2.3. Measurement of background radiation
Two types of TLDs were used for the study, one type for outdoor survey (areawise, one TLD per 625 km2) which was deployed above 1 to 2 m from the ground level and another for indoor survey (one TLD per 1
000
000 population). 72 Outdoor sites and 32 indoor sites were monitored for the study. TLD batches were deployed for a 3 month period in the open environment at different locations and the work was completed in seven batches. TLDs were sent to BARC for analysis. A handheld survey meter was used for on time monitoring of background radiation.
2.4. Dose evaluation
The proper dose evaluation for a passive monitoring system involves many parameters. Some of them can be considered as permanent properties because they are independent of the changing aspects during the monitoring period. These parameters are normally determined during type testing and they provide the performances and features of the system. The criteria and procedures for environmental radiation monitoring systems using passive dosimeters are described in some international standards [IEC91b], including tests for linearity, batch homogeneity, reproducibility, detection threshold, photon energy response, angular response and stability.
Passive detectors need to be prepared, packed, and transported to the field site and, after the field exposure, returned to the laboratory for the readout. Therefore, the detectors receive not only the dose in the field, but also undesired doses during these procedures. The transit dose Dt is a measure of this additional contribution and it should be subtracted from the field readouts
D = [M × N × Fad × F(E,Q)] − Dt − SD |
D = field dose,
M − detector readout, non-radiation induced component subtracted,
N = calibration factor, ICF = individual correction factor (ICF = 1 for batch calibration), Fad = fading correction factor,
F(
E,
Q) = energy and angular response correction factors, Dt = transit dose, SD = self-dose correction.
3. Results and discussion
Primordial radionuclides are omnipresent in the environment in trace concentrations in all soils; of these, gamma emitting radionuclides such as 238Th, 232U series and 40K are the major contributors to the irradiation of human body from external sources. Among different rock types, igneous rocks like granite were found to have higher radiation dosage levels whereas sedimentary rocks showed lower levels with exceptions wherein some shale and phosphate rocks consisted of higher amount of radionuclides.11 The presence of springs and quarries in certain regions can sometimes increase the background radiation to significant levels.12
Natural ionizing radiation contributes to around 80% of the collective radiation dose in the world population.13 Humans may receive radiation dose either through external or internal exposure. Cosmic rays and terrestrial gamma rays are the prime contributors to external exposure whereas radon progeny and 40K are the major contributors to internal exposure.14 Geomagnetic field, altitude and solar cycle influence the external dosage received from cosmic radiation while the concentrations of radioactive potassium and radioactive components of natural uranium and thorium radioactive decay chains influence the variation in the external dose received from terrestrial radiation.11 Locations situated in high altitude regions have 10–12% more background radiation than low lands.15 Additional sources like urbanisation, industrial waste, weapon testing and radioactive waste also contribute to the background radiation level.16,17
3.1. Annual outdoor gamma dose rate variation in selected sites across different districts of Kerala
Estimation of natural terrestrial gamma dose rate is important as it is the prime external source of irradiation the human body is exposed to. It also helps in the identification of potential health risks and the data generated could be used as a tool for environmental monitoring.18 The annual outdoor gamma radiation levels in 72 sites across different districts of Kerala were monitored. Table 1 depicts the range and average of the gamma dose rate measured in various districts across the state. The reported national average of outdoor natural radiation in India is 0.734 mGy per year.16,19 Out of the 14 districts monitored, only Wayanad (0.66 mGy per year), Malappuram (0.64 mGy per year) and Thrissur (0.71 mGy per year) districts fell within the national average. Dose levels at all the other sites surpassed the world average value of 0.48 mGy per year.20 Among the 14 districts, Malappuram (0.64 mGy per year), Wayanad (0.66 mGy per year), Palakkad (0.72 mGy per year) and Thrissur (0.71 mGy per year) recorded lower annual mean dose values. Very high dose values were observed in Kollam (2.38 mGy per year) which is about 5 times higher than that of the world average. This (Kollam) region is situated in the high background area of the state.21,22 Wayanad and Idukki are the two districts that fall in the high altitude region. Both have an average elevation of 903 MSL (Wayanad)23 and 1200 MSL (Idukki),24 respectively. Neutron contribution from cosmic rays fluctuates from 8% near the sea level to about 35% 4000 meters above sea level (m.a.s.l). This may lead to regions located at high elevations having more radiation dose values than regions at low elevation.25 The annual average dose values recorded for Wayanad and Idukki were 0.66 mGy per year and 1.06 mGy per year, respectively. So, there was a significant difference in outdoor gamma dose value measured for these (Wayanad and Idukki) districts. Furthermore, we could not observe significant changes in the gamma dose levels with altitude difference within the state. Therefore, it may be concluded that the radiation dose from geographical features (soil and rock characteristics) is more predominant in determining the radiation dose in the study area than the effect of cosmic ray contributions.
Table 1 Natural background outdoor gamma radiation levels across different districts of Kerala
District |
Range (mGy per year) |
Average (mGy per year) |
Kollam |
0.97–4.27 |
2.32 |
Kottayam |
0.67–1.17 |
0.99 |
Ernakulam |
0.81–1.05 |
0.95 |
Idukki |
0.99–1.17 |
1.06 |
Thiruvananthapuram |
0.85–1.53 |
1.20 |
Thrissur |
0.53–0.85 |
0.71 |
Pathanamthitta |
0.77–1.03 |
0.95 |
Palakkad |
0.53–0.83 |
0.72 |
Alapuzha |
0.56–1.10 |
0.83 |
Kozhikode |
0.65–1.11 |
0.77 |
Malappuram |
0.54–0.76 |
0.64 |
Wayanad |
0.62–0.73 |
0.66 |
Kannur |
0.71–1.19 |
0.89 |
Kasaragod |
0.60–1.12 |
0.79 |
3.2. Indoor radiation levels: a comparison with the outdoor data
Presence of radionuclides in the walls, floors and ceilings of houses could enhance the natural radiation background exposure as they contain 226Ra, 232Th, their associated decay products and 40K.26,27 A study carried out in Iran to analyse five prime construction materials, such as cement, gypsum, cement blocks, brick and gravel, found that the highest values for mean concentrations of 226Ra and 232Th were obtained for cement samples and the lowest values for gypsum. In the case of 40K, the highest and lowest values of mean concentrations were obtained from bricks and gypsum, respectively.12,28 A total of 32 sites were monitored, almost covering the entire districts in the state of Kerala to obtain the data for both indoor and outdoor natural background gamma radiation levels. The outdoor data are part of the total 72 sites mentioned in the previous section. The results obtained from this study are presented in Table 2. The indoor dosage values for this study ranged between 0.68 and 1.69 mGy per year with an average value of 1.05 mGy per year. The variation in factors like altitudes (mid, low and highlands), soil and rock characteristics, meteorological conditions and the ventilation rates among different sampling locations must have influenced the differences observed in the indoor gamma dose.29 Most of the houses monitored recorded a high indoor gamma dose when compared to outdoor gamma dose. The houses monitored were mainly constructed using cement and bricks/cement and cut stones (laterite). The roof characteristics could be divided into three types such as concrete, tiled and mixed roofs (concrete and tile), respectively. The basements of the houses were generally constructed using stones (e.g., Charnackite). The houses largely had cemented floors. Few houses had different floor types such as marble, granite, mosaic or vitrified tiles. Houses with floors of mixed nature, i.e., a combination of both cement and other materials (granite, marble, mosaic and tiles), were also present. In the houses that are located in rural areas, the residents prefer to open windows on most of the days while in houses that are in close proximity to roads having heavy traffic, especially the ones that are located close to the cities, often experience considerable amount of dust. Therefore, residents opt to close the windows; moreover, they also seal the holes and gaps kept in place for ventilation (generally found in the upper wall of the houses just below the roof to prevent dust from entering their houses). There has been a considerable change in the constructional pattern of houses in Kerala especially in terms of roofing characteristics in the last 30 to 40 years. A shift in the usage of reinforced concrete from the conventional tiled roofing is observed. Some houses that were monitored have both concrete and tiled roofing. This type of mixed roofing was observed in relatively old houses that had undergone renovation. The synergistic effect from the presence of the radionuclides within the building materials and insufficient ventilation must have elevated the indoor gamma dose levels29 in the houses with respect to outdoor dose levels in the study area. The houses located at Mayanoor in Thrissur District were made of cement and cut stones with mixed roofing where tiled roofing was more prevalent compared to concrete. This dwelling (Mayanoor) recorded the lowest indoor gamma dose value (0.68 mGy per year) in our study. The lower outdoor gamma radiation levels in the Mayanoor area and the adequate ventilation provided by the dominance of tiled roofing must have contributed to the lower indoor gamma dose value. Houses at Palod (1.69 mGy per year), Thonackal (1.62 mGy per year), Thumpamon (1.64 mGy per year) and Chavara (1.58 mGy per year) recorded relatively higher indoor gamma dose values compared to the rest of the houses monitored. Higher indoor values were observed for houses made of cement and bricks with concrete roofing. Bricks tend to have high 40K content making them an efficient contributor to indoor radiation (Mehdizadeh et al.,28 2011; Shahbazi-Gahrouei et al.,12 2013). Insufficient ventilation prevailed in these (Palod, Thonackal, Thumpamon and Chavara) houses. Construction design hindered the free flow of air into and out of the houses to an extent. Therefore, factors such as ventilation, building materials and outdoor gamma radiation levels existing in these areas may have contributed to the observed increased indoor gamma dose levels.
Table 2 Indoor gamma radiation levels across different districts of Kerala
District |
Location |
Outdoor (O) (mGy per year) |
Indoor (I) (mGy per year) |
Indoor–outdoor ratio (I/O) |
Kollam |
Chavara |
2.67 |
1.58 |
0.59 |
Kottayam |
Athirampuzha |
0.94 |
1.10 |
1.17 |
Vakathanam |
1.05 |
1.12 |
1.06 |
Ernakulam |
Maradu |
0.92 |
1.02 |
1.10 |
Kuthatukulam |
0.80 |
0.88 |
1.09 |
Muvattupuzha |
0.88 |
0.90 |
1.02 |
Paravur |
1.04 |
1.00 |
0.96 |
Eloor |
0.95 |
0.79 |
0.83 |
Idukki |
Kumali |
1.00 |
1.11 |
1.10 |
Thiruvananthapuram |
Palod |
1.40 |
1.69 |
1.20 |
Neyyatinkara |
1.29 |
1.32 |
1.02 |
Thonakkal |
1.52 |
1.62 |
1.06 |
Kallar |
0.84 |
1.18 |
1.39 |
Alappuzha |
Mavelikara |
0.99 |
1.14 |
1.15 |
Thrissur |
Mayanoor |
0.52 |
0.68 |
1.30 |
Kaipumangalam |
0.84 |
1.15 |
1.36 |
Vetilapara |
0.75 |
1.26 |
1.66 |
Pathanamthitta |
Thumpamon |
0.76 |
1.64 |
2.13 |
Konni |
1.03 |
1.03 |
1.00 |
Palakkad |
Vadakancheri |
0.79 |
1.08 |
1.36 |
Nelliyambathi |
0.82 |
1.31 |
1.58 |
Kozhikode |
Perambra |
0.83 |
0.69 |
0.83 |
Koyilandi |
1.10 |
0.96 |
0.87 |
Malappuram |
Parapanagadi |
0.70 |
0.82 |
1.16 |
Manjeri, Payanadu |
0.66 |
0.84 |
1.26 |
Wayanad |
Mananthavadi |
0.72 |
0.75 |
1.03 |
Sulthanbatheri |
0.62 |
0.68 |
1.08 |
Kannur |
Chembra |
1.18 |
0.96 |
0.81 |
Thalassery |
0.76 |
0.79 |
1.03 |
Kasaragod |
Manjeshwaram |
0.89 |
0.99 |
1.11 |
Kanchangad |
0.69 |
0.90 |
1.29 |
Chittazhikal |
1.12 |
0.92 |
0.82 |
We have further calculated the indoor to outdoor (I/O) ratios and the values are presented in Table 2. The average I/O ratios observed for the present study was 1.13 which is lower than the average I/O (1.95 ± 20%) values for typical houses in India.19 However, high I/O ratios were obtained for dwellings in Thumpamon (2.13) in Pathanamthitta, Nelliyampathi (1.58) in Palakkad and Vetilapara (1.66) in Thrissur, respectively. The house in Chavara that recorded the lowest I/O ratio (0.59) is located in the high background radiation area of the state. This lower ratio is the result of the contribution from higher outdoor radiation existing in this region. Furthermore, the house was chiefly made of concrete that may have provided a shielding effect on the outdoor radiation.22 Otherwise, one would expect at least same indoor dose values as that of the outdoor, since the outdoor values are anyway high. Therefore, we can conclude that concrete could act as both a shield and a source of radiation depending upon the location of the house.
Table 3 depicts a comparison of mean indoor to outdoor ratios of the present study with different studies across the world. The mean I/O value (1.13) obtained for the present study falls within the range of I/O values measured for 42 countries.13 At the same time, I/O ratios obtained in this study are lower when compared to other studies in Cuba, Spain, Brazil, Ireland, and Austria. Higher background outdoor gamma radiation received in some sites of the study area must be the main reason for the lower I/O ratio. The high background radiation is the result of the presence of monazite sands.22 Generally, houses located at high altitudes have efficient insulation facilities and room heating facilities. This can actually lead to an increase in the concentration of radon particles and the daughter nuclei inside the houses. This may eventually lead to an increase in gamma dose in indoor environments.34 In the case of countries situated near the .equator such as India there is more ventilation in houses, especially for reducing heat inside the houses. Kerala being located in a tropical environment has houses with increased ventilation. This can lead to the reduction of radon levels within the dwellings (radon concentrations are inversely proportional to ventilation).34 In addition, the amount of moisture in the indoor environment of the houses was observed to be quite high during the study period. High humidity could increase the water content on walls, floors and in the atmosphere thereby leading to possible effective shielding of indoor gamma radiation that emanates mainly from within the building materials.35 However, it is difficult to make a general statement on this reduced indoor value in Kerala as in a few study areas, the values are bit high depending on the kind of construction materials used.
Table 3 Comparison of mean I/O (Indoor/Outdoor) ratios with other studies
Study Area |
I/O |
Reference |
Kerala, India |
1.13 |
This study |
Province of Camagüey, Cuba |
1.45 |
30
|
Province of Cáceres, Spain |
1.30 |
31
|
Rio Grande do Norte, Brazil |
1.30 |
32
|
Ireland |
1.48 |
33
|
Austria |
1.65 |
13
|
USCEAR 1993 (mean of 42 countries) |
0.8–2.02 |
13
|
The average annual indoor vs. outdoor gamma dose rate correlation plot (Fig. 2) shows that there is a small positive linear relationship between the indoor and outdoor dosage rates. Here the value for the reciprocal of the slope (average of indoor to outdoor gamma radiation dose) is 1.22. This value agrees well with the value (>1) observed for regions having normal background radiation where U, Th and K exist in the soil in the order of 20, 20 and 400 Bq kg−1, respectively.22 In India, the average indoor to outdoor gamma radiation doses measured particularly in dwellings that have tiled or cemented floors, concrete walls and ceilings, were found to be around 1.2.19 Majority of the houses that were monitored in our study have concrete ceilings, cemented walls and floors.
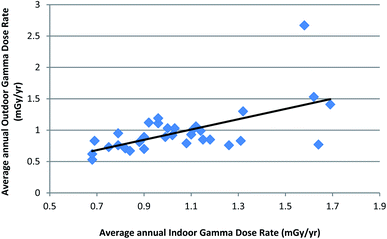 |
| Fig. 2 Average annual indoor vs. outdoor gamma dose rate (mGy per year). | |
The mean indoor value measured in this study is 1.059 mGy (Table 4) which is fairly close to the value obtained for indoor gamma radiation studies at Hungary, Germany and Sweden. However, the observed value (1.059 mGy) exceeds the national (India) average of 0.604 mGy. Compared to the average indoor gamma dose values of countries such as France, Denmark, Poland, Ireland, Netherlands and United Kingdom we have obtained a higher indoor dose level. The large deviation in the observed mean dose value from the national average maybe attributed to the change in the construction pattern specifically in terms of the building materials used for construction of dwellings in the study area. Inclination towards usage of concrete for the construction of dwellings and preference of masonry materials such as granite (mainly exported) for flooring and various other interior applications is on the rise. These factors must have synergistically contributed to the increased gamma dose level observed in indoor environments.
Table 4 Comparison of the mean indoor radiation from the present study with studies in other countries
Study area |
Dose (mGy) |
Reference |
Cosmic radiation excluded.
|
Kerala |
1.059 |
This study |
Indian average |
0.604 |
35
|
Poland |
0.6395 |
36
|
Germany |
0.9986 |
37
|
Hungary |
1.0177 |
38
|
Denmark |
0.7446 |
39
|
France |
0.760 |
40
|
Irelanda |
0.561 |
41
|
Netherlandsa |
0.561 |
42
|
United Kingdoma |
0.543 |
43
|
Swedena |
0.964 |
44
|
3.3. Annual effective doses (AEDs)
AED mainly depends on the construction material of the houses or buildings. It is necessary to measure radiation levels inside indoor environments, as most humans spend considerable amount of time (80%) indoors.45 In order to estimate the Annual Effective Doses (AEDs), one has to take into account the conversion coefficient from the absorbed dose rate in air to the effective dose and the occupancy factor. UNSCEAR recommended outdoor and indoor occupancy factors of 0.2 and 0.8.11 The occupancy factor is the proportion of the total time during which an individual is exposed to a radiation field, implying that 20% of the time is spent outdoors, on average, around the world. The total AED for 32 sites was calculated using the following formula
Total AED (mSv per year) = AED (mSv per year) outdoor + AED (mSv per year) indoor = D (nGy h−1) × 8760 (h per year) × 0.2 × 0.7 (Sv Gy−1) × 10−6 + D (nGy h−1) × 8760 (h per year) × 0.8 × 0.7 (Sv Gy−1) × 10−6 |
In the present study, Chavara area of Kollam district recorded the highest AED value (1.798 mSv per year) while the lowest value (0.65 mSv per year) was observed at Mayanoor area of Thrissur District (Table 5). The presence of monazite rich coastal sand deposits elevates the outdoor gamma radiation levels in certain parts of Kollam district.22,46 Chavara lies close to the high background radiation area of the state. Both annual mean outdoor and indoor gamma radiation level is quite high in Chavara when compared to other monitored sites (Table 5). Mayanoor recorded the lowest annual mean outdoor and indoor gamma radiation levels in the state (Table 5). However, all the monitored sites exceeded the mean AED value (excluding high background regions of Kerala and Tamil Nadu) for India which is estimated to be 0.44 ± 0.13 mSv per year. The mean AED obtained for the present study is 1.04 mSv per year which slightly exceeds the world mean AED value of 1 mSv per year.11 A comparison of the AED values obtained in the present study is made with the values reported from USA, Finland, Cuba and other regions falling under normal background radiations and is given in Table 6. The value obtained from our present study is closer to the value reported from USA.
Table 5 Annual effective dose (AED) level across different sites of Kerala
District |
Location |
AED (mSv per year) |
Kollam |
Chavara |
1.798 |
Kottayam |
Athirampuzha |
1.066 |
Vakathanam |
1.108 |
Ernakulam |
Maradu |
1.000 |
Kuthatukulam |
0.866 |
Muvattupuzha |
0.898 |
Paravur |
1.006 |
Eloor |
0.822 |
Idukki |
Kumali |
1.092 |
Thiruvananthapuram |
Palod |
1.634 |
Neyyatinkara |
1.316 |
Thonakkal |
1.602 |
Kallar |
1.114 |
Alappuzha |
Mavelikara |
1.110 |
Thrissur |
Mayanoor |
0.650 |
Kaipumangalam |
1.090 |
Vetilapara |
1.160 |
Pathanamthitta |
Thumpamon |
1.466 |
Konni |
1.030 |
Palakkad |
Vadakancheri |
1.022 |
Nelliyambathi |
1.214 |
Kozhikode |
Perambra |
0.718 |
Koyilandi |
0.990 |
Malappuram |
Parapanagadi |
0.798 |
Manjeri, payanadu |
0.806 |
Wayanad |
Mananthavadi |
0.746 |
Sulthanbatheri |
0.668 |
Kannur |
Chembra |
1.006 |
Thalassery |
0.784 |
Kasaragod |
Manjeshwaram |
0.970 |
Kanchangad |
0.860 |
Chittazhikal |
0.960 |
Table 6 Comparison of the annual effective dose from background radiations
Study area |
Annual effective dose (AED), mSv per year |
Reference |
Range |
Mean |
Kerala |
0.650–1.798 |
1.042 |
This study |
USA |
0.609–2.459 |
1.060 |
47
|
Finland |
0.701–3.855 |
1.288 |
48
|
Cuba |
0.468–0.630 |
0.510 |
30
|
Regions falling under normal background radiations |
|
0.760 |
13
|
4. Conclusion
The present study aimed at finding out the natural background gamma radiation levels in indoor and outdoor environments across the state of Kerala. It was found that the average annual outdoor natural background radiation dosage at all sites across Kerala ranged from 0.53 to 4.27 mGy per year which is much above the average world annual dosage of 0.48 mGy per year.20 It was found that the southern regions of the state have higher radiation dosage than the other regions. The p.resence of Monazite rich sand deposits in coastal areas of the Kollam district contributes to the high outdoor radiation dosage observed in the region, i.e. the average annual outdoor dosage rate was about 5 times higher than the global average. The high background radiation area of Kollam–Arattupuzha coastal belt recorded the highest value among the study areas. Average annual indoor and outdoor gamma dose rates showed a positive correlation. The indoor to outdoor gamma radiation ratio varied from 0.59 to 2.13. It was found from the indoor to outdoor dosage ratio that the radiation dosage in the indoor environment is higher than that of the outdoor environment in most of the houses. The construction materials used for building the houses may have contributed to this higher indoor dosage. In the last three decades, the construction pattern and building materials for house construction have undergone a significant shift in Kerala, showing a lineage in opting for concrete structures for houses. 90% of the houses under the study have concrete walls and 60% of houses have concrete walls with concrete roof. Since houses devoid of proper ventilation design are on the rise, adequate measures are required for efficient reduction of the indoor radiation.49 This strategy is a key recommendation for the study area to annul the potential ill effects caused as a result of exposure to elevated indoor radiation dose. This study is expected to support carving out a detailed radiation map of the state.
Conflicts of interest
There are no conflicts to declare.
Acknowledgements
Financial Support from the Department of Atomic Energy, Govt of India is gratefully acknowledged.
References
- J. Sannappa, M. S. Chandrashekara, L. A. Sathish, L. Paramesh and P. Venkataramaiah, Radiat. Meas., 2003, 37, 55–65 CrossRef CAS.
-
K. Kovler, H. Friedmann, B. Michalik, W. Schroeyers, A. Tsapalov, S. Antropov, T. Bituh and D. Nicolaides, in Naturally Occurring Radioactive Materials in Construction, ed. W. Schroeyers, Woodhead Publishing, 2017, pp. 13–36, DOI:10.1016/B978-0-08-102009-8.00003-7.
- D. Moeller and L.-s. Sun, Health Phys., 2006, 91, 338–353 CrossRef CAS PubMed.
-
F. X. Roser, G. H. Kegel, L. Hainberger, T. L. Cullen and R. T. Drew, A study of artificial and natural radioactive contamination in brazil, United States, 1964 Search PubMed.
-
D. Bodansky, M. A. Robkin and D. R. Stadler, Indoor radon and its hazards, University of Washington Press, United States, 1987 Search PubMed.
- D. Pressyanov, M. Guelev and B. Sharkov, Atmos. Environ., 1995, 29, 3433–3439 CrossRef CAS.
- R. D. Evans, J. H. Harley, W. Jacobi, A. S. McLean, W. A. Mills and C. G. Stewart, Nature, 1981, 290, 98–100 CrossRef CAS PubMed.
- R. Ramola, M. S. Kandari and R. Rawat, Current Science, 1997, 73, 771–774 Search PubMed.
- F. Steinhäusler, Environ. Int., 1996, 22, 1111–1123 CrossRef.
- M. Doi and S. Kobayashi, Health Phys., 1994, 66, 274–282 CrossRef CAS PubMed.
-
UNSCEAR, Sources and effects of ionizing radiation UNSCEAR 2000 report to the General Assembly, with scientific annexes Volume I: Sources, UN, United Nations, 2000 Search PubMed.
- D. Shahbazi-Gahrouei, M. Gholami and S. Setayandeh, Adv. Biomed. Res., 2013, 2, 65 CrossRef PubMed.
-
UNSCEAR, Sources and effects of ionizing radiation: UNSCEAR 1993 report to the General Assembly with scientific annexes/United Nations Scientific Committee on the Effects of Atomic Radiation, United Nations, New York, 1993 Search PubMed.
- Y.-M. Lin, C.-J. Chen and P.-H. Lin, Environ. Int., 1996, 22, 45–48 CrossRef.
- S. M. H. Pooya, M. R. Dashtipour, A. Enferadi and T. Orouji, J. Environ. Radioact., 2015, 147, 97–99 CrossRef CAS PubMed.
- T. V. Ramachandran, K. P. Eappen and Y. S. Mayya, Int. Congr. Ser., 2005, 1276, 337–338 CrossRef.
- M. S. M. Sanusi, A. T. Ramli, W. M. S. W. Hassan, M. H. Lee, A. Izham, M. N. Said, H. Wagiran and A. Heryanshah, Environ. Int., 2017, 104, 91–101 CrossRef CAS PubMed.
- B. Senthilkumar, V. Dhavamani, S. Ramkumar and P. Philominathan, J. Med. Phys., 2010, 35, 48–53 CrossRef CAS PubMed.
-
K. S. V. Nambi, V. N. Bapat, M. David, V. K. Sundaram, C. M. Sunta and S. D. Soman, Natural background radiation and population dose distribution in India, Bhabha Atomic Research Centre, India, 1986 Search PubMed.
-
UNSCEAR, Sources, effects and risks of ionizing radiation, UN, United Nations, 1988 Search PubMed.
- R. R. K. Nair, B. Rajan, S. Akiba, P. Jayalekshmi, M. K. Nair, P. Gangadharan, T. Koga, H. Morishima, S. Nakamura and T. Sugahara, Health Phys., 2009, 96(1), 55–66 CrossRef CAS PubMed.
- M. P. Chougaonkar, K. P. Eappen, T. V. Ramachandran, P. G. Shetty, Y. S. Mayya, S. Sadasivan and V. Venkat Raj, J. Environ. Radioact., 2004, 71, 275–297 CrossRef CAS.
- R. Bhaskaran, R. C. Damodaran, V. A. Kumar, J. Panakal John, D. Bangaru, C. Natarajan, B. S. Sathiamurthy, J. Mundiyanikal Thomas and R. Mishra, J. Environ. Public Health, 2017, 2017, 1930787 Search PubMed.
- K. Kandiannan, K. S. Krishnamurthy, C. K. Thankamani and S. J. Ankegowda, Journal of Spices and Aromatic Crops, 2018, 45–53 Search PubMed.
- M. Pérez, E. Chávez, M. Echeverría, R. Córdova and C. Recalde, Radiat. Phys. Chem., 2018, 146, 73–76 CrossRef.
- M. F. Máduar and G. Hiromoto, Radiat. Prot. Dosim., 2004, 111, 221–228 CrossRef PubMed.
- A. Clouvas, S. Xanthos and M. Antonopoulos-Domis, Radiat. Prot. Dosim., 2001, 94, 233–245 CrossRef CAS PubMed.
- S. Mehdizadeh, R. Faghihi and S. Sina, Nukleonika, 2011, 56(4), 363–368 CAS.
- A. S. Alghamdi, K. A. Aleissa and I. F. Al-Hamarneh, Heliyon, 2019, 5, e01133 CrossRef PubMed.
-
O. Brígido, A. Caballero, A. Montalvan, M. García and D. Perez-Sanchez, Environmental gamma radiation measurements on province of camagüey, CUBA, 1999 Search PubMed.
- A. Baeza, M. Del Rio, C. Miro and J. Paniagua, J. Environ. Radioact., 1994, 23, 19–37 CrossRef CAS.
- A. Malanca, L. Gaidolfi, V. Pessina and G. Dallara, J. Environ. Radioact., 1996, 30, 55–67 CrossRef CAS.
- I. R. McAulay and P. A. Colgan, Health Phys., 1980, 39, 821–826 CAS.
- W. Burkart, Nucl. Technol., 1983, 60, 114–123 CrossRef CAS.
- R. Sivakumar, S. Selvasekarapandian, N. Mugunthamanikandan and V. M. Raghunath, Appl. Radiat. Isot., 2002, 56, 883–889 CrossRef CAS PubMed.
- T. Niewiadomski, J. Koperski and E. Ryba, Health Phys., 1980, 38, 25–32 CrossRef CAS PubMed.
- H. Pfister and H. Pauly, Radiat. Prot. Dosim., 1984, 7, 279–282 CrossRef CAS.
- I. Nikl and L. B. Sztanyik, Radiat. Prot. Dosim., 1988, 24, 387–389 CrossRef CAS.
- K. Ulbak, B. Stenum, A. Sørensen, B. Majborn, L. Bøtter-Jensen and S. P. Nielsen, Radiat. Prot. Dosim., 1988, 24, 401–405 CrossRef CAS.
- A. Rannou, F. Posny, J. M. Guezengar and C. Madelmont, Radiat. Prot. Dosim., 1984, 7, 317–320 CrossRef CAS.
- I. R. McAulay and J. P. McLaughlin, Sci. Total Environ., 1985, 45, 319–325 CrossRef CAS.
- H. W. Julius and R. Van Dongen, Sci. Total Environ., 1985, 45, 449–458 CrossRef CAS.
- B. M. R. Green, L. Brown, K. D. Cliff, C. M. H. Driscoll, J. C. H. Miles and A. D. Wrixon, Sci. Total Environ., 1985, 45, 459–466 CrossRef CAS.
- L. Mjönes, Radiat. Prot. Dosim., 1986, 15, 131–140 CrossRef.
- S. Selvasekarapandian, K. S. Lakshmi, G. M. Brahmanandhan and V. Meenakshisundaram, Int. Congr. Ser., 2005, 1276, 327–328 CrossRef.
-
S. Fareeduddin, R. G. Garg and H. N. Sethna, Production of nuclear grade thorium nitrate, India, 1959 Search PubMed.
-
A. W. Klement, CRC handbook of environmental radiation, CRC Press, Boca Raton, FL, 1982 Search PubMed.
-
H. Arvela, L. Blomqvist, H. Lemmelae, A. L. Savolainen and S. Sarkkula, Environmental gamma radiation measurements in Finland and the influence of the meteorological conditions after the Chernobyl accident in 1986, Report 951-47-0496-7, Finland, 1987 Search PubMed.
- D. Deva Jayanthi, C. G. Maniyan and S. Perumal, Radiat. Phys. Chem., 2011, 80, 782–785 CrossRef CAS.
Footnote |
† Present Address: Environmental Science and Engineering Department, Indian Institute of Technology (IIT), Mumbai 400076, India. |
|
This journal is © The Royal Society of Chemistry 2022 |