DOI:
10.1039/D1CE01219C
(Paper)
CrystEngComm, 2022,
24, 95-106
Double substitution leads to a highly polymorphic system in 5-methyl-2-m-tolylamino-benzoic acid†
Received
9th September 2021
, Accepted 7th November 2021
First published on 8th November 2021
Abstract
Addition of methyl group(s) to either or both aromatic rings of fenamic acid (FA) afforded three FA derivatives (1–3), designed to investigate the effect on the polymorphic behavior of these compounds exerted by substitution. A relatively comprehensive polymorph screen led to the discovery of four polymorphs for compound 3, for which substitution took place on both aromatic rings, in contrast to the production of either two or one form(s) for compounds 1 and 2, both of which are mono-substituted. The observation indicated that both substitution position and pattern are important in the polymorphism of these compounds. The thermal properties of each system were investigated by differential scanning calorimetry (DSC). Conformational scans and Hirshfeld surface analyses were performed to study the mechanism of polymorphism and the intermolecular interactions contributing to the stability of each crystal form.
1 Introduction
Anthranilic acids are diarylamines with a carboxylic acid functional group on one of the aromatic rings. These compounds have medical applications in a variety of contexts, such as NSAIDs,1,2 analgesics3,4 and antirheumatics,5,6 antibacterial,7,8 antiviral,9 and antitubercular,10,11 and recently have been investigated as therapeutics for amyloid diseases,12 Alzheimer's disease,13,14 and cancer.15,16 These compounds are also fascinating as far as solid-state structures are concerned as some of them are highly polymorphic (Scheme 1). For example, flufenamic acid (FFA) has nine crystallographically characterized polymorphs so far.17 Moreover, a ninth polymorph of tolfenamic acid (TA) was discovered recently.18 Mefenamic acid (MA),19 clonixin (CLX)20 and flunixin (FLX)21 each has three, four and two reported forms, respectively. However, surprisingly, the parent molecule, i.e., fenamic acid (FA) has only one crystal form thus far discovered despite an exhaustive polymorph screening.22–25 Since nearly all anthranilic acids are conformationally flexible to certain degrees, conformational flexibility cannot be the only factor affecting the polymorphic behavior of these compounds, and substitution obviously plays an important role. To account for the polymorphism of some anthranilic acids, Matzger and Price proposed polymorphophore,25,26 a collective ensemble of conformational, steric, and electronic features, which leads to the polymorphic behavior.
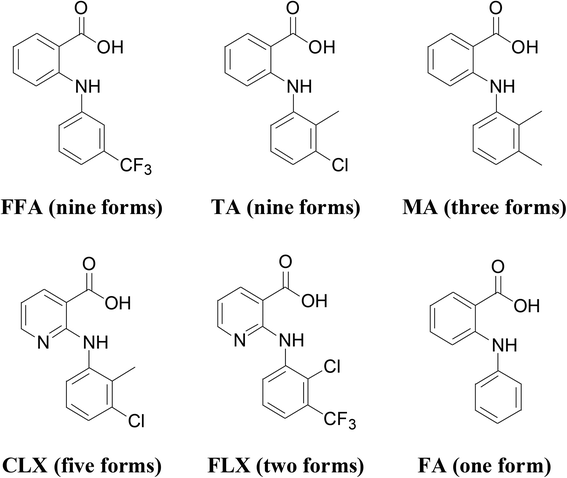 |
| Scheme 1 Molecular structure and number of polymorphs identified for some representative anthranilic acids. | |
Previously, substitution was mainly restricted to the aniline ring of FAs.21,27 In this study, we would like to extend the substitution to the benzoic acid ring to further investigate the role played by substitution patterns. Since there are many potential substitution patterns, for simplicity, we designed three molecules, (1–3), as follows: 1 has a methyl group at the meta position of the aniline; 2 has a methyl at the meta position of benzoic acid; 3 has two methyl groups at the meta position of each aromatic ring (Scheme 2). Compound 1 was first synthesized by the Ullmann coupling of o-chlorobenzoic acid and m-tolylamine in 1908 to investigate its anti-inflammatory activities, and it showed modest activity.28 In 2005, Mei et al. determined the first crystal structure of 1,29 which should be the same as 1-II in this study as the two structures were solved at different temperatures, i.e., the literature structure at 173 K with Z′ = 1, and ours at 90 K with Z′ = 2. Compound 2 was investigated much later because most NSAIDs have the substituents on aniline, and 2 is also an intermediate in the synthesis of acridone derivatives30–32 and quinazolinone derivatives.33,34 Compound 3 was synthesized for the first time in this study. Herein, we report the discovery and characterization of a 2nd form of 1 (1-I), the first crystal structure of 2, and four polymorphs of 3. All crystal forms were fully characterized by single-crystal X-ray diffraction, PXRD, and FT-IR spectroscopy. Their phase behavior was investigated with DSC. To shed light on the role played by substitution in the polymorphism of these compounds, theoretical studies were also performed.
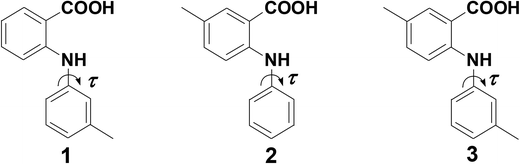 |
| Scheme 2 Structure of compounds 1–3. | |
2 Experimental section
2.1 Materials
Reagents for the synthesis of compounds 1–3 and solvents for the crystal growth were purchased from commercial sources: 2-chlorobenzoic acid, aniline and Cu2O were purchased from Aladdin (Shanghai, China); 2-chloro-5-methyl-benzoic acid was purchased from Bide Pharmatech Ltd (Shanghai, China); m-tolylamine was purchased from Energy Chemical (Shanghai, China); Cu, K2CO3, 2-ethoxyethanol, and the solvents used for crystal growth were purchased from Sinopharm Chemical Reagent Co., Ltd (Shanghai, China), and were used as received.
2.2 Synthesis
Compounds 1–3 were synthesized according to a literature procedure routinely applied in our lab and purified by column chromatography and recrystallization35 (ESI†).
2.3 Characterization
Each compound was characterized by 1H NMR, 13C NMR, mass spectrometry (MS) and IR. NMR spectra were recorded on an Agilent 400/54 Premium Shielded spectrometer (Agilent, USA) in DMSO-d6. The MS was measured using a Thermo LTQ XL, liquid chromatography-mass spectrometer (LC-MS). IR spectra were recorded on a Perkin Elmer FT-IR spectrometer (Perkin Elmer, USA) with samples dispersed in KBr pellets. Thermal analyses were performed on SII instruments DSC6220 (Japan). Tzero pans and aluminum hermetic lids were used to measure a few milligrams of the finely ground sample. A heating rate of 10 °C min−1 was applied.
2.4 Crystallization and structure determination
Slow evaporation was employed for polymorph screening for all three compounds,20 and high-quality crystals were used for structure determination by single-crystal X-ray diffraction.
The crystallographic data of 1-I, 2, 3-II, 3-III, and 3-IV were collected on a Rigaku Oxford diffractometer at ambient temperatures, data for 3-I were collected on a Bruker APEX-II diffractometer at 296 K, and data of 1-II were acquired on a Nonius Kappa CCD diffractometer using a CuKα radiation (λ = 1.54184 Å) at 90 K. Cell refinement and data reduction were performed using either CrysAlisPro,36 Bruker-APEX2,37 or Denzo-SMN,38 respectively. Structure solution and refinement were carried out using the SHELXS39 and SHELXL programs,40 respectively. Powder X-ray diffraction (PXRD) data for the crystal forms were collected on a Rigaku X-ray diffractometer with CuKα radiation (40 kV, 15 mA, λ = 1.5406 Å) between 5.0 and 50.0° (2θ) at ambient temperatures.
2.5 Computational details
Conformation search.
Similar to other FAs, the conformational flexibility potentially arises from N–C (aniline) (Scheme 2). Thus, the energy of different conformations of each molecule defined by the torsion angle, τ, was evaluated with Gaussian 09 (Gaussian, Inc., Wallingford, CT).41 The structures were first optimized from the initial (crystallographic) models in order to identify the most stable conformations, which were then used for scanning each torsion angle, with all bond lengths, bond angles, and other torsion angles fixed. Structural optimization and conformational searches were performed at the M06-2X42/6-311++G(d.p) level of theory. For the conformational search, a scan step size of 10 degrees was used.
Hirshfeld surface analysis.
Hirshfeld surface analyses43 were performed with CrystalExplorer (Version 3.1)44 to further understand the relative contributions to the crystal stability by various intermolecular interactions in the polymorphs of the three compounds.
3 Results and discussion
3.1 Crystal structures
The condition and solvents used for crystallization and the form(s) obtained in individual solvents are listed in Table S1.† Two crystal forms (1-I and 1-II) were obtained for compound 1, one for 2, and four polymorphs (3-I, 3-II, 3-III, and 3-IV) were obtained for 3. Representative crystals of each form are shown in Fig. 1.
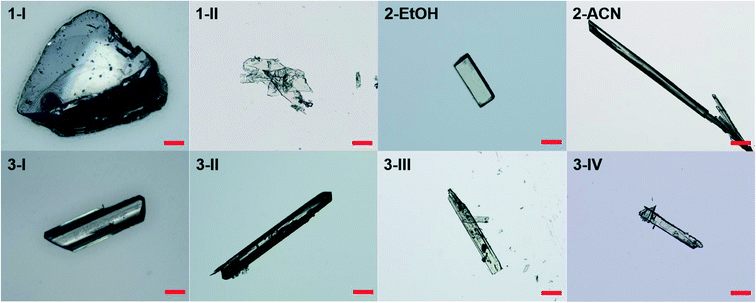 |
| Fig. 1 Representative crystals of different forms of 1–3. Scale bar 0.2 mm. | |
The crystallographic data of all the crystal forms are listed in Table 1, and complete CIF files are provided in the ESI.†
Table 1 Crystallographic data of all crystal forms of compounds 1–3
|
1-I
|
1-II
|
2
|
3
-I
|
3
-II
|
3
-III
|
3
-IV
|
Formula |
C14H13NO2 |
C14H13NO2 |
C14H13NO2 |
C15H15NO2 |
C15H15NO2 |
C15H15NO2 |
C15H15NO2 |
Formula weight |
227.25 |
227.25 |
227.25 |
241.28 |
241.28 |
241.28 |
241.28 |
Crystal size (mm) |
0.15 × 0.08 × 0.07 |
0.20 × 0.20 × 0.05 |
0.20 × 0.05 × 0.05 |
0.2 × 0.2 × 0.1 |
0.04 × 0.03 × 0.02 |
0.04 × 0.03 × 0.02 |
0.05 × 0.03 × 0.02 |
Crystal system |
Monoclinic |
Triclinic |
Triclinic |
Monoclinic |
Triclinic |
Monoclinic |
Monoclinic |
Space group |
P21/c |
P![[1 with combining macron]](https://www.rsc.org/images/entities/char_0031_0304.gif) |
P![[1 with combining macron]](https://www.rsc.org/images/entities/char_0031_0304.gif) |
P21/c |
P![[1 with combining macron]](https://www.rsc.org/images/entities/char_0031_0304.gif) |
P21/n |
P21/c |
a/Å |
11.89630(10) |
8.6511(2) |
7.6873(3) |
12.0066(19) |
7.2703(3) |
9.6103(8) |
11.9463(5) |
b/Å |
7.77840(10) |
9.2344(2) |
9.8086(5) |
15.844(3) |
11.7920(5) |
4.1942(4) |
14.7794(6) |
c/Å |
12.82620(10) |
15.2887(4) |
15.7011(7) |
6.9519(11) |
15.9516(5) |
31.194(3) |
7.4808(3) |
α/° |
90 |
87.8734(10) |
97.313(4) |
90 |
111.362(3) |
90 |
90 |
β/° |
95.1240(10) |
80.979(1) |
93.706(3) |
102.663(2) |
91.144(3) |
91.776(8) |
106.083(4) |
γ/° |
90 |
69.9465(10) |
94.222(4) |
90 |
91.912(4) |
90 |
90 |
Z, Z′ |
4, 1 |
4, 2 |
4, 2 |
4, 1 |
4, 2 |
4, 1 |
4, 1 |
V/Å3 |
1182.12(2) |
1132.96(5) |
1167.78(9) |
1269.1(5) |
1272.12(9) |
1256.72(18) |
1269.11(9) |
D
cal/g cm−3 |
1.277 |
1.332 |
1.293 |
1.242 |
1.260 |
1.275 |
1.263 |
T/K |
299.87(10) |
90.0(2) |
293(2) |
296.15 |
297.25(11) |
297.45(10) |
297.64(10) |
Abs coeff (mm−1) |
0.693 |
0.090 |
0.702 |
0.083 |
0.673 |
0.681 |
0.674 |
F(000) |
480 |
480 |
480 |
512.0 |
512.0 |
512.0 |
512.0 |
Range (deg) |
6.663–77.409 |
2.348–27.573 |
4.561–66.720 |
3.476–64.106 |
5.952–155.178 |
5.67–155.706 |
7.702–155.154 |
Limiting indices |
−14 ≤ h ≤ 15 |
−11 ≤ h ≤11 |
−9 ≤ h ≤ 7 |
−17 ≤ h ≤ 17 |
−9 ≤ h ≤ 9 |
−11 ≤ h ≤ 11 |
−15 ≤ h ≤ 15 |
−9 ≤ k ≤ 8 |
−11 ≤ k ≤12 |
−11 ≤ k ≤ 11 |
−21 ≤ k ≤ 23 |
−14 ≤ k ≤ 14 |
−5 ≤ k ≤ 3 |
−17 ≤ k ≤ 18 |
−15 ≤ l ≤15 |
−19 ≤ l ≤ 19 |
−18 ≤ l ≤ 18 |
−10 ≤ l ≤ 10 |
−19 ≤ l ≤ 14 |
−39 ≤ l ≤ 36 |
−9 ≤ l ≤ 5 |
Completeness to 2θ |
92.7% |
99.3% |
98.4% |
93.5% |
93.0% |
93.1% |
92.0% |
Unique reflections |
2149 |
2958 |
3169 |
2880 |
3584 |
1777 |
1908 |
R
1[I > 2σ(I)] |
0.0392 |
0.0579 |
0.0598 |
0.0507 |
0.0533 |
0.0659 |
0.0482 |
wR2 (all data) |
0.1145 |
0.1859 |
0.1856 |
0.0788 |
0.1729 |
0.2135 |
0.1439 |
CSD accession code |
2108380
|
2108381
|
2108382
|
2108383
|
2108385
|
2108387 |
2108388
|
The crystallographically independent molecules in all forms are twisted to different degrees as evidenced by the dihedral angle between the two aromatic rings (Table 2). The superposition of the different conformations in compounds 1–3 is provided in Fig. 2.
Table 2 Values of the dihedral angle, τ, between the two aromatic rings of the molecules in the crystal structures of 1–3
1
-I
|
1
-II
|
2
|
3
-I
|
3
-II
|
3
-III
|
3
-IV
|
56.09 (3)° |
A: 60.07 (5)° |
A: 33.79 (6)° |
56.60 (3)° |
A: 31.39 (6)° |
47.76 (8)° |
49.25 (6)° |
B: 44.93 (6)° |
B: 73.28 (5)° |
B: 50.29 (5)° |
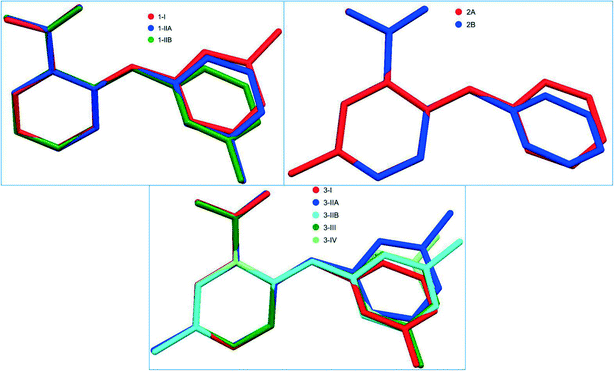 |
| Fig. 2 Superposition of the crytallographically independent molecules in different forms of each system of 1–3. | |
The three crystallographically independent molecules, one for 1-I and two for 1-II, are highly twisted to varying degrees (Table 2 and Fig. 2). Two identical molecules of 1-I associated with each other to form an acid–acid dimer, and the two conformationally different molecules of 1-II paired up to form an acid–acid dimer (Fig. 3). The corresponding intermolecular hydrogen bond parameters were: 1.827 Å of bond length and 178.01° of bond angle for 1-I; 1.748 Å and 174.08° when 1-IIA (red) was the hydrogen bond donor (HBD), and 1.692 Å and 173.03° when 1-IIA was the hydrogen bond acceptor (HBA). The intramolecular hydrogen bond had the following bond parameters: 1.965 Å and 136.28° for 1-I; and 1.897 Å and 138.46° for 1-IIA, and 1.982 Å and 135.04° for 1-IIB (blue).
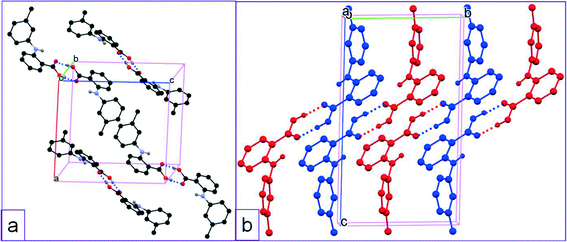 |
| Fig. 3 Crystal packing of a) 1-I, and b) 1-II (for clarity, H atoms not involved in hydrogen bonding are omitted). | |
The two crystallographically asymmetric molecules (A: red; B: blue) in 2 are both nonplanar with the two aromatic rings tilted towards each other to varying degrees (Table 2 and Fig. 2). An acid–acid dimer is formed between A and B (Fig. 4). The intermolecular hydrogen bonds have the following bond parameters: 1.834 Å and 174.34° where molecule A serves as the HBD, and 1.814 Å and 173.38° when A is the HBA. The intramolecular hydrogen bond between NH and carbonyl of the carboxyl have bond parameters of 1.958 Å and 139.23° for A; and 2.010 Å and 134.12° for B. The overall packing of 2 is very similar to that of 1-II as the molecular packing of each is viewed along the a, b, and c axes although the substitution positions are different (Fig. 5).
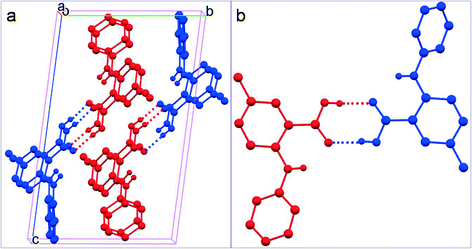 |
| Fig. 4 a) Crystal packing of 2, and b) acid–acid dimer motif (for clarity, H atoms not involved in hydrogen bonding are omitted). | |
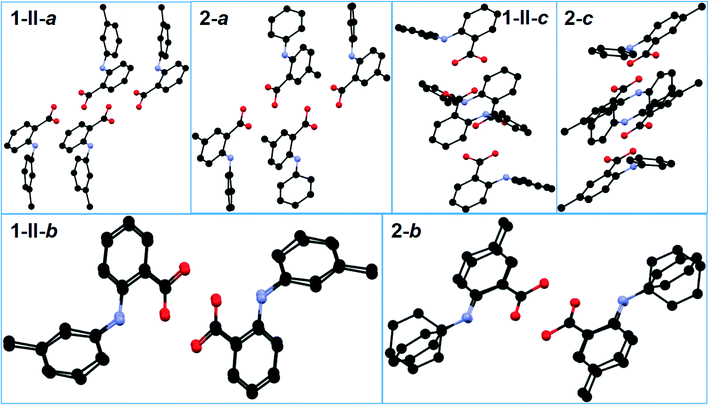 |
| Fig. 5 A comparison between the packing of 1-II and 2 viewed along the a, b, and c axes. | |
All five conformations in the four polymorphs of 3 are twisted to different degrees (Table 2 and Fig. 2). The acid–acid dimer is the only observed hydrogen bonding motif in all forms (Fig. 6). For 3-I, 3-III, and 3-IV, the dimer is between identical molecules of each form, and for 3-II, the dimer is between two conformationally different molecules (A: red; B: green). The hydrogen bonding parameters for intermolecular hydrogen bonds are: 1.831 Å for bond length and 171.73° for bond angle for 3-I; 1.8 Å and 175.84° when the OH of the carboxylic acid of molecule A is HBD, and 1.819 Å and 176.76° when the carboxylic acid OH of molecule B serves as HBD for 3-II; 1.819 Å and 172.93° for 3-III; 1.837 Å and 173.81° for 3-IV. The same intramolecular hydrogen bond between NH and the C
O of the carboxylic acid has bond parameters of 2.046 Å and 129.99° for 3-I; 1.942 Å and 140.50° for 3-IIA and 2.009 Å and 133.13° for 3-IIB; 2.012 Å and 132.54° for 3-III; and 1.981 Å and 136.29° for 3-IV. Structural similarity is observed between 3-I and 3-IV, when the packing of each is viewed along the a, b, and c axes (Fig. 7).
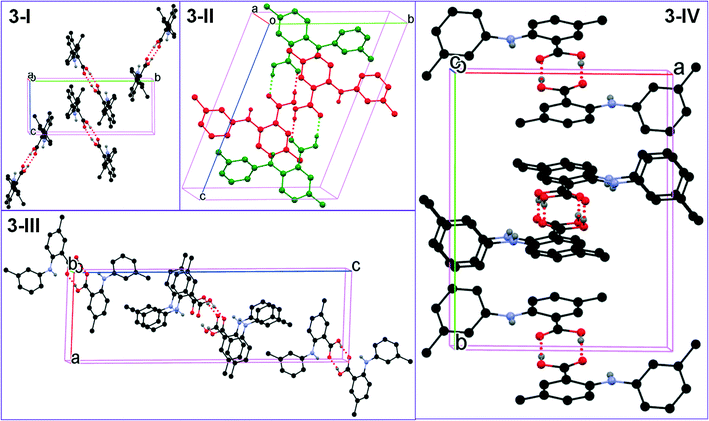 |
| Fig. 6 Crystal packing of the four forms of 3 (for clarity, H atoms not involved in hydrogen bonding are omitted). | |
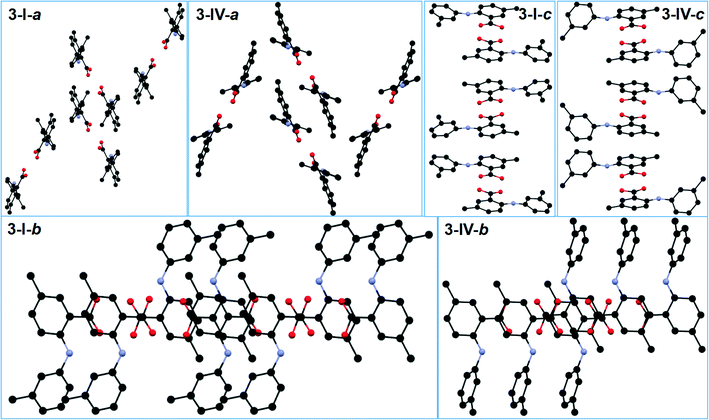 |
| Fig. 7 A comparison between the packing of 3-I and 3-IV viewed along the a, b and c axes. | |
3.2 Phase purity and thermal properties
Phase purity of the crystal forms was investigated by PXRD. The experimental and calculated PXRD patterns of each form were compared side-by-side. The high correspondence indicated that phase-pure samples of each crystal form were obtained. The polymorphic system of compound 3 is given as an example (Fig. 8). The mapping of experimental and calculated PXRD patterns of compounds 1 and 2 is provided in the ESI.†
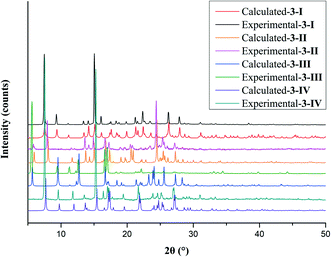 |
| Fig. 8 Experimental and calculated PXRD patterns of the four polymorphs of compound 3. | |
The thermal properties of the crystal forms of 1–3 were studied by DSC. The two polymorphs of 1, i.e.,1-I and 1-II had one endothermic peak on the DSC trace with onset temperatures of 139.3 °C and 137.3 °C, respectively, which corresponds to melting (Fig. S6†). For 2, only one thermal event was detected by DSC, i.e., the melting of the sample at an onset temperature of 184.3 °C (Fig. S7†). For 3-I and 3-II, the DSC traces showed only one thermal event with onset temperatures of 170.3 °C and 174.2 °C, respectively, which were the melting of the samples. 3-III had two thermal events, one exothermic event with an onset temperature of 124.8 °C, and the other an endothermic event with an onset temperature of 173.2 °C. It is demonstrated by PXRD that the first thermal event is a phase transition into 3-I (Fig. S8†). 3-IV also has two endothermic DSC peaks. The first, with an onset temperature of 151.3 °C, appears to be a phase transition to 3-I that melts at approximately 170.9 °C (Fig. 9).
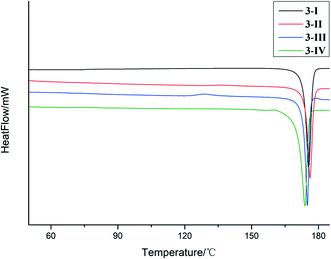 |
| Fig. 9 DSC thermograms of the polymorphs of compound 3. | |
3.3 Computational analyses
Free rotation along the N–C (aniline) bond is allowed in all three compounds, but the observed conformations in the crystal forms are not necessarily conformers; they could be just conformational adjustments.45 Conformational polymorphism is widely (but not necessarily) observed in conformationally flexible molecules. Conformational scans could distinct whether the conformations are conformational adjustments or conformational changes.46 The conformational scan over τ for a single compound 1 molecule is illustrated in Fig. 10. The global minimum is located at 145° and there are three local minima at 45°, −40°, and −135°, respectively. The experimental conformations are found in two energy wells. 1-IIA and 1-IIB are in the same minimum, and 1-I in another. Thus, 1-I and 1-IIA/1-IIB are conformers, and 1-IIA and 1-IIB are merely conformational adjustments.
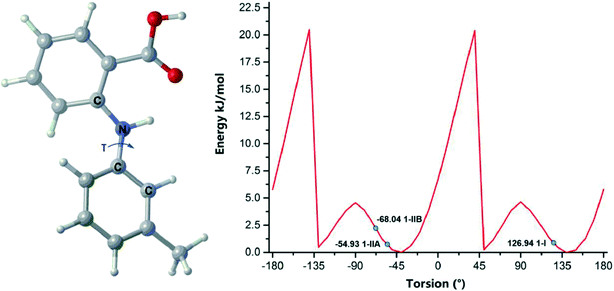 |
| Fig. 10 Ball and stick model of 1 and conformational scan of single molecule. | |
The global minimum of 2 is identified at −35°, and another local minimum is found at around 50°. The two conformations of 2 are in the same energy well (Fig. S9†).
The global minimum of 3 is at −25°. Three local minima are identified at −125°, 50°, and 145°, respectively. The experimental conformations are located in two energy wells, and one is at a maximum. 3-I and 3-IV are conformational changes to each other; 3-IIA and 3-IIB are also related by conformational adjustment; while 3-IV, 3-I/3-III, and 3-IIA/3-IIB are conformers (Fig. 11).
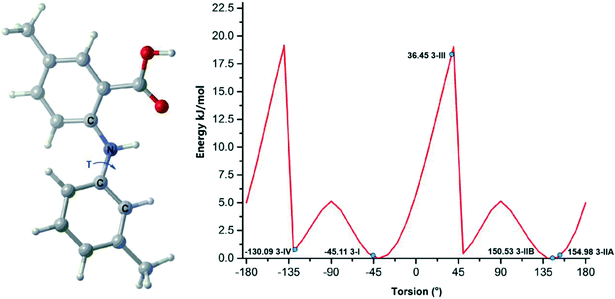 |
| Fig. 11 Ball and stick model of 3 and conformation scan of the single molecule. | |
3.4 Hirshfeld analysis
The Hirshfeld analysis results are shown in Fig. 12, 13 and S10.† It is evident that in all crystal forms, hydrogen–hydrogen contacts predominated, contributing to more than 50% of the overall intermolecular interactions. The second most significant intermolecular interaction in all forms is C⋯H, resulting in more than 20% of the sum of the intermolecular interactions, except for 3-III. H⋯O interactions are the third most important intermolecular contacts, contributing significantly to the total intermolecular interactions. The difference of 1-I and 1-II is demonstrated by the variety of intermolecular interactions shown by the Hirshfeld analysis. At the same time, the structural similarity between 3-I and 3-IV is shown by the variety and relative contribution of the intermolecular interactions (Fig. 13).
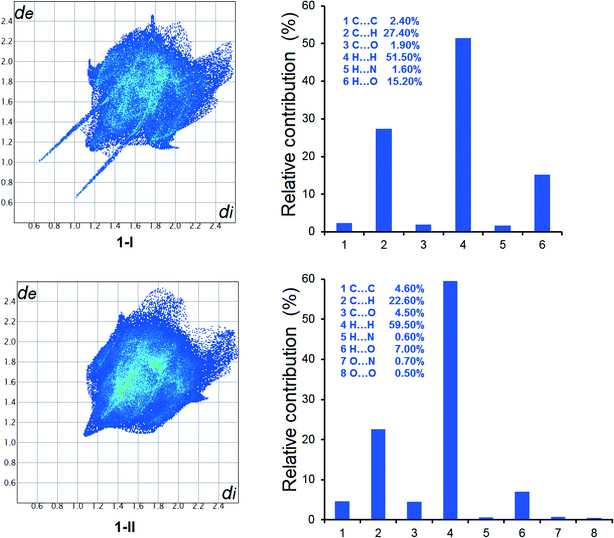 |
| Fig. 12 2D fingerprint plots of Hirshfeld surface and relative contributions to the Hirshfeld surface by various intermolecular contacts in the polymorphs of 1. | |
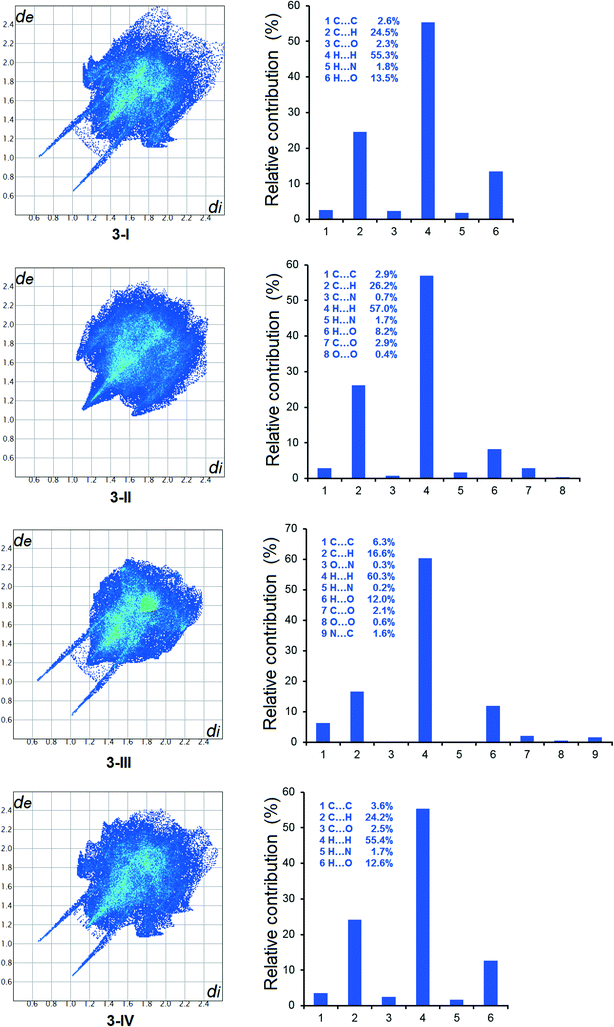 |
| Fig. 13 2D fingerprint plots of Hirshfeld surface and relative contributions to the Hirshfeld surface by various intermolecular contacts in the polymorphs of 3. | |
4 Conclusions
Three fenamic acid derivatives were synthesized by an Ullmann reaction. The compounds differed from FA and each other in the substitution position and pattern. Polymorph screening in commonly used solvents generated two forms for compound 1, one form for 2 and four forms for 3. Double substitution led to the highly polymorphic system of 3 likely due to both packing variability and conformational flexibility. Structural similarity was observed between 1-II and 2, and 3-I and 3-IV. No phase transitions between the two forms of 1 were detected by DSC studies, but DSC studies revealed phase transitions between the four forms of 3. PXRD demonstrated that 3-III and 3-IV were transferred into 3-I after a thermal treatment. Conformational scans of compounds 1 and 3 suggested that the two polymorphic systems are of conformational polymorphism. Hirshfeld analysis revealed that different intermolecular interactions contributed to the overall stability of each crystal form, and the difference/similarity of structurally different/similar structures. In this study, we only investigated the substitution at one given position. The effects of changing the substitution pattern on the solid-state properties of the compounds are currently under investigation.
Conflicts of interest
There are no conflicts of interest to declare.
Acknowledgements
SL thanks Natural Science Foundation of Hubei Province for the financial support (2014CFB787). YT thanks the sponsorship from the Innovation Fund of the Graduate School (CX2020034). PZ is grateful to the Hubei Key Laboratory of Novel Reactor and Green Chemical Technology for the sponsorship (K202007).
References
- R. N. Brogden, Non-steroidal anti-inflammatory analgesics other than salicylates, Drugs, 1986, 32, 27 CrossRef PubMed.
- Y. Masubuchi, H. Saito and T. Horie, Structural requirements for the hepatotoxicity of nonsteroidal anti-inflammatory drugs in isolated rat hepatocytes, J. Pharmacol. Exp. Ther., 1998, 287, 208 CAS.
- O. Fadeyi, C. Obafemi, C. Adewunmi and E. Iwalewa, Antipyretic, analgesic, anti-inflammatory and cytotoxic effects of four derivatives of salicylic acid and anthranilic acid in mice and rats, Afr. J. Biotechnol., 2004, 3, 426 CrossRef CAS.
- P. Stableforth, Mefenamic acid and dextropropoxyphene with paracetamol as analgesics in the accident department, Curr. Med. Res. Opin., 1977, 5, 189 CrossRef CAS.
- L. Nyfos, A comparative clinical study of a new antirheumatic agent, tolfenamic acid (Clotam), and Phenylbutazone in rheumatoid arthritis, Scand. J. Rheumatol., 1979, 8, 5 CrossRef.
- M. E. Mavrikakis, M. Macleod, W. Watson Buchanan, L. A. Hernandez and J. Norris Rennie, Mefenamic acid: an under-rated antirheumatic?, Curr. Med. Res. Opin., 1977, 4, 535 CrossRef CAS.
- A. Thorarensen, J. Li, B. D. Wakefield, D. L. Romero, K. R. Marotti, M. T. Sweeney, G. E. Zurenko and R. W. Sarver, Preparation of novel anthranilic acids as antibacterial agents: Extensive evaluation of structural and physical properties on antibacterial activity and human serum albumin affinity, Bioorg. Med. Chem. Lett., 2007, 17, 3113 CrossRef CAS PubMed.
- S. H. Mahdi, Spectroscopic, structural and antibacterial activity of mixed ligand complexes from schiff base with anthranilic acid, J. Phys.: Conf. Ser., 2019, 1234, 012089 CrossRef.
- A. D. Inglot, Comparison of the antiviral activity in vitro of some non-steroidal anti-inflammatory drugs, J. Gen. Virol., 1969, 4, 203 CrossRef CAS.
- D. Kovala-Demertzi, V. Dokorou, A. Primikiri, R. Vargas, C. Silvestru, U. Russo and M. A. Demertzis, Organotin meclofenamic complexes: synthesis, crystal structures and antiproliferative activity of the first complexes of meclofenamic acid-novel anti-tuberculosis agents, J. Inorg. Biochem., 2009, 103, 738 CrossRef CAS.
- H. Iqbal, S. Ali and S. Shahzadi, Antituberculosis study of organotin (IV) complexes: A review, Cogent Chem., 2015, 1, 1029039 CrossRef.
- L. J. Simons, B. W. Caprathe, M. Callahan, J. M. Graham, T. Kimura, Y. Lai, H. LeVine III, W. Lipinski, A. T. Sakkab and Y. Tasaki, The synthesis and structure-activity relationship of substituted N-phenyl anthranilic acid analogs as amyloid aggregation inhibitors, Bioorg. Med. Chem. Lett., 2009, 19, 654 CrossRef CAS.
- A. Paul, K. C. Nadimpally, T. Mondal, K. Thalluri and B. Mandal, Inhibition of Alzheimer's amyloid-β peptide aggregation and its disruption by a conformationally restricted α/β hybrid peptide, Chem. Commun., 2015, 51, 2245 RSC.
- P. Chatterjee, H. Zetterberg, K. Goozee, C. K. Lim, K. R. Jacobs, N. J. Ashton, A. Hye, S. Pedrini, H. R. Sohrabi and T. Shah, Plasma neurofilament light chain and amyloid-β are associated with the kynurenine pathway metabolites in preclinical Alzheimer's disease, J. Neuroinflammation, 2019, 16, 1 CrossRef.
- A. S. Culf, H. Yin, S. Monro, A. Ghosh, D. A. Barnett, R. J. Ouellette, M. Čuperlović-Culf and S. A. McFarland, A spectroscopic study of substituted anthranilic acids as sensitive environmental probes for detecting cancer cells, Bioorg. Med. Chem., 2016, 24, 929 CrossRef CAS PubMed.
- M. Hiroi, M. Onda, E. Uchida and T. Aimoto, Anti-tumor effect of N-[3, 4-dimethoxycinnamoyl]-anthranilic acid (tranilast) on experimental pancreatic cancer, J. Nippon Med. Sch., 2002, 69, 224 CrossRef CAS PubMed.
- V. López-Mejías, J. W. Kampf and A. J. Matzger, Nonamorphism in flufenamic acid and a new record for a polymorphic compound with solved structures, J. Am. Chem. Soc., 2012, 134, 9872 CrossRef.
- P. Sacchi, S. M. Reutzel-Edens and A. J. Cruz-Cabeza, The unexpected discovery of the ninth polymorph of tolfenamic acid, CrystEngComm, 2021, 23, 3636 RSC.
- S. SeethaLekshmi and T. N. Guru Row, Conformational polymorphism in a non-steroidal anti-inflammatory drug, mefenamic acid, Cryst. Growth Des., 2012, 12, 4283 CrossRef CAS.
- S. Long, T. Mao, P. Chen, M. Liu, S. Parkin, M. Zhang, T. Li, P. Zhou and F. Yu, Strong hydrogen bond leads to a fifth crystalline form and polymorphism of clonixin, ChemistrySelect, 2017, 2, 4942 CrossRef CAS.
- H. Liu, X. Yang, S. Wu, M. Zhang, S. Parkin, S. Cao, T. Li, F. Yu and S. Long, An investigation of the polymorphism of a potent nonsteroidal anti-inflammatory drug flunixin, CrystEngComm, 2020, 22, 448 RSC.
- S. Paul, An X-Ray Study of N-Phenyl Anthranilic Acid, Indian J. Phys., 1968, 42, 668 Search PubMed.
- M. Sbit, L. Dupont, O. Dideberg, J.-F. Liegeois and J. Delarge, Structure de lacide diphénylamino-2-carboxylique, Acta Crystallogr., Sect. C: Cryst. Struct. Commun., 1987, 43, 926 CrossRef.
- T. Zhou, F. Li, Y. Fan, W. Song, X. Mu, H. Zhang and Y. Wang, Hydrogen-bonded dimer stacking induced emission of aminobenzoic acid compounds, Chem. Commun., 2009, 3199 RSC.
- V. López-Mejías and A. J. Matzger, Structure-polymorphism study of fenamates: toward developing an understanding of the polymorphophore, Cryst. Growth Des., 2015, 15, 3955 CrossRef.
- O. G. Uzoh, A. J. Cruz-Cabeza and S. L. Price, Is the fenamate group a polymorphophore? Contrasting the crystal energy landscapes of fenamic and tolfenamic acids, Cryst. Growth Des., 2012, 12, 4230 CrossRef CAS.
- M. Liu, G. Shen, Z. Yuan, S. Parkin, F. Yu, M. Zhang, S. Long and T. Li, Substituent electronegativity and isostructurality in the polymorphism of clonixin analogues, Cryst. Growth Des., 2018, 18, 7006 CrossRef CAS.
- M. Whitehouse, Biochemical properties of anti-inflammatory drugs-XI: Structure-action relationship for the uncoupling of oxidative phosphorylation and inhibition of chymotrypsin by N-substituted anthranilates and related compounds, Biochem. Pharmacol., 1967, 16, 753 CrossRef CAS PubMed.
- X. Mei, A. T. August and C. Wolf, Regioselective copper-catalyzed amination of chlorobenzoic acids: synthesis and solid-state structures of N-aryl anthranilic acid derivatives, J. Org. Chem., 2006, 71, 142 CrossRef CAS.
- X. Li, H. Wang and S. Yang, Sc(OTf)3-catalyzed dehydrogenative cyclization for synthesis of N-methylacridones, Org. Lett., 2013, 15, 1794 CrossRef CAS PubMed.
- Z. Zheng, L. Dian, Y. Yuan, D. Zhang-Negrerie, Y. Du and K. Zhao, PhI(OAc)2-mediated intramolecular oxidative aryl-aldehyde Csp2–Csp2 bond formation: metal-free synthesis of acridone derivatives, J. Org. Chem., 2014, 79, 7451 CrossRef CAS PubMed.
- B. Shu, J. Cao, G. Kuang, J. Qiu, M. Zhang, Y. Zhang, M. Wang, X. Li, S. Kang and T.-M. Ou, Syntheses and evaluation of new acridone derivatives for selective binding of oncogene c-myc promoter i-motifs in gene transcriptional regulation, Chem. Commun., 2018, 54, 2036 RSC.
- K.-I. Ozaki, Y. Yamada, T. Oine, T. Ishizuka and Y. Iwasawa, Studies on 4 (1H)-quinazolinones. 5. Synthesis and antiinflammatory activity of 4 (1H)-quinazolinone derivatives, J. Med. Chem., 1985, 28, 568 CrossRef CAS.
- D. Jing, C. Lu, Z. Chen, S. Jin, L. Xie, Z. Meng, Z. Su and K. Zheng, Light-driven intramolecular C-N cross-coupling via a long-lived photoactive photoisomer complex, Angew. Chem., 2019, 131, 14808 CrossRef.
- R. Hu, Y. Zhoujin, M. Liu, M. Zhang, S. Parkin, P. Zhou, J. Wang, F. Yu and S. Long, Solution growth and thermal treatment of crystals lead to two new forms of 2-((2, 6-dimethylphenyl) amino) benzoic acid, RSC Adv., 2018, 8, 15459 RSC.
-
Version: 1.171. 39.13 a, Rigaku Corporation, Oxford, SUK, 2017 Search PubMed.
-
A. Bruker, Topas V4. 2: General profile and structure analysis software for powder diffraction data, Bruker AXS, Karlsruhe, Germany, 2009 Search PubMed.
- W. Minor, M. Cymborowski, Z. Otwinowski and M. Chruszcz, HKL-3000: the integration of data reduction and structure solution-from diffraction images to an initial model in minutes, Acta Crystallogr., Sect. D: Biol. Crystallogr., 2006, 62, 859 CrossRef PubMed.
- G. M. Sheldrick, A short history of SHELX, Acta Crystallogr., Sect. A: Found. Crystallogr., 2008, 64, 112 CrossRef CAS.
- G. M. Sheldrick, Crystal structure refinement with SHELXL, Acta Crystallogr., Sect. C: Struct. Chem., 2015, 71, 3 Search PubMed.
-
M. J. Frisch, G. W. Trucks, H. B. Schlegel, G. E. Scuseria, M. A. Robb, J. R. Cheeseman, G. Scalmani, V. Barone, B. Mennucci, G. A. Petersson, H. Nakatsuji, M. Caricato, X. Li, H. P. Hratchian, A. F. Izmaylov, J. Bloino, G. Zheng, J. L. Sonnenberg, M. Hada, M. Ehara, K. Toyota, R. Fukuda, J. Hasegawa, M. Ishida, T. Nakajima, Y. Honda, O. Kitao, H. Nakai, T. Vreven, J. J. A. Montgomery, J. E. Peralta, F. Ogliaro, M. Bearpark, J. J. Heyd, E. Brothers, K. N. Kudin, V. N. Staroverov, T. Keith, R. Kobayashi, J. Normand, K. Raghavachari, A. Rendell, J. C. Burant, S. S. Iyengar, J. Tomasi, M. Cossi, N. Rega, J. M. Millam, M. Klene, J. E. Knox, J. B. Cross, V. Bakken, C. Adamo, J. Jaramillo, R. Gomperts, R. E. Stratmann, O. Yazyev, A. J. Austin, R. Cammi, C. Pomelli, J. W. Ochterski, R. L. Martin, K. Morokuma, V. G. Zakrzewski, G. A. Voth, P. Salvador, J. J. Dannenberg, S. Dapprich, A. D. Daniels, O. Farkas, J. B. Foresman, J. V. Ortiz, J. Cioslowski and D. J. Fox, Gaussian 09, Revision D.01, Gaussian, Inc., Wallingford, CT, 2013 Search PubMed.
- Y. Zhao and D. G. Truhlar, The M06 suite of density functionals for main group thermochemistry, thermochemical kinetics, noncovalent interactions, excited states, and transition elements: two new functionals and systematic testing of four M06-class functionals and 12 other functionals, Theor. Chem. Acc., 2008, 120, 215 Search PubMed.
- M. A. Spackman and D. Jayatilaka, Hirshfeld surface analysis, CrystEngComm, 2009, 11, 19 RSC.
-
S. Wolff, D. Grimwood, J. McKinnon, M. Turner, D. Jayatilaka and M. Spackman, CrystalExplorer (Version 3.1), University of Western Australia, 2012 Search PubMed.
- F. H. Allen, S. E. Harris and R. Taylor, Comparison of conformer distributions in the crystalline state with conformational energies calculated by ab initio techniques, J. Comput.-Aided Mol. Des., 1996, 10, 247 CrossRef CAS PubMed.
- A. J. Cruz-Cabeza and J. Bernstein, Conformational polymorphism, Chem. Rev., 2014, 114, 2170 CrossRef CAS.
Footnotes |
† Electronic supplementary information (ESI) available: Synthesis and characterization of the three compounds, crystal growth results in a series of solvents and crystal structure of all the forms in the form of crystallographic information file (CIF) are provided. The structures are deposited in CCDC with accession codes 2108380–2108383, 2108385, 2108287 and 2108388. For ESI and crystallographic data in CIF or other electronic format see DOI: 10.1039/d1ce01219c |
‡ Yunping Zhoujin and Yang Tao contributed to this work equally. |
|
This journal is © The Royal Society of Chemistry 2022 |
Click here to see how this site uses Cookies. View our privacy policy here.