DOI:
10.1039/D2AY00614F
(Paper)
Anal. Methods, 2022,
14, 2689-2697
Sulfonated halloysite nanotubes as a novel cation exchange material for solid phase extraction of toxic pyrrolizidine alkaloids
Received
12th April 2022
, Accepted 10th June 2022
First published on 29th June 2022
Abstract
Pyrrolizidine alkaloids are phytochemicals, which present a highly toxic class of compounds in multiple food resources and are therefore a late-breaking topic in food safety. This study describes the first use of modified halloysite nanotubes as a novel solid material for solid phase extraction. As a result of a fast one-pot sulfonation of the cheap and non-toxic halloysite nanotubes, an efficient cation exchange phase has been prepared. After optimization of the solid phase extraction protocol, high extraction efficiencies and overall recoveries were obtained for a mixture of four pyrrolizidine alkaloid structures through UHPLC-MS/MS analysis with caffeine as the internal standard. Furthermore, the novel solid phase was used for the selective binding of the toxic pyrrolizidine alkaloids in a real-life honey sample, which itself is often contaminated with these compounds. In-house validation showed great extraction efficiencies up to 99.9% for senecionine with a lower limit for lycopsamine with 59.3%, which indicated high selectivity even in the presence of potential interfering compounds. Subsequently, overall recoveries up to 91.5% could be obtained for senecionine while the lowest value was reached for lycopsamine with 55.1%. Comparison with a commercial strong cation exchange tube procedure showed the high competitiveness of the novel solid phase with respect to overall performance. Only slight disadvantages regarding precision and repeatability with values under 5.7% and 11.6% could be observed. Therefore, sulfonated halloysite nanotubes present themselves as an easy to prepare, cheap and highly efficient novel cation exchange material for the selective solid phase extraction of toxic pyrrolizidine alkaloids in frequently contaminated real-life samples like honey.
Introduction
Pyrrolizidine alkaloids are secondary plant metabolites and belong to a compound class with over 660 known, highly diverse structures, which show hepatotoxic and possible carcinogenic properties to humans with over 10
000 reported cases.1–6 Therefore, possible contamination through pyrrolizidine alkaloids in food such as beehive products and herbal teas, but also a variety of animal-derived products, is a highly recent topic in today's food industry.1,4–11 Accidental harvesting of pyrrolizidine alkaloid containing plants, mostly from the family of Asteraceae, Boraginaceae or Fabaceae, is the main source of contamination.4–6,10–12 Regular intake of low contamination concentrations may already cause chronic liver disease.5,12,13 For this reason, the European Commission defined a maximum limit for pyrrolizidine alkaloids in food products varying from 1 to 1000 μg kg−1 coming into effect in July 2022. Furthermore, the maximum value depends on the approximate daily intake of the product.14 Therefore, fast, cheap and easy-to-use methods for the determination and sample preparation of pyrrolizidine alkaloids in food, are crucial. Nevertheless, due to their high structural diversity, toxicity even at low concentrations and possible complex sample matrices, pyrrolizidine alkaloid analysis proves itself as a challenging task.1,15 For this reason, a combination of highly sensitive methods and effective sample preparation is required. Nowadays, the methods of choice are mostly based on liquid chromatography (LC) coupled to a tandem mass spectrometer (MS/MS), since they present highly sensitive and stable methods for quantification of pyrrolizidine alkaloids.1,2,5,10,16–19 Furthermore, there are several published methods that use gas chromatography with MS detection, thin-layer chromatography, immunoaffinity, ultraviolet-spectroscopic methods, nuclear magnetic resonance, capillary electrophoresis, or more recently, direct analysis in real-time mass spectrometry to ensure the sensitive analysis of pyrrolizidine alkaloids.1,6,20 The selection of an appropriate measurement instrument is largely oriented towards the sample matrix. In LC-MS/MS, the selection of the right source for ionization can be crucial, since matrix effects can falsify obtained results.21 Electrospray ionization (ESI) is the most used interface in today's LC-MS hardware.21 However, it shows higher susceptibility with respect to other atmospheric pressure ionization (API) sources.21,22 To assess the order of the matrix effect, post-column infusion and post-extraction addition are the methods of choice, with the latter being widely used for method validation and for this study.21,22 In addition to this, there is also the possibility of solvent dilution and minimizing the injection volume to reduce the matrix effect.21,22 However, this can lead to problems in terms of sensitivity.17 To reduce matrix effects, sample preparation is crucial. For the preparation of samples containing pyrrolizidine alkaloids, different types of solid phase extractions (SPE) can be found in multiple publications.1,6,9,16,18,23–25 Method descriptions of official institutions and several working groups use octadecyl-modified silica for solid phase extractions while other working groups propose treatment with cation exchange solid phase extractions utilizing the weak basic properties of the pyrrolizidine alkaloids.1,9,17,18,23–25 In addition to the solid phase extraction approach, methods using dispersive liquid–liquid microextraction or solid–liquid extractions, in the form of quick, easy, cheap, effective, rugged and safe (QuEChERS) approaches, have already been published with satisfactory extraction results.16,19,26 However, this work presents a new way to extract toxic pyrrolizidine alkaloids with the help of modified halloysite nanotubes (HNTs). They attract great interest in interdisciplinary sciences and apart from their carbon nanotube like structure, they possess multiple advantages.27 Low toxicity, biocompatibility, empty inner cavity and different possibilities for inner–outer surface chemistry, as a reason for their different inner and outer surfaces, are just a few examples.27–30 Structurally, halloysite nanoclay is a two-layered aluminosilicate with water molecules in between and a hollow tubular structure with walls formed through the rolling of 15 to 20 aluminosilicate layers.27,29,31–33 The outer shell, which is built out of silicium dioxide with free hydroxy groups on the surface, can easily be modified and presents itself therefore as an ideal substrate.33 The size of the tubes varies from 50 to 70 nm in external diameter and 10 to 20 nm in lumen diameter. The length lies between 0.5 and 1.5 μm.27,28,31 Furthermore, large specific surface areas as well as great natural resources result in economically favourable prices. Their natural cation exchange capacity of 30–50 × 10−2 mol kg−1 makes this compound compelling for further use as a solid phase material.28,29 Therefore, halloysite nanoclay, also called halloysite nanotubes, was modified through sulfonation to generate a novel cation exchange material for selective extraction of toxic pyrrolizidine alkaloids from standard mixtures and a spiked real-life sample.
Experimental
Materials and methods
Reagents and standards.
Acetonitrile (for LC-MS; minimum 99.95% acetonitrile) and methanol (for LC-MS; minimum 99.95% methanol) were purchased from Chemsolute® (Th. Geyer, Renningen, Germany). Ammonium formate (≥95%) and formic acid (ROTIPURAN® ≥98%, p.a., ACS) were obtained from Carl Roth (Carl Roth GmbH + Co. KG, Karlsruhe, Germany). Chlorosulfonic acid (purum, >98.0% (T)) was purchased from Fluka AG (Honeywell International Inc., Morristown, USA). Thiourea (pro analysis) was obtained from Merck (Merck KGaA, Darmstadt, Germany). Heliotrine (phyproof® Reference Substance, ≥85% (HPLC)), lycopsamine (phyproof® Reference Substance, ≥85% (HPLC)) and senecionine (phyproof® Reference Substance, ≥85% (HPLC)) were purchased from PhytoLab (PhytoLab GmbH & Co. KG, Vestenbergsgreuth, Germany). Caffeine (ReagentPlus®, ≥99%), halloysite nanoclay and monocrotaline (≥98%) were ordered from Sigma-Aldrich (Sigma-Aldrich, St. Louis, USA). Furthermore, purified water from a Merck Millipore Milli-Q™ Reference Ultrapure Water Purification System with deionized water as the feedwater source was used for analysis. Empty 1 mL pre-fritted (polyethylene, 20 μm porosity) polypropylene SPE cartridges were purchased from Sigma-Aldrich. Supel™-Select strong cation exchange (SCX) SPE tubes with a bed weight of 30 mg and a volume of 1 mL were obtained from Merck. Pyrrolizidine alkaloid reference substances were first dissolved in acetonitrile and then further diluted with water to their respective concentration.
Honey sample.
For the analysis of a spiked real-life sample, bee honey from Bergland-Honig (Bergland-Honig GmbH, Urban, Austria) was purchased from a local supermarket. The sample was prepared as mentioned in the following section without spiking. To ensure a pyrrolizidine alkaloid-free matrix, the sample was analyzed with the, later presented, UHPLC-MS/MS method.
Sample preparation
10 g of bee honey was dissolved in 100 mL of Milli-Q™ water in a volumetric flask and vortexed until no honey residue could be observed in the flask. The solution was then centrifuged at 14
000 rpm for 5 minutes and the liquid transferred into a fresh flask. Subsequently, the sample was spiked with an aquatic standard mixture of the pyrrolizidine alkaloids heliotrine, lycopsamine, monocrotaline and senecionine to obtain a final concentration of 12.5 μg L−1 for each pyrrolizidine alkaloid. These pyrrolizidine alkaloids were chosen as they present four of the six basic structures of this compound group. The spike level was adapted to the current regulation of the European Union for pyrrolizidine levels in honey, which must not exceed a maximum value of 500 μg kg−1 of pyrrolizidine alkaloids in pollen-based food supplements, pollen and pollen products.14
Synthesis of sulfonated halloysite nanotubes (HNT-SO3H)
Direct sulfonation of the outer shell of the halloysite nanotubes was achieved through one-pot preparation according to Silva et al. using chlorosulfonic acid for the modification process (Fig. 1). This modification of halloysite nanotubes was chosen due to high sulfur content, high acid capacity and easy one-pot preparation.34 For this, 2 g of halloysite nanoclay was dispersed in 35 mL of dichloromethane for 10 min in a flask equipped with a depressurizing funnel. The suspension was vigorously stirred with a magnetic stirrer and cooled in an ice bath. In the next step, 2 mL of chlorosulfonic acid was added dropwise over a period of 10 min through an air compensation funnel to the still cool suspension. After the sulfonation of the tubes, an increase in the lumen diameter was expected.28,29,33 Afterwards the ice bath was removed and the solution was stirred for an additional 4 hours. Finally, the suspension was centrifuged and decanted to separate the modified solid material from the residual liquid. The modified halloysite nanotubes were washed four times with 10 mL of methanol and subsequently dried for 24 hours at 100 °C.34 The dried powder was then ground with a mortar to ensure that there was no clumping, which would lead to possible channel production during the SPE.
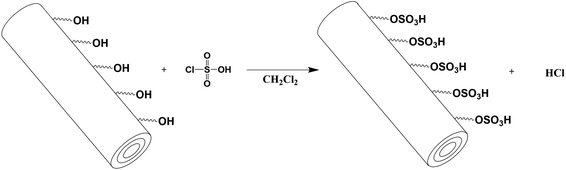 |
| Fig. 1 Sulfonation of halloysite nanotubes through substitution of the hydroxyl group with the sulfonic acid group of chlorosulfonic acid. | |
FE-SEM analysis.
Images of the unmodified and sulfonated HNTs were captured with a JEOL 7610F field emission scanning electron microscope (FE-SEM) (JEOL Ltd., Tokyo, Japan). The SEM pictures were obtained in the high-resolution mode (pressure p ≈ 4 × 10−6 mbar). The HNTs were placed on a graphite glue pad and coated with a thin (30 s deposition time) gold layer (Agar Sputter Coater) to prevent charging. The accelerating voltage was set to 15 keV, and the working distance was between 7 and 9 mm. The secondary electrons were scanned with an in-lens detector.
Solid phase extraction
Pyrrolizidine alkaloid standard mixture.
For the preparation of the SPE cartridges 30 mg of ground, modified halloysite nanotubes were packed into the empty cartridges and covered with an additional polyethylene frit. To ensure reproducibility, which often presents a problem with self-packed cartridges, a force meter with a fitting stamp was used to pack all cartridges with a weight of 12.5 kg. All extractions were then first performed with a 12.5 μg L−1 aquatic mixture of the four pyrrolizidine base structures mentioned before, to ensure a satisfactory working SPE protocol for the real-life sample.
The solvents for the extraction were passed through the solid phase with the help of pressurized air at a pressure of 2.5 bar. For the extraction itself a one-to-one dilution of the samples with the conditioning solvent did not show improved recoveries and was therefore not implemented further in the SPE protocol. Additionally, a drop rate of four to five drops per minute was used in every step of the extraction protocol. Conditioning of the solid phase was performed with 1 mL of a 0.03 mM formic acid solution (pH = 4.5) to ensure the maximum amount of protonated sulfonic acid groups on the halloysite nanotubes. A thin layer of conditioning solvent was left on the first frit to ensure that the solid phase will not dry before loading the sample. For the extraction of the sample, 1 mL of the 12.5 μg L−1 aquatic pyrrolizidine alkaloid mixture was applied onto the solid phase. For the standard mixture no washing step was implemented. Elution was achieved through two portions of 1 mL of 0.1 M ammonium formate in methanol, since a basic elution showed poor recoveries. The extracted samples and the eluates were then dried under vacuum and resuspended in 1 mL of 0.1 mg L−1 methanolic caffeine solution which was used as an internal standard.35–39
Reusability study.
As green chemistry becomes increasingly relevant, the new solid material was tested for reusability. For this purpose, solid phase extraction of the pyrrolizidine standard mixture was carried out as previously described. To purify the solid phase for reuse and to avoid carryover from previous extractions, several washing steps were performed after elution. The first washing step was performed with an additional two portions of 1 mL of the eluent solvent (0.1 M ammonium formate in methanol). Afterwards, two washes with 1 mL of methanol were performed. In order to control the effectiveness of the washing steps, UHPLC-MS/MS analysis of the last wash step was performed, which did not show any traces of pyrrolizidine alkaloids. Finally, the SPE cartridge was pre-dried using compressed air for 3 min before being completely dried at 50 °C for 10 min. This procedure was performed before each replicate cycle, resulting in a total of four SPE cycles using the same cartridge.
Honey sample.
The solid phase extraction of spiked real-life samples was performed identically to the pyrrolizidine alkaloid standard mixture SPE. However, 60 mg of modified halloysite nanotubes showed overall better recoveries than the amount of solid material used for the standard mixture. Furthermore, 1 mL of Milli-Q™ water was initially implemented as a washing step for real-life samples but was discarded after recoveries showed significant decreases (18.67% average recovery for lycopsamine in the washing solution) for the four pyrrolizidine alkaloid basic structures. The extracted samples and the eluates were also dried under vacuum and resuspended in 1 mL of 0.1 mg L−1 methanolic caffeine solution. Finally, all samples were centrifuged at 14
000 rpm for 5 minutes and filtered through a 0.20 μm syringe filter. To evaluate whether a further increase in the amount of phase could achieve even better results, extractions with 90 mg of phase were also carried out in the same manner.
Matrix matched calibration.
For the in-house-validation of the presented method, a matrix matched calibration was prepared. The honey extracts, prepared according to the previously described sample preparation steps, were spiked with an aquatic standard mixture of the four pyrrolizidine alkaloids in a concentration range between 0.0 μg L−1 and 75.0 μg L−1. The calibration samples were then dried under vacuum at 30 °C and resuspended in a 0.1 mg L−1 methanolic caffeine solution. Finally, all samples were centrifuged and filtered through a 0.20 μm syringe filter.
Method comparison with commercial strong cation exchange SPE tubes.
The performance of the presented method using sulfonated halloysite nanotubes as a novel solid phase was compared with a method using commercially available strong cation exchange SPE tubes with respect to overall recovery, precision and repeatability. To guarantee the best possible results for the reference SPE experiments, extractions were carried out according to the enclosed protocol. Therefore, the sample was diluted 1
:
1 with 0.05% formic acid/10 mM ammonium formate buffer (pH = 3.5). The solid phase was then conditioned with 1 mL of methanol followed by 1 mL of 0.05% formic acid/10 mM ammonium formate buffer (pH = 3.5). Subsequently, 1 mL of the sample was loaded and the solid phase was allowed to run dry. Washing was achieved through 1 mL of 0.05% formic acid/10 mM ammonium formate buffer (pH = 3.5) followed by 1 mL of 10% aquatic methanol with 0.05% of formic acid as an acidic modifier to ensure no premature elution. Finally, the compounds of interest were eluted with 1 mL of a 1
:
1 acetonitrile/methanol solution with 5% aquatic ammonia. The eluates were then dried under vacuum and resuspended in 0.5 mL internal standard (0.1 mg L−1 methanolic caffeine solution).
UHPLC-MS/MS analysis
UHPLC-MS/MS analysis was performed on a Waters Acquity Premier liquid chromatograph coupled to a Waters Xevo TQD triple quadrupole. A Thermo Fisher Hypersil Gold™ C18 Selectivity column with the dimensions of 150 × 2.1 mm and a particle size of 1.9 μm was used.23,24 The column temperature was set to 40 °C and the autosampler temperature was set to 25 °C. The injection volume was set to 1 μL. The following gradient method was used with 0.1% formic acid in water (A) and acetonitrile (B) at a flow rate of 0.2 mL min−1: 0–2 min (5% B), 2–15 min (5–50% B), 15–15.10 min (50–100% B), 15.10–16.50 min (100% B), 16.50–16.60 min (100–5% B), and 16.60–20.00 min (5% B). Acquisition was performed with Waters MassLynx. Tuning and creation of a multi reaction monitoring (MRM) method was performed in positive electro spray-mode (ES) through the implemented software IntelliStart from Waters. Nitrogen was used as sheath and auxiliary gas, while argon was used as collision gas. For the measurements the tuning method of monocrotaline was used as a representative of the analyte compound class. For each compound of interest, with the exception of the internal standard caffeine, 3 transitions were chosen and used for quantification. In Table 1, the observed transitions of the four pyrrolizidine alkaloid basic structures and the internal standard caffeine are displayed with the respective retention times, cone and collision voltages. A dead time of 2.0 minutes was determined through the measurement of an aqueous 1 mg L−1 thiourea solution. For this reason, MS acquisition was started after 2.5 min and terminated after 15 minutes to save the mass spectrometer from possible contamination with highly polar substances, for instance, salts and saccharides. Peak detection and integration of the observed chromatographic peaks were performed through the, in MassLynx implemented, software TargetLynx to ensure reproducible data evaluation.
Table 1 Retention times and MS/MS parameters of the observed transitions of the four compounds of interest and the internal standard caffeine
Compound |
R
t
/min |
Precursor ion [M + H]+ |
Product ions |
Cone voltage/V |
Collison voltage/V |
Monocrotaline |
4.5 |
326 |
120; 194; 237 |
58 |
34; 32; 26 |
Lycopsamine |
6.2 |
300 |
93; 138; 156 |
50 |
32; 22; 36 |
Caffeine (IS) |
7.4 |
195 |
41; 137 |
32 |
40; 18 |
Heliotrine |
8.0 |
314 |
120; 138; 156 |
40 |
32; 24; 36 |
Senecionine |
9.2 |
336 |
93; 120; 138 |
60 |
38; 34; 40 |
Results and discussion
Synthesis of sulfonated halloysite nanotubes
FE-SEM analysis.
Fig. 2 shows a direct comparison between the obtained surface electron microscope images of the unmodified and the sulfonated halloysite nanotubes. Therefore, the typical nanotube structure of halloysite nanoclay can be observed for both the unmodified (Fig. 2a) and modified materials (Fig. 2b). Additionally, further examination of the first two magnifications (magnification: ×15
000 and ×25
000) reveals noteworthy higher local proximity of the unmodified nanotubes when compared to the sulfonated halloysite nanotubes. This explains the notably higher backpressure of the unmodified halloysite nanotubes in solid phase extractions, which makes unmodified nanotubes not applicable for SPE. Furthermore, sulfonated halloysite nanotubes display rougher surfaces in comparison with the unmodified tubes. Marbling within the structures can be attributed to the coating with gold. This was necessary to obtain adequate images of the nanostructures.
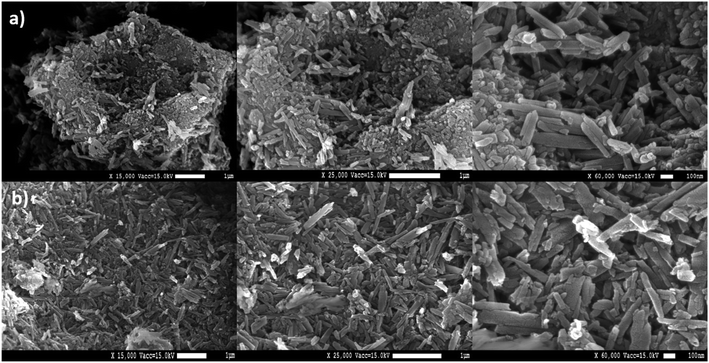 |
| Fig. 2 SEM images of unmodified halloysite nanotubes (a) and sulfonated halloysite nanotubes (b). | |
Solid phase extraction
SPE with unmodified halloysite nanotubes.
For comparison, a solid phase extraction with unmodified halloysite nanoclay, using the same procedure described earlier, was executed. However, no extraction was possible as the resulting back-pressure was too high for the solvents and sample to pass through the extraction cartridge. This indicates a crucial preparation step of the halloysite nanotubes in the form of etching with acid, as performed within the synthesis of the sulfonated halloysite nanotubes. This step could, next to the sulfonation of the halloysite nanotubes, increase the lumen diameter of the tubes significantly and therefore make the material suitable for solid phase extraction.29
Pyrrolizidine alkaloid standard mixture.
Sulfonated halloysite nanotubes showed adequate results for the extraction of the standard mixture of pyrrolizidine alkaloid basic structures. Furthermore, pyrrolizidine alkaloid N-oxides can also be analyzed with a required previous reduction step, which has already been published multiple times.9,16,38,40 In Table 2, the extraction performance of the presented solid phase extraction with modified halloysite nanotubes is shown. Subsequently, the performance of the solid phase extraction with the sulfonated halloysite nanotubes displays high extraction efficiencies with a minimum of 94.1% for lycopsamine. However, for senecionine a nearly complete extraction is observed. Elution of the compounds generally shows excellent results with overall recoveries of 100%, as shown in Fig. 4a, with standard deviations (SD) under 10%. The higher standard deviation of monocrotaline can be reasoned through an apparently less efficient ionization of the compound. The precision of the presented method can be seen as satisfactory with relative standard deviation (RSD) values lower than 8.5% and RSD values below 6.7% for repeatability.
Table 2 Extraction performance of sulfonated halloysite nanotubes for solid phase extraction of a pyrrolizidine alkaloid standard mixture
Monocrotaline |
Lycopsamine |
Heliotrine |
Senecionine |
Extraction efficiency ± SD/% (n = 7)
|
98.8 ± 0.5 |
94.1 ± 0.3 |
98.5 ± 0.3 |
99.61 ± 0.1 |
![[thin space (1/6-em)]](https://www.rsc.org/images/entities/char_2009.gif) |
Recovery ± SD/% (n = 7)
|
107.5 ± 9.0 |
101.3 ± 4.3 |
103.8 ± 4.7 |
100.2 ± 5.2 |
![[thin space (1/6-em)]](https://www.rsc.org/images/entities/char_2009.gif) |
Precision (RSD)/% (n = 7)
|
8.4 |
4.2 |
4.5 |
5.2 |
![[thin space (1/6-em)]](https://www.rsc.org/images/entities/char_2009.gif) |
Repeatability (RSD)/% (n = 7)
|
5.5 |
6.6 |
3.0 |
3.0 |
Reusability study.
In Fig. 3, the results of the reusability study can be seen. It is evident that the sulfonated halloysite nanotubes used show no loss of performance after four solid phase extractions have been performed. Recoveries reveal values between 90.7% and 103.2% with no clear trend towards deterioration with increasing reuse. Therefore, it can be concluded that the presented method can be referred to as a further step towards green chemistry as the modified halloysite nanotubes can be used for at least four extractions with no loss in analytical performance.
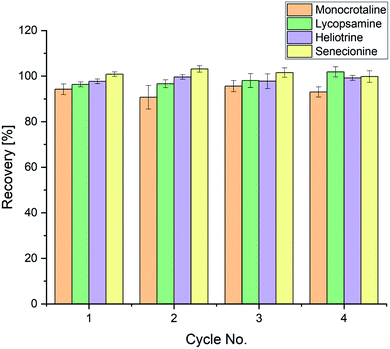 |
| Fig. 3 Recoveries of a 12.5 μg L−1 pyrrolizidine alkaloid standard mixture after reusing the same solid material for four SPE cycles. | |
Honey sample
Analytical performance.
The presented novel solid phase was furthermore tested through an extraction of spiked honey (12.5 μg L−1). Subsequently, the analytical performance of the presented method with modified halloysite nanotubes as the solid phase was in-house-validated in terms of linearity, selectivity, sensitivity, extraction efficiency, overall recovery, precision and repeatability through matrix matched calibration prepared according to the previously presented section. The analytical performance of the method is summarized in Table 3. The presented method shows satisfying linearities for the given concentration range (0.0–75.0 μg L−1), which is indicated through correlation coefficients greater than 0.997. Furthermore, the method exhibits high selectivity and sensitivity, which can be reasoned through the utilization of MRM acquisition with three transitions per pyrrolizidine alkaloid and the utilization of caffeine as the internal standard. As a result, sensitivity with respect to the limit of detection (LOD) and the limit of quantification (LOQ) of the presented method shows appropriate values for the analysis of the spike levels used. However, it is visible that for monocrotaline the LOQ is close to the working concentration, which can again be reasoned through the less efficient ionization for this compound, resulting in a less steep slope and therefore higher standard deviations. The matrix effect (ME) was calculated through the comparison of slopes of a matrix matched calibration and a calibration in an aqueous matrix. For every compound of interest ion enhancement effects are given in the matrix matched samples, with medium matrix effects ranging from [±21.4%] to [±44.7%].41–43 Depending on another reference, the obtained matrix effects can also be classified as soft.19 Therefore, it is not necessary to further consider them in the calibration, with the exception of monocrotaline.19 Nevertheless, the matrix effect of monocrotaline shows an increased value of +44.7% with respect to the other analytes of interest with an averaged matrix effect value of +23.8%. This could be reasoned with the, in general, worse ionization of monocrotaline, with respect to the other compounds. Therefore, the ionization of said compound is amplified even more significantly through the honey matrix. Subsequently, the extraction performance of solid phase extraction with novel sulfonated halloysite nanotubes displays high extraction efficiencies with a lower limit of 59.3% for lycopsamine and values over 93.5% for the remaining three pyrrolizidine alkaloids. Overall recoveries, as shown in Fig. 4b, present a lower limit for lycopsamine with 55.1%. Nevertheless, in general recoveries equal to or higher than 82.0% could be achieved for the remaining compounds of interest with standard deviations below 5%. However, satisfactory selectivity of the novel solid phase towards the pyrrolizidine alkaloids can be reasoned through the high extraction efficiencies and overall recoveries of the four compounds of interest in a spiked sample with other competing compounds.
Table 3 In-house validation of solid phase extraction of a spiked honey sample using sulfonated halloysite nanotubes
|
Monocrotaline |
Lycopsamine |
Heliotrine |
Senecionine |
Ion enhancement.
|
Linearity
|
R
2
|
0.9974 |
0.9988 |
0.9989 |
0.9989 |
![[thin space (1/6-em)]](https://www.rsc.org/images/entities/char_2009.gif) |
Sensitivity/μg L
−1
|
LOD |
4.8 |
3.2 |
3.3 |
3.4 |
LOQ |
12.3 |
7.0 |
6.7 |
7.0 |
Monocrotaline |
Lycopsamine |
Heliotrine |
Senecionine |
Matrix effect/%
|
144.7a |
123.7a |
121.4a |
126.4a |
![[thin space (1/6-em)]](https://www.rsc.org/images/entities/char_2009.gif) |
Extraction efficiency ± SD/% (n = 7)
|
96.6 ± 0.9 |
59.3 ± 1.3 |
93.5 ± 0.3 |
99.9 ± 0.0(3) |
![[thin space (1/6-em)]](https://www.rsc.org/images/entities/char_2009.gif) |
Recovery ± SD/% (n = 7)
|
83.1 ± 4.7 |
55.1 ± 1.7 |
82.0 ± 3.3 |
91.5 ± 4.4 |
![[thin space (1/6-em)]](https://www.rsc.org/images/entities/char_2009.gif) |
Precision (RSD)/% (n = 7)
|
5.6 |
3.2 |
4.0 |
4.7 |
![[thin space (1/6-em)]](https://www.rsc.org/images/entities/char_2009.gif) |
Repeatability (RSD)/% (n = 7)
|
7.3 |
11.5 |
5.5 |
5.0 |
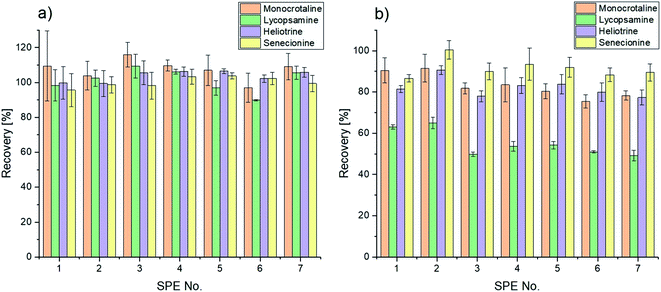 |
| Fig. 4 Overall recoveries and standard deviations of a pyrrolizidine alkaloid mixture (a) and a spiked honey sample (b) using the sulfonated halloysite nanotubes as the novel solid phase. | |
The presented method indicates good precision with RSD values below 5.7% and RSD values smaller than 11.6% for repeatability. When comparing the calculated ME values from Table 3 with matrix effects from previously published methods, no clear trend is observable, since some articles present higher, and others lower values.9,16,26,38,43,44 Some sources even report that no matrix effect could be detected.45 In general, it can be said that the matrix effect in this study is slightly higher than in most other studies. This could be reasoned through the missing washing step, which could not be included due to significant losses in analyte recoveries, as already mentioned before. However, one also finds values that correspond well with the obtained values in this work.44 Nevertheless, it should be noted that the matrix effect varies not only by method, but of course also within a sample type, as honey samples vary by origin and nature and therefore in their chemical composition.21 In addition, a further increase in the amount of solid material showed slightly better extraction efficiencies for all analytes, caused by presumably better binding of the analytes. On average, 8.5% more analyte could be loaded for lycopsamine. However, the eluates only showed a slight improvement of 1.4% for the recovery of monocrotaline compared to the extractions performed with 60 mg of sulfonated halloysite nanotubes.
Conclusions
In this work, sulfonated halloysite nanotubes were introduced as a novel cation exchange material for solid phase extraction of toxic pyrrolizidine alkaloids. Subsequently, the presented method showed satisfactory extraction performance for a standard mixture of pyrrolizidine alkaloids and a spiked honey sample. However, endangered herbal products such as tea could probably also be used as a real-life sample matrix. For the spiked sample, in-house-validation was performed, which showed overall satisfactory results. Furthermore, comparison with a method using commercially available strong cation exchange tubes indicated good competitiveness of the novel method using modified halloysite nanotubes as the solid phase with respect to recovery, precision and repeatability. In addition, the presented method is easier to handle with respect to commercial SCX, as no washing steps have to be carried out. Moreover, the low price of the nanotubes also shows an economic advantage in comparison to the commercial products. Finally, with respect to previous publications, the obtained matrix effects are slightly higher, resulting presumably from the missing washing step. However, better matching values can also be found in published literature. Therefore, solid phase extraction with sulfonated halloysite nanotubes can be seen as a cheap and easier to use method for the highly selective binding of toxic pyrrolizidine alkaloids in honey.
Author contributions
Tobias Schlappack: conceptualization, solid phase extractions, UHPLC-MS/MS analysis, investigation, methodology, validation, visualization, writing – original draft. Matthias Rainer: conceptualization, supervision, project administration, writing – review & editing. Nikolaus Weinberger: field emission scanning electron microscope measurements. Günther K. Bonn: project administration, supervision, writing – review & editing.
Conflicts of interest
There are no conflicts to declare.
Notes and references
- C. Crews, F. Berthiller and R. Krska, Anal. Bioanal. Chem., 2010, 396, 327–338 CrossRef CAS PubMed.
- M. Kempf, M. Wittig, A. Reinhard, K. von der Ohe, T. Blacquière, K.-P. Raezke, R. Michel, P. Schreier and T. Beuerle, Food Addit. Contam., Part A, 2011, 28, 332–347 CrossRef CAS PubMed.
- F. Liu, S. Y. Wan, Z. Jiang, S. F. Y. Li, E. S. Ong and J. C. C. Osorio, Talanta, 2009, 80, 916–923 CrossRef CAS PubMed.
- C. Ma, Y. Liu, L. Zhu, H. Ji, X. Song, H. Guo and T. Yi, Food Chem. Toxicol., 2018, 119, 50–60 CrossRef CAS PubMed.
- D. Schrenk, L. Gao, G. Lin, C. Mahony, P. P. J. Mulder, A. Peijnenburg, S. Pfuhler, I. M. C. M. Rietjens, L. Rutz, B. Steinhoff and A. These, Food Chem. Toxicol., 2020, 136, 111107 CrossRef CAS PubMed.
- N. Casado, S. Morante-Zarcero and I. Sierra, Trends Food Sci. Technol., 2022, 120, 123–139 CrossRef CAS.
-
Fragen und Antworten zu Pyrrolizidinalkaloiden in Lebensmitteln, Bundesinstitut für Risikobewertung, 2020, vol. 1–7 Search PubMed.
-
Pyrrolizidinalkaloide: Gehalte in Lebensmitteln sollen nach wie vor so weit wie möglich gesenkt werden, Bundesinstitut für Risikobewertung, 2016, vol. 1–53 Search PubMed.
- E. Kowalczyk, Z. Sieradzki and K. Kwiatek, Food Anal. Methods, 2018, 11, 1345–1355 CrossRef.
- H. Wiedenfeld and J. Edgar, Phytochem. Rev., 2011, 10, 137–151 CrossRef CAS.
- H. Wiedenfeld, Food Addit. Contam., Part A, 2011, 28, 282–292 CrossRef CAS.
- P. Brugnerotto, S. K. T. Seraglio, M. Schulz, L. V. Gonzaga, R. Fett and A. C. O. Costa, Food Chem., 2021, 342, 128384 CrossRef CAS.
-
Pyrrolizidinalkaloide in Kräutertees und Tees, Bundesinstitut für Risikobewertung, 2013, vol. 1–31 Search PubMed.
-
European Commission, Commission Regulation (EU) 2020/2040 of 11 December 2020 Amending Regulation (EC) No. 1881/2006 as Regards Maximum Levels of Pyrrolizidine Alkaloids in Certain Foodstuffs, 2022 Search PubMed.
-
Analytik und Toxizität von Pyrrolizidinalkaloiden sowie eine Einschätzung des gesundheitlichen Risikos durch deren Vorkommen in Honig, Bundesinstitut für Risikobewertung, 2013, vol. 1–37 Search PubMed.
- R. Celano, A. L. Piccinelli, L. Campone, M. Russo and L. Rastrelli, J. Agric. Food Chem., 2019, 67, 8689–8699 CrossRef CAS.
- S. W. C. Chung and C.-H. Lam, J. Agric. Food Chem., 2018, 66, 3009–3018 CrossRef CAS PubMed.
- Y. He, L. Zhu, J. Ma, L. Wong, Z. Zhao, Y. Ye, P. P. Fu and G. Lin, Environ. Pollut., 2020, 267, 115542 CrossRef CAS PubMed.
- S. Izcara, N. Casado, S. Morante-Zarcero, D. Pérez-Quintanilla and I. Sierra, Food Chem., 2022, 380, 132189 CrossRef CAS PubMed.
- Y. Chen, L. Li, F. Xiong, Y. Xie, A. Xiong, Z. Wang and L. Yang, Food Chem., 2021, 334, 127472 CrossRef CAS PubMed.
- A. Nasiri, R. Jahani, S. Mokhtari, H. Yazdanpanah, B. Daraei, M. Faizi and F. Kobarfard, Analyst, 2021, 146, 6049–6063 RSC.
- W. Zhou, S. Yang and P. G. Wang, Bioanalysis, 2017, 9, 1839–1844 CrossRef CAS PubMed.
-
Bestimmung
von Pyrrolizidinalkaloiden (PA) in Honigmittels SPE-LC-MS/MS, Bundesinstitut für Risikobewertung, 2013, vol. 1–17 Search PubMed.
-
Bestimmung von Pyrrolizidinalkaloiden (PA) in Pflanzenmaterial mittels SPE-LC-MS/MS, Bundesinstitut für Risikobewertung, 2014, vol. 1–17 Search PubMed.
- C. T. Griffin, M. Danaher, C. T. Elliott, D. Glenn Kennedy and A. Furey, Food Chem., 2013, 136, 1577–1583 CrossRef CAS PubMed.
- M. Martinello, A. Borin, R. Stella, D. Bovo, G. Biancotto, A. Gallina and F. Mutinelli, Food Chem., 2017, 234, 295–302 CrossRef CAS PubMed.
- G. Lazzara, G. Cavallaro, A. Panchal, R. Fakhrullin, A. Stavitskaya, V. Vinokurov and Y. Lvov, Curr. Opin. Colloid Interface Sci., 2018, 35, 42–50 CrossRef CAS.
- M. Fizir, P. Dramou, N. S. Dahiru, W. Ruya, T. Huang and H. He, Microchim. Acta, 2018, 185, 389 CrossRef PubMed.
- Y. Lvov, W. Wang, L. Zhang and R. Fakhrullin, Adv. Mater., 2016, 28, 1227–1250 CrossRef CAS PubMed.
- D. Rawtani, G. Pandey, M. Tharmavaram, P. Pathak, S. Akkireddy and Y. K. Agrawal, Appl. Clay Sci., 2017, 150, 293–302 CrossRef CAS.
- R. El-Sheikhy, Sci. Rep., 2020, 10, 21557 CrossRef CAS PubMed.
- J. Nicholson, J. Weisman, C. Boyer, C. Wilson and D. Mills, Appl. Sci., 2016, 6, 265 CrossRef.
- P. Yuan, D. Tan and F. Annabi-Bergaya, Appl. Clay Sci., 2015, 112–113, 75–93 CrossRef CAS.
- S. M. Silva, A. F. Peixoto and C. Freire, Appl. Catal., A, 2018, 568, 221–230 CrossRef CAS.
- R. Chizzola, J. Chromatogr. A, 1994, 668, 427–433 CrossRef CAS.
- L. De Jesus Inacio, R. Merlanti, L. Lucatello, V. Bisutti, B. Contiero, L. Serva, S. Segato and F. Capolongo, Heliyon, 2020, 6, e03593 CrossRef PubMed.
- A. Eller and R. Chizzola, Plant Biosyst., 2016, 150, 1306–1312 CrossRef.
- L. Lorena, M. Roberta, R. Alessandra, M. Clara and C. Francesca, Food Anal. Methods, 2016, 9, 1825–1836 CrossRef.
- M. Martinello, C. Cristofoli, A. Gallina and F. Mutinelli, Food Control, 2014, 37, 146–152 CrossRef CAS.
- L. Joosten, P. P. J. Mulder, K. Vrieling, J. A. van Veen and P. G. L. Klinkhamer, Phytochem. Anal., 2010, 21, 197–204 CrossRef CAS PubMed.
- C. Ferrer Amate, H. Unterluggauer, R. J. Fischer, A. R. Fernández-Alba and S. Masselter, Anal. Bioanal. Chem., 2010, 397, 93–107 CrossRef CAS PubMed.
- P. Kaczyński and B. Łozowicka, J. Pharm. Biomed. Anal., 2020, 187, 113351 CrossRef PubMed.
- L. Lucatello, R. Merlanti, L. De Jesus Inacio, V. Bisutti, L. Montanucci and F. Capolongo, J. Food Compos. Anal., 2021, 104, 104182 CrossRef CAS.
- E. Kowalczyk and K. Kwiatek, Journal of Veterinary Research, 2018, 62, 173–181 CrossRef CAS PubMed.
- C. T. Griffin, S. M. Mitrovic, M. Danaher and A. Furey, Food Addit. Contam., Part A, 2015, 32, 214–228 CrossRef CAS.
|
This journal is © The Royal Society of Chemistry 2022 |