DOI:
10.1039/C9SC02448D
(Edge Article)
Chem. Sci., 2019,
10, 8399-8404
Light-induced metal-free transformations of unactivated pyridotriazoles†
Received
18th May 2019
, Accepted 24th July 2019
First published on 25th July 2019
Abstract
A highly efficient and practical method for incorporation of the arylmethylpyridyl moiety into diverse molecules has been developed. This method features the transition metal-free light-induced room temperature transformation of pyridotriazoles into pyridyl carbenes, which are capable of smooth arylation, X–H insertion, and cyclopropanation reactions. The synthetic usefulness of the developed method was illustrated in a facile synthesis of biologically active molecules.
Transition metal-catalyzed denitrogenative transformations of pyridotriazoles have been recently evolving as a powerful tool for synthesis of diverse molecules possessing N-hetero-cyclic fragments.1,2 These protocols take advantage of the well-known ring-chain tautomerism of the pyridotriazole core in solution into the corresponding diazo tautomer A, which then can be trapped by a transition metal catalyst to form the reactive pyridyl metal carbene intermediate B (Scheme 1a). Since the first report on the transannulation reaction of pyridotriazoles in 2007,3 numerous effective catalytic methods including transannulation,4 X–H insertions,5 and cyclopropanation6 reactions have been developed.7 However, all these methods are not without shortcomings. The reactions proceeding at room temperature require Cl, Br, or OMe activating groups (AG) at C7,3a,b otherwise high temperatures3c–e are necessary for achieving sufficient amounts of the open form of pyridotriazole A, which would lead to the reaction products. In either case, the employment of transition metal catalysts is required. Herein, we report room temperature efficient and operationally simple light-induced metal-free arylation, X–H insertion, and cyclopropanation reactions of pyridotriazoles giving access to various pyridyl-containing synthons, which can be used for convenient synthesis of bioactive molecules.
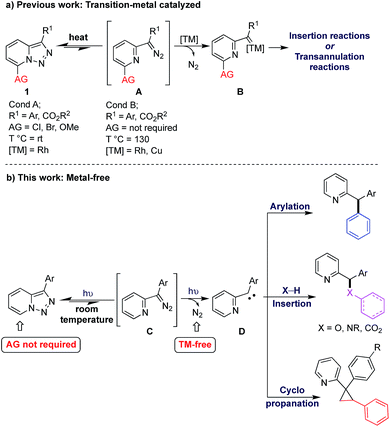 |
| Scheme 1 Thermal and light-induced generation of carbenes from pyridotriazoles. | |
In continuation of our studies on application of pyridotriazoles in the synthesis of nitrogen-containing heterocycles,3a–e we hypothesized a metal-free strategy which can be accessed by utilizing light irradiation.8 Compared with numerous methods reported for thermal processes, photochemical studies of pyridiotriazoles are relatively scarce.9 Aiming at the development of milder reaction conditions, we started our investigation by analysing the UV-vis absorption spectra of pyridotriazoles 1a–d (Fig. 1, see ESI† for more details). Among them, only pyridotriazoles 1a and 1b bearing aryl substituents at the C3 position showed appreciable absorption around 390 nm region, which is attributed to the extended conjugation in these systems. Thus, we hypothesized that upon irradiation, the excited pyridotriazole 1a could undergo a ring-chain tautomerism to deliver its diazo tautomer C (Scheme 1b), which upon denitrogenation10 would deliver reactive carbene species D.
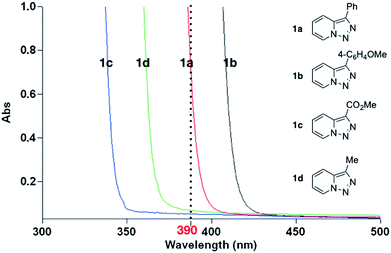 |
| Fig. 1 UV-vis absorption spectra of pyridotriazoles. | |
To test this hypothesis, we examined a metal-free arylation reaction of 3-phenyl[1,2,3]triazolo[1,5-a]pyridine 1a with boronic acid 2 (Table 1). The optimization studies indicated that performing this reaction under 390 nm irradiation in 0.1 M benzene solution with 1.5 equiv. of boronic acid in the presence of 3 equiv. K2CO3 at room temperature allows to produce diphenyl-2-pyridylmethane 3aa in 89% yield (entry 1). Employment of other additives (entries 2–4) or solvents (entries 5–7) led to diminished yields. Only 25% of the product was formed without K2CO3 (entry 8), which points on the importance of the base for formation of the reactive triphenylboroxine arylating reagent.7a The control experiments indicated no reaction under 455 nm or 427 nm LED irradiating (entries 9 and 10) or under thermal conditions in the absence of light (entry 11).11 Expectedly, the attempts on employment of pyridotriazoles 1c and 1d, which are transparent in 390 nm region under these reaction conditions failed. Although the pyridotriazole 1d showed notable absorption in 370 nm region, the attempts of its arylation under irradiation with 370 nm LED lamp failed probably due to competing side reactions of the formed unstable carbene.
Table 1 Optimization of arylation reaction parametersa
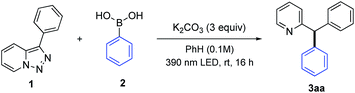
|
Entry |
Deviation from standard conditions |
Yieldb, % |
Reaction conditions: pyridotriazole 1 (0.05 mmol), boronic acids 2 (1.5 equiv.), K2CO3 (3 equiv.), benzene (0.1 M), and a 40 W 390 nm LED at room temperature.
GC/MS yields.
|
1 |
None |
89 |
2 |
Cs2CO3 instead of K2CO3 |
73 |
3 |
K3PO4 instead of K2CO3 |
44 |
4 |
NEt3 instead of K2CO3 |
40 |
5 |
PhMe instead of PhH |
84 |
6 |
THF instead of PhH |
0 |
7 |
CHCl3 instead of PhH |
24 |
8 |
Without K2CO3 |
25 |
9 |
455 nm LED instead of 390 nm LED |
0 |
10 |
427 nm LED instead of 390 nm LED |
0 |
11 |
No light, dark, 50 to 120 °C |
0 |
Intrigued by the uncovered novel reactivity of pyridotriazoles under photo-induced conditions and inspired by the importance of triarylmethanes,12 we further explored the scope of this arylation reaction. Markedly, this C–C coupling reaction showed wide scope and high functional-group tolerance on both reaction partners (Table 2a). Thus, diversely functionalized boronic acids 2 bearing electron-rich (2b–2d), electron-deficient (2h–2j), electron-neutral (2n), halogen-containing (2e–2g and 2k–2m), and sterically encumbered (2o) substituents at the ortho-, meta- and para-positions smoothly reacted with 3-phenyl[1,2,3]triazolo[1,5-a]pyridine 1a to produce diphenyl-2-pyridylmethanes 3aa–3an in good to excellent yields. In addition, the reaction of phenylboronic acid with the para-siloxy substituent worked well to give 3ab in good yield, which upon desilylation offered access to the phenol product. Notably, the reaction also efficiently proceeded with the alkenyl boronic acid 2p providing cyclohexen-1-yl product 3ap in 70% yield. Studies on the scope of pyridotriazoles showed that pyridotriazoles 1 bearing different 4-substituted phenyl or heteroaryl groups at C3 position furnished the corresponding triarylmethane products 3ea–3ia in moderate to high yields. In addition, 5-chloro pyridotriazole, 6-bromo pyridotriazole and N-fused heterocyclic pyrazinotriazole successfully underwent arylation with different arylboronic acids to give 3ja, 3ka, 2-(diphenylmethyl) pyrazine (3la, 3le and 3lf) in good yields.
Table 2 Arylation reactions of pyridotriazolesa,b
Reaction conditions: pyridotriazole 1 (0.2 mmol), boronic acids 2 (1.5 equiv.), K2CO3 (3 equiv.), benzene (0.1 M), 40 W 390 nm LED at room temperature.
Yield of isolated product.
Reaction was performed in 1 mmol scale.
Toluene (0.1 M) used as a solvent.
|
|
Next, we turned our attention to carbene X–H insertion reactions10a (Table 3). To this end, pyridotriazoles 1, under standard reaction conditions, were examined in reactions with phenols, alcohols, sulfonamides, and carboxylic acids (Table 3a). All substituted phenols tested provided the O–H insertion products 7aa–7af in moderate yields, albeit with trace to substantial amounts of the C–H insertion regioisomers.13 These reaction conditions appeared to be very general for reactions with alcohols 4g–4n. Thus, alcohols possessing various alkyl, alkenyl, thiomethyl, and even sterically hindered bicyclo groups all reacted well, providing ethers 7ag–7fm in good to high yields. Moreover, this reaction chemoselectively gave O–H insertion products with alkenols (7al and 7am), double bond moiety of which was not compromised. Likewise, aliphatic chlorine substituent was tolerated in 4n, which constitutes an additional handle for further derivatizations. In contrast to a facile O–H insertion reaction with phenols, the insertion into the N–H bond of aniline 5a was sluggish (Table 3b), which can probably be attributed to its higher pKa value.5d However, more acidic primary and secondary sulfonamides 5b–g reacted smoothly to produce the N–H insertions products 8ab–8ag in reasonable to high yields. Phthalimide 5h provided the insertion product 8ah in reasonable yield. Furthermore, both aliphatic and aromatic carboxylic acids 6a–6c were also found to be the competent substrates for the COO–H insertion reactions producing the corresponding esters 9aa–9cc in good yields (Table 3c).
Table 3 X–H insertion reactionsab
Reaction conditions: pyridotriazole 1 (0.2 mmol), X–H insertion partners 4, 5 or 6 (4 equiv.), benzene (0.1 M), 40 W 390 nm LED at room temperature.
Yield of isolated product.
dr 1 : 1.
|
|
In addition, it was also found that upon photoirradiation, the pyridotriazoles underwent efficient cyclopropanation with alkenes 10 (Table 4). The scope of pyridotriazoles was studied first. Pyridotriazoles 1 bearing different 4-substituted phenyl or heteroaryl groups at C3 position gave the corresponding cyclopropanes 11aa–11ia in moderate to high yields.14 3-Naphthalenyl pyridotriazole furnished product 11na in high yield. Furthermore, N-fused heterocyclic quinolinotriazole and 7-chloro pyridotriazole successfully underwent cyclopropanation with styrene to give 11ma and 11oa. The scope of the process with respect to the alkene components was examined next. Cyclopropanes 11ab–11ae were obtained from a diverse array of ortho-, meta-, para- and disubstituted styrenes in high yields. 2-Vinylpyridine, vinyl ether, acrylonitrile, vinyl ketone and ethyl acrylate efficiently participated in the reaction to give the products 11ag–11ak. Notably, a double bond of indole also participated in this reaction, providing fused product 11al in moderate yield.
Table 4 Cyclopropanation reactionsa
Reaction conditions: pyridotriazole 1 (0.2 mmol), styrene 10 (3 equiv.), benzene (0.1 M), 40 W 390 nm LED at room temperature.
|
|
Synthetic usefulness of this methodology was illustrated on the facile syntheses of selected bioactive molecules. Thus, desacetyl bisacodyl (DAB) 1215 and bisacodyl 13,16 which are used as stimulant laxative drugs, were efficiently obtained via the one-pot procedures from the pyridotriazole 1b with arylboronic acid 2b (Scheme 2a). Furthermore, piperidine derivative 14,17 the synthetic precursor of antihistamine agent Bepotastine, was easily accessed by the O–H insertion reaction of 7n with pyridotriazole 1f (Scheme 2b).
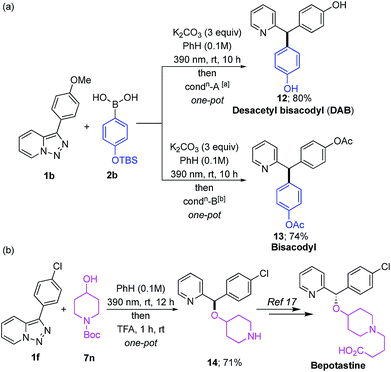 |
| Scheme 2 Synthesis of biologically active molecules. (a) Conditions A: HBr/AcOH = 1 : 1, reflux, overnight. (b) Conditions B: HBr/AcOH = 1 : 1, reflux, overnight. Then Et3N (3 equiv.), Ac2O (4 equiv.), DCM (0.1 M), rt, overnight. | |
Conclusions
We developed general and efficient arylation, X–H insertions, and cyclopropanation reactions of pyridotriazoles. This transition metal-free light-induced18 protocol, operating under mild conditions, exhibits wide functional-group tolerance efficiently producing valuable triarylmethanes and heteroatom-substituted benzylpyridine derivatives.
Conflicts of interest
There are no conflicts to declare.
Acknowledgements
We thank the National Institutes of Health (GM120281) and National Science Foundation (CHE-1663779) for the financial support of this work.
Notes and references
- For reviews on reactivity of pyridotriazoles, see:
(a) B. Chattopadhyay and V. Gevorgyan, Angew. Chem., Int. Ed., 2012, 51, 862–872 (
Angew. Chem.
, 2012
, 124
, 886–896
) CrossRef CAS PubMed;
(b) P. Anbarasan, D. Yadagiri and S. Rajasekar, Synthesis, 2014, 46, 3004–3023 CrossRef CAS.
- For reviews on reactivity of N-sulfonyl triazoles, see:
(a) A. V. Gulevich and V. Gevorgyan, Angew. Chem., Int. Ed., 2013, 52, 1371–1373 (
Angew. Chem.
, 2013
, 125
, 1411–1413
) CrossRef CAS PubMed;
(b) H. M. L. Davies and J. S. Alford, Chem. Soc. Rev., 2014, 43, 5151–5162 RSC;
(c) Y. Jiang, R. Sun, X. Tang and M. Shi, Chem.–Eur. J., 2016, 22, 17910–17924 CrossRef CAS PubMed.
- For the first report on transannulation of pyridotriazoles, see:
(a) S. Chuprakov, F. Hwang and V. Gevorgyan, Angew. Chem., Int. Ed., 2007, 46, 4757–4759 (
Angew. Chem.
, 2007
, 119
, 4841–4843
) CrossRef CAS PubMed; see also:
(b) S. Chuprakov and V. Gevorgyan, Org. Lett., 2007, 9, 4463–4466 CrossRef CAS PubMed;
(c) Y. Shi, A. V. Gulevich and V. Gevorgyan, Angew. Chem., Int. Ed., 2014, 53, 14191–14195 CrossRef CAS PubMed;
(d) V. Helan, A. V. Gulevich and V. Gevorgyan, Chem. Sci., 2015, 6, 1928–1931 RSC;
(e) Y. Shi and V. Gevorgyan, Chem. Commun., 2015, 51, 17166–17169 RSC;
(f) J. H. Kim, T. Gensch, D. Zhao, L. Stegemann, C. A. Strassert and F. Glorius, Angew. Chem., Int. Ed., 2015, 54, 10975–10979 CrossRef CAS PubMed;
(g) W. H. Jeon, J. Y. Son, J. E. Kim and P. H. Lee, Org. Lett., 2016, 18, 3498–3501 CrossRef CAS PubMed;
(h) A. Joshi, D. C. Mohan and S. Adimurthy, J. Org. Chem., 2016, 81, 9461–9469 CrossRef CAS PubMed;
(i) A. Joshi, D. C. Mohan and S. Adimurthy, Org. Lett., 2016, 18, 464–467 CrossRef CAS PubMed;
(j) R. Adam, S. Alom, B. Abarca and R. Ballesteros, Tetrahedron, 2016, 72, 8436–8441 CrossRef CAS;
(k) H. Kim, S. Kim, J. Kim, J.-Y. Son, K. Um and P. H. Lee, Org. Lett., 2017, 19, 5677–5680 CrossRef CAS PubMed;
(l) S. Roy, S. K. Das and B. Chattopadhyay, Angew. Chem., Int. Ed., 2018, 57, 2238–2243 CrossRef CAS PubMed.
- For selected reports on transannulation of N-sulfonyl triazoles, see:
(a) T. Horneff, S. Chuprakov, N. Chernyak, V. Gevorgyan and V. V. Fokin, J. Am. Chem. Soc., 2008, 130, 14972–14974 CrossRef CAS PubMed;
(b) T. Miura, M. Yamauchi and M. Murakami, Chem. Commun., 2009, 1470–1471 RSC;
(c) B. Chattopadhyay and V. Gevorgyan, Org. Lett., 2011, 13, 3746–3749 CrossRef CAS PubMed;
(d) B. T. Parr, S. A. Green and H. M. L. Davies, J. Am. Chem. Soc., 2013, 135, 4716–4718 CrossRef CAS PubMed;
(e) J. E. Spangler and H. M. L. Davies, J. Am. Chem. Soc., 2013, 135, 6802–6805 CrossRef CAS PubMed;
(f) J. S. Alford, J. E. Spangler and H. M. L. Davies, J. Am. Chem. Soc., 2013, 135, 11712–11715 CrossRef CAS PubMed;
(g) B. T. Parr and H. M. L. Davies, Angew. Chem., Int. Ed., 2013, 52, 10044–10047 (
Angew. Chem.
, 2013
, 125
, 10228–10231
) CrossRef CAS PubMed;
(h) M. Zibinsky and V. V. Fokin, Angew. Chem., Int. Ed., 2013, 52, 1507–1510 (
Angew. Chem.
, 2013
, 125
, 1547–1550
) CrossRef CAS PubMed;
(i) S. Chuprakov, S. W. Kwok and V. V. Fokin, J. Am. Chem. Soc., 2013, 135, 4652–4655 CrossRef CAS PubMed;
(j) T. Miura, T. Tanaka, K. Hiraga, S. G. Stewart and M. Murakami, J. Am. Chem. Soc., 2013, 135, 13652–13655 CrossRef CAS PubMed;
(k) T. Miura, K. Hiraga, T. Biyajima, T. Nakamuro and M. Murakami, Org. Lett., 2013, 15, 3298–3301 CrossRef CAS PubMed;
(l) E. E. Schultz and R. Sarpong, J. Am. Chem. Soc., 2013, 135, 4696–4699 CrossRef CAS PubMed;
(m) Y. Shi and V. Gevorgyan, Org. Lett., 2013, 15, 5394–5396 CrossRef CAS PubMed;
(n) T. Miura, Y. Funakoshi and M. Murakami, J. Am. Chem. Soc., 2014, 136, 2272–2275 CrossRef CAS PubMed;
(o) C. Kim, S. Park, D. Eom, B. Seo and P. H. Lee, Org. Lett., 2014, 16, 1900–1903 CrossRef CAS PubMed;
(p) J. Yang, C. Zhu, X. Tang and M. Shi, Angew. Chem., Int. Ed., 2014, 53, 5142–5146 (
Angew. Chem.
, 2014
, 126
, 5242–5246
) CAS;
(q) H. Shang, Y. Wang, Y. Tian, J. Feng and Y. Tang, Angew. Chem., Int. Ed., 2014, 53, 5662–5666 CrossRef CAS PubMed;
(r) K. Chen, Z.-Z. Zhu, Y.-S. Zhang, X.-Y. Tang and M. Shi, Angew. Chem., Int. Ed., 2014, 53, 6645–6649 CrossRef CAS PubMed;
(s) F. Medina, C. Besnard and J. Lacour, Org. Lett., 2014, 16, 3232–3235 CrossRef CAS PubMed;
(t) V. N. G. Lindsay, H. M.-F. Viart and R. Sarpong, J. Am. Chem. Soc., 2015, 137, 8368–8371 CrossRef CAS PubMed;
(u) W. Cheng, Y. Tang, Z. Xu and C. Li, Org. Lett., 2016, 18, 6168–6171 CrossRef CAS PubMed;
(v) Y. Li, R. Zhang, A. Ali, J. Zhang, X. Bi and J. Fu, Org. Lett., 2017, 19, 3087–3090 CrossRef CAS PubMed;
(w) L. Fu, H. M. L. Davis and R. Sarpong, Org. Lett., 2017, 19, 1504–1507 CrossRef CAS PubMed;
(x) W. Chen, Y. Bai, Y. Luo and P. Xu, Org. Lett., 2017, 19, 364–367 CrossRef CAS PubMed;
(y) D. Yadagiri, M. Chaitanya, A. C. S. Reddy and P. Anbarasan, Org. Lett., 2018, 20, 3762–3765 CrossRef CAS PubMed.
- For selected reports on X–H insertions of N-sulfonyl triazoles, see:
(a) S. Chuprakov, J. A. Malik, M. Zibinsky and V. V. Fokin, J. Am. Chem. Soc., 2011, 133, 10352–10355 CrossRef CAS PubMed;
(b) T. Miura, T. Biyajima, T. Fujii and M. Murakami, J. Am. Chem. Soc., 2012, 134, 194–196 CrossRef CAS PubMed;
(c) T. Miura, T. Tanaka, T. Biyajima, A. Yada and M. Murakami, Angew. Chem., Int. Ed., 2013, 52, 3883–3886 (
Angew. Chem.
, 2013
, 125
, 3975–3978
) CrossRef CAS PubMed;
(d) S. Chuprakov, B. T. Worrell, N. Selander, R. K. Sit and V. V. Fokin, J. Am. Chem. Soc., 2014, 136, 195–202 CrossRef CAS PubMed;
(e) T. Miura, Q. Zhao and M. Murakami, Angew. Chem., Int. Ed., 2017, 56, 16645–16649 CrossRef CAS PubMed.
- For selected reports on cyclopropanation of N-sulfonyl triazoles, see:
(a) S. Chuprakov, S. W. Kwok, L. Zhang, L. Lercher and V. V. Fokin, J. Am. Chem. Soc., 2009, 131, 18034–18035 CrossRef CAS PubMed;
(b) N. Grimster, L. Zhang and V. V. Fokin, J. Am. Chem. Soc., 2010, 132, 2510–2511 CrossRef CAS PubMed;
(c) J. S. Alford and H. M. L. Davies, Org. Lett., 2012, 14, 6020–6023 CrossRef CAS PubMed;
(d) J. C. Culhane and V. V. Fokin, Org. Lett., 2011, 13, 4578–4580 CrossRef CAS PubMed.
- For selected transformations on N-sulfonyl triazoles, see:
(a) N. Selander, B. T. Worrell, S. Chuprakov, S. Velaparthi and V. V. Fokin, J. Am. Chem. Soc., 2012, 134, 14670–14673 CrossRef CAS PubMed;
(b) D. Yadagiri, A. C. S. Reddy and P. Anbarasan, Chem. Sci., 2016, 7, 5934–5938 RSC;
(c) A. Bosmani, A. Guarnieri-Ibáñez, S. Goudedranche, C. Besnard and J. Lacour, Angew. Chem., Int. Ed., 2018, 57, 7151–7155 CrossRef CAS PubMed.
- M. Ratushnyy, M. Kamenova and V. Gevorgyan, Chem. Sci., 2018, 9, 7193–7197 RSC.
- Harsh UVC irradiation by Hg-Xe lamp combined with monochromators or fillers was reported
(a) M. Kuzaj, H. Lüerssen and C. Wentrup, Angew. Chem., Int. Ed., 1986, 25, 480–482 CrossRef;
(b) K. Komatsu, N. Ichikawa and H. Tomioka, J. Am. Chem. Soc., 1993, 115, 8621–8626 CrossRef;
(c) A. Ignatchenko and H. E. Zimmerman, J. Org. Chem., 1999, 64, 6635–6645 CrossRef PubMed;
(d) A. Kuhn, C. Plüg and C. Wentrup, J. Am. Chem. Soc., 2000, 122, 1945–1948 CrossRef CAS;
(e) C. Plüg, A. Kuhn and C. Wentrup, J. Chem. Soc., Perkin Trans. 1, 2002, 1366–1368 RSC;
(f) H. G. Aderson, P. Bednarek and C. Wentrup, J. Phys. Org. Chem., 2003, 16, 519–524 CrossRef;
(g) N. Fu, A. D. Allen, S. Kobayashi, P. A. Sequeira, M. Shang, T. T. Tidewell and M. Mishima, J. Am. Chem. Soc., 2007, 129, 6210–6215 CrossRef CAS PubMed. For recent report on photo-redox transformation of benzotriazoles, see:
(h) M. Teders, A. Gómez-Suárez, L. Pitzer, M. N. Hopkinson and F. Glorius, Angew. Chem., Int. Ed., 2017, 56, 902–906 CrossRef CAS PubMed;
(i) M. Teders, L. Pitzer, S. Buss and F. Glorius, ACS Catal., 2017, 7, 4053–4056 CrossRef CAS.
-
(a) I. D. Jurberg and H. M. L. Davies, Chem. Sci., 2018, 9, 5112–5118 RSC;
(b) T. Xiao, M. Mei, Y. He and L. Zhou, Chem. Commun., 2018, 54, 8865–8868 RSC;
(c) R. Hommelsheim, Y. Guo, Z. Yang, C. Empel and R. M. Koenigs, Angew. Chem., Int. Ed., 2019, 58, 1203–1207 CrossRef CAS PubMed;
(d) Ł. W. Ciszewski, K. Rybicka-Jasińska and D. Gryko, Org. Biomol. Chem., 2019, 17, 432–448 RSC;
(e) F. He and R. M. Koenigs, Chem. Commun., 2019, 55, 4881–4884 RSC;
(f) J. Yang, J. Wang, H. Huang, G. Qin, Y. Jiang and T. Xiao, Org. Lett., 2019, 21, 2654–2657 CrossRef CAS PubMed;
(g)
S. Jana and R. M. Koenigs, Asian J. Org. Chem., 2019, 8, 683–686 Search PubMed;
(h)
Z. Yang, Y. Guo and R. M. Koenigs, Chem.–Eur. J., 2019, 25, 6703 Search PubMed;
(i) X. Zhang, C. Du, H. Zhang, X. Li, Y. Wang, J. Niu and M. Song, Synthesis, 2019, 51, 889–898 CrossRef CAS.
- During manuscript preparation, a related work on thermal arylation of mostly activated pyridotriazoles has been reported, see: C. Dong, X. Wang, Z. Pei and R. Shen, Org. Lett., 2019, 21, 4148–4152 CrossRef CAS PubMed.
- For application and importance of triarylmethanes, see:
(a) M. Irie, J. Am. Chem. Soc., 1983, 105, 2078–2079 CrossRef CAS;
(b) D. Duxbury, Chem. Rev., 1993, 93, 381–433 CrossRef CAS;
(c) M. S. Shchepinov, Chem. Soc. Rev., 2003, 32, 170–180 RSC;
(d) R. S. Dothager, K. S. Putt, B. J. Allen, B. J. Leslie, V. Nesterenko and P. J. Hergenrother, J. Am. Chem. Soc., 2005, 127, 8686–8696 CrossRef CAS PubMed;
(e) V. Nair, S. Thomas, S. C. Mathew and K. G. Abhilash, Tetrahedron, 2006, 62, 6731–6747 CrossRef CAS;
(f) A. C. Bhasikuttan, J. Mohanty, W. M. Nau and H. Pal, Angew. Chem., Int. Ed., 2007, 46, 4120–4122 CrossRef CAS PubMed;
(g) S. K. Das, G. Panda, V. Chaturvedi, Y. S. Manju, A. K. Gaikwad and S. Sinha, Bioorg. Med. Chem. Lett., 2007, 17, 5586–5589 CrossRef CAS PubMed;
(h) H. Abe, J. Wang, K. Furukawa, K. Oki, M. Uda, S. Tsuneda and Y. Ito, Bioconjugate Chem., 2008, 19, 1219–1226 CrossRef CAS PubMed;
(i) H. N. Kim, M. H. Lee, H. J. Kim, J. S. Kim and J. Yoon, Chem. Soc. Rev., 2008, 37, 1465–1472 RSC;
(j) M. Beija, C. A. M. Afonso and M. G. Martinho, Chem. Soc. Rev., 2009, 38, 2410–2433 RSC. For the synthesis of triarylmethanes, selected examples:
(k) T. Niwa, H. Yorimitsu and K. Oshima, Org. Lett., 2007, 9, 2373–2375 CrossRef CAS PubMed;
(l) Y. Xia, F. Hu, Z. Liu, P. Qu, R. Ge, C. Ma, Y. Zhang and J. Wang, Org. Lett., 2013, 15, 1784–1787 CrossRef CAS PubMed;
(m) M. Nambo and C. M. Crudden, Angew. Chem., Int. Ed., 2014, 53, 742–746 CrossRef CAS PubMed;
(n) X. Cao, S. Sha, B. Kim, C. Morgan, R. Huang, C. Yang and P. J. Walsh, Chem. Sci., 2016, 7, 611–618 RSC.
- For nucleophilic addition of electron-rich arenes to rhodium carbenoids, see:
(a) D. Yadagiri and P. Anbarasan, Org. Lett., 2014, 16, 2510–2513 CrossRef CAS PubMed;
(b) S. Shin, Y. Park, C. Kim, J. Son and P. Lee, J. Org. Chem., 2015, 80, 5859–5869 CrossRef CAS PubMed . We were unsuccessful under optimized condtion with different electron rich arenes using stoichiometric amount of solvent.
- Employment of methyl-substituted pyridotriazole 1d under 370 nm irradiation produced trace amounts of the product.
- T. Fujita, S. Karaki, T. Tateika and A. Kuwahara, Biomed. Res., 2016, 37, 13–20 CrossRef CAS PubMed.
-
(a) R. D. Kacere, S. S. Srivatsa, W. J. Tremaine, L. E. Ebnet and K. P. Batts, Mayo Clin. Proc., 1993, 68, 355–357 CrossRef CAS PubMed;
(b) K. Kudo, C. Miyazaki, R. Kadoya, T. Imamura, N. Jitsufuchi and N. Ikeda, J. Anal. Toxicol., 1998, 22, 274–278 CrossRef CAS PubMed;
(c) S. D. Wexner, T. Force, D. E. Beck, T. H. Baron, R. D. Fanelli, N. Hyman, B. Shen and K. E. Wasco, Gastrointestinal Endoscopy, 2006, 63, 894–909 CrossRef PubMed.
- T. H. Ha, K. H. Suh and G. S. Lee, Bull. Korean Chem. Soc., 2013, 34, 549–553 CrossRef CAS.
- Test experiments indicated that analogously to the arylation reaction (Table 1, entry 11), no X–H insertion or cyclopropanation reactions occur under dark conditions.
Footnotes |
† Electronic supplementary information (ESI) available. See DOI: 10.1039/c9sc02448d |
‡ These authors contributed equally to this work. |
|
This journal is © The Royal Society of Chemistry 2019 |
Click here to see how this site uses Cookies. View our privacy policy here.