DOI:
10.1039/D1SC05811H
(Edge Article)
Chem. Sci., 2021,
12, 15765-15769
Three-component 1,2-carboamination of vinyl boronic esters via amidyl radical induced 1,2-migration†
Received
21st October 2021
, Accepted 16th November 2021
First published on 16th November 2021
Abstract
Three-component 1,2-carboamination of vinyl boronic esters with alkyl/aryl lithium reagents and N-chloro-carbamates/carboxamides is presented. Vinylboron ate complexes generated in situ from the boronic ester and an organo lithium reagent are shown to react with readily available N-chloro-carbamates/carboxamides to give valuable 1,2-aminoboronic esters. These cascades proceed in the absence of any catalyst upon simple visible light irradiation. Amidyl radicals add to the vinylboron ate complexes followed by oxidation and 1,2-alkyl/aryl migration from boron to carbon to give the corresponding carboamination products. These practical cascades show high functional group tolerance and accordingly exhibit broad substrate scope. Gram-scale reaction and diverse follow-up transformations convincingly demonstrate the synthetic potential of this method.
Alkenes are important and versatile building blocks in organic synthesis. 1,2-Difunctionalization of alkenes offers a highly valuable synthetic strategy to access 1,2-difunctionalized alkanes by sequentially forming two vicinal σ-bonds.1a–h Among these vicinal difunctionalizations, the 1,2-carboamination of alkenes, in which a C–N and a C–C bond are formed, provides an attractive route for the straightforward preparation of structurally diverse amine derivatives (Scheme 1a).2a–c Along these lines, transition-metal-catalyzed or radical 1,2-carboaminations of activated and unactivated alkenes have been reported.3a–p However, the 1,2-carboamination of vinylboron reagents, a privileged class of olefins,4a–h to form valuable 1,2-aminoboron compounds which can be readily used in diverse downstream functionalizations,5a–c,6a–d has been rarely investigated. To the best of our knowledge, there are only two reported examples, as shown in Schemes 1b and c. In 2013, Molander disclosed a Rh-catalyzed 1,2-aminoarylation of potassium vinyltrifluoroborate with benzhydroxamates via C–H activation (Scheme 1b).7 Thus, the 1,2-carboamination of vinylboron reagents is still underexplored but highly desirable.
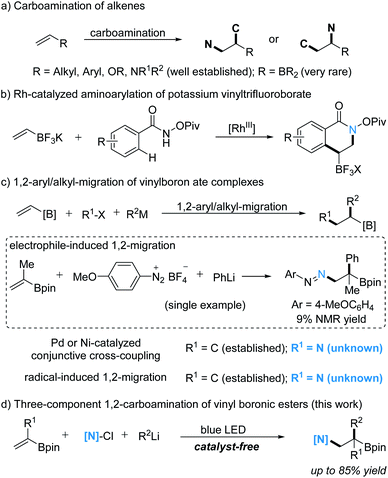 |
| Scheme 1 Intermolecular 1,2-carboamination of alkenes. | |
1,2-Alkyl/aryl migrations induced by β-addition to vinylboron ate complexes have been shown to be highly reliable for 1,2-difunctionalization of vinylboron reagents (Scheme 1c).4d–h In 1967, Zweifel's group developed 1,2-alkyl/aryl migrations of vinylboron ate complexes induced by an electrophilic halogenation.8 In 2016, the Morken group reported the electrophilic palladation-induced 1,2-alkyl/aryl migration of vinylboron ate complexes.9a–k Shortly thereafter, we,10a–c Aggarwal,11a–c and Renaud12 developed alkyl radical induced 1,2-alkyl/aryl migrations of vinylboron ate complexes. In these recent examples, the migration is induced by a C-based radical/electrophile, halogen and chalcogen electrophiles.13a,b
In contrast, N-reagent-induced migration of vinylboron ate complexes proceeding via β-amination is not well investigated. To our knowledge, as the only example the Aggarwal laboratory described the reaction of a vinylboron ate complex with an aryldiazonium salt as the electrophile, but the desired β-aminated rearrangement product was formed in only 9% NMR yield (Scheme 1c).13a No doubt, β-amino alkylboronic esters would be valuable intermediates in organic synthesis. Encouraged by our continuous work on amidyl radicals14a–i and 1,2-migrations of boron ate complexes,10a–c,15a–f we therefore decided to study the amidyl radical-induced carboamination of vinyl boronic esters for the preparation of 1,2-aminoboronic esters. N-chloroamides were chosen as N-radical precursors,16a–c as these N-chloro compounds can be easily prepared from the corresponding N–H analogues.17 Herein, we present a catalyst-free three-component 1,2-carboamination of vinyl boronic esters with N-chloroamides and readily available alkyl/aryl lithium reagents (Scheme 1d).
We commenced our study by exploring the reaction of the vinylboron ate complex 2a with tert-butyl chloro(methyl)carbamate 3a applying photoredox catalysis. Complex 2a was generated in situ by addition of n-butyllithium to the boronic ester 1a in diethyl ether at 0 °C. After solvent removal, the photocatalyst fac-Ir(ppy)3 (1 mol%) and THF were added followed by the addition of 3a. Upon blue LED light irradiation, the mixture was stirred at room temperature for 16 hours. To our delight, the desired 1,2-aminoboronic ester 4a was obtained, albeit with low yield (26%, Table 1, entry 1). Solvent screening revealed acetonitrile to be superior to all other solvents tested, and a 56% yield was achieved (Table 1, entries 1–3). With Ru(bpy)3Cl2·6H2O in place of fac-Ir(ppy)3, yield of 4a increased to 69% (Table 1, entry 4). The use of the photocatalyst Na2Eosin Y gave a similar result (Table 1, entry 5). Decreasing the concentration led to an improvement of the yield with Na2Eosin Y as the photocatalyst (70%, Table 1, entry 6). Surprisingly, a control experiment run in the absence of any photocatalyst under irradiation with blue LED afforded the aminoboronic ester 4a in 45% yield (Table 1, entry 7). Upon lowering the temperature, the yield of 4a under such catalyst-free conditions further improved, and the best result was obtained when the reaction was conducted at −20 °C (85% yield of isolated product; Table 1, entries 8 and 9). Very little product was formed upon conducting the reaction in the dark, which showed that light was essential (Table 1, entry 10).
Table 1 Reaction optimizationa
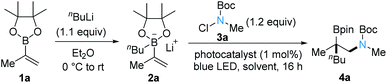
|
Entry |
Photocatalyst |
Solvent |
T (°C) |
Yieldb (%) |
Reaction conditions: 1a (0.20 mmol), nBuLi (0.22 mmol), in Et2O (2 mL), 0 °C to rt, 1 h, under Ar. After vinylboron ate complex formation, solvent exchange to the selected solvent (2 mL) was performed.
GC yield using n-C14H30 as an internal standard; yield of isolated product is given in parentheses.
4 mL MeCN was used.
Reaction carried out in the dark.
|
1 |
fac-Ir(ppy)3 |
THF |
rt |
26 |
2 |
fac-Ir(ppy)3 |
DMSO |
rt |
2 |
3 |
fac-Ir(ppy)3 |
MeCN |
rt |
56 |
4 |
Ru(bpy)3Cl2·6H2O |
MeCN |
rt |
69 |
5 |
Na2Eosin Y |
MeCN |
rt |
69 |
6c |
Na2Eosin Y |
MeCN |
rt |
70 |
7c |
None |
MeCN |
rt |
45 |
8c |
None |
MeCN |
0 |
78 |
9c |
None |
MeCN |
−20 |
88 (85) |
10c,d |
None |
MeCN |
−20 |
2 |
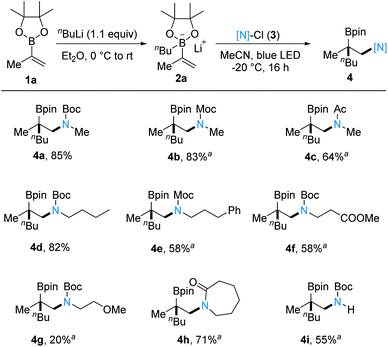 |
| Scheme 2 1,2-Carboamination of 1a with various amidyl radical precursors. Reaction conditions: 1a (0.20 mmol, 1.0 equiv.), nBuLi (0.22 mmol, 1.1 equiv.), in Et2O (2 mL), 0 °C to rt, 1 h, under Ar; then [N]-Cl (0.24 mmol, 1.2 equiv.), −20 °C, 16 h, in MeCN (4 mL). Yields given correspond to yields of isolated products. aA solution of [N]-Cl (0.30 mmol, 1.5 equiv.) in MeCN (1 mL) was used. See the ESI† for experimental details. | |
With optimal conditions in hand, we then investigated the scope of this new 1,2-carboamination protocol keeping 2a as the N-radical acceptor (Scheme 2). This transformation turned out to be compatible with various primary amine reaction partners bearing carbamate (4a, 4b and 4d–4g) or acyl protecting groups (4c) (20–85%). Notably, N-chlorolactams can be used as N-radical precursors, as shown by the successful preparation of 4h (71%). Moreover, Boc-protected ammonia was also tolerated, delivering 4i in an acceptable yield (55%).
We continued the studies by testing a range of vinylboron ate complexes (Scheme 3). To this end, various vinylboron ate complexes were generated by reacting the vinyl boronic ester 1a with methyllithium, n-hexyllithium, isopropyllithium and tert-butyllithium. For the n-alkyl-substituted vinylboron ate complexes, the 1,2-carboamination worked smoothly to afford 4j and 4k in good yields. However, the vinylboron ate complex derived from isopropyllithium addition provided the desired products in much lower yield (4l, 18% yield). When tert-butyllithium was employed, only a trace of the targeted product was detected (see ESI†). As expected, cascades comprising a 1,2-aryl migration from boron to carbon worked well. Thus, by using PhLi for vinylboron ate complex formation, the 1,2-aminoboronic esters 4m–4o were obtained in 69–73% yields with the Boc (t-BuOCONClMe), ethoxycarbonyl-(EtOCONClMe) and methoxycarbonyl (Moc)-(MeOCONClMe) protected N-chloromethylamines (for the structures of 3, see ESI†) as radical amination reagents. Keeping 3b as the N-donor, other aryllithiums bearing various functional groups at the para position of the aryl moiety, such as methoxy (4p), trimethylsilyl (4q), methyl (4r), phenyl (4s), trifluoromethoxy (4t), trifluoromethyl (4u), and halides (4v–4x) all reacted well in this transformation. Aryl groups bearing meta substituents are also tolerated, as documented by the preparation of 4y (81%). To our delight, a boron ate complex generated with a 3-pyridyl lithium reagent engaged in the cascade and the carboamination product 4z was isolated in high yield (82%).
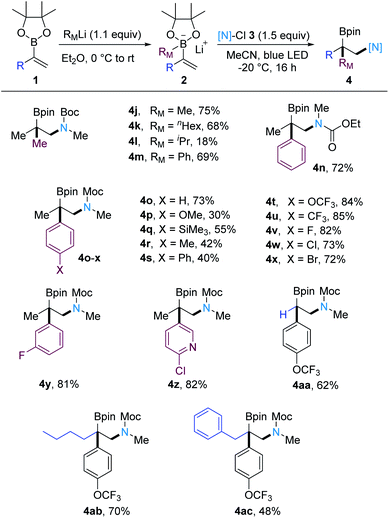 |
| Scheme 3 Scope of vinylboron ate complexes. Reaction conditions: 1 (0.20 mmol, 1.0 equiv.), RMLi (0.22 mmol, 1.1 or 1.3 equiv.), Et2O or THF, under Ar; then [N]-Cl (0.30 mmol, 1.5 equiv.), −20 °C, 16 h, in MeCN. Yields given correspond to yields for isolated products. See the ESI† for experimental details. | |
The reason for the dramatic reduction in yield when α-branched alkyllithium or electron-rich aryllithium reagents were used might be that the corresponding vinylboron ate complexes could be oxidized by N-chloroamides via a single-electron oxidation process.18a–e Furthermore, the α-unsubstituted vinyl boronic ester and vinyl boronic ester bearing various α-substituents are suitable N-radical acceptors and the corresponding products 4aa–4ac were obtained in 48–70% yield.
To gain insights into the mechanism of this 1,2-carboamination, a control experiment was conducted. The reaction could be nearly fully suppressed when the reaction was carried out in the presence of a typical radical scavenger (2,2-6,6-tetramethyl piperidine-N-oxyl, TEMPO), indicating a radical mechanism (Scheme 4a). Further, considering an ionic process, the N-chloroamides would react as Cl+-donors that would lead to Zweifel-type products, which were not observed under the applied conditions. The proposed mechanism is shown in Scheme 4b. As chloroamides have been recently proposed to undergo homolysis under visible light irradiation,19a,b we propose that initiation proceeds via homolytic N–Cl cleavage generating the electrophilic amidyl radical A, which then adds to the electron-rich vinylboron ate complex 2a to give the adduct boronate radical B. The radical anion B then undergoes single electron transfer (SET) oxidation with 3a in an electron-catalyzed process20a,b or chloride atom transfer with 3a to provide C or D along with the amidyl radical A, thereby sustaining the radical chain. Intermediates C or D can then react via a boronate 1,2-migration10c,11c,21 to eventually give the isolated product 4a.
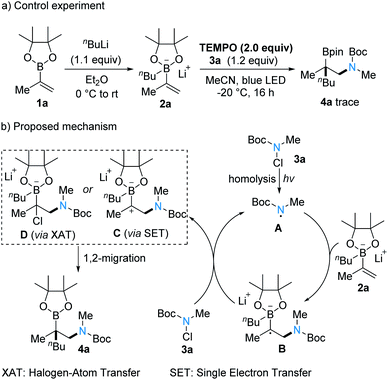 |
| Scheme 4 Control experiment and proposed mechanism. | |
To document the synthetic utility of the method, a larger-scale reaction and various follow-up transformations were conducted. Gram-scale reaction of 2a with 3a afforded the desired product 4a in good yield, demonstrating the practicality of this transformation (Scheme 5a). Oxidation of 4a with NaBO3 provided the β-amino alcohol 5 in 89% yield (Scheme 5b). The N-Boc homoallylic amine 6 was obtained by Zweifel-olefination with a commercially available vinyl Grignard reagent and elemental iodine in good yield (79%).22 Heteroarylation of the C–B bond in 4a was realized by oxidative coupling of 4a with 2-thienyl lithium to provide 7.23
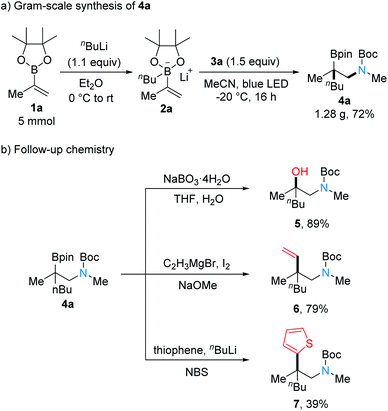 |
| Scheme 5 Gram-scale reaction and follow-up chemistry. | |
In summary, we have described an efficient method for the preparation of 1,2-aminoboronic esters from vinyl boronic esters via catalyst-free three-component radical 1,2-carboamination. Readily available N-chloro-carbamates/carboxamides, which are used as the N-radical precursors, react efficiently with in situ generated vinylboron ate complexes to afford the corresponding valuable 1,2-aminoboronic esters in good yields. The reaction features broad substrate scope and high functional group tolerance. The value of the introduced method was documented by Gram-scale reaction and successful follow-up transformations.
Data availability
The data that support the findings of this study are available in the ESI† or on request from the corresponding author.
Author contributions
C. Y. conducted all experiments and characterized the novel compounds. C. Y and A. S. designed the experiments and wrote the manuscript.
Conflicts of interest
The authors declare no conflict of interest.
Acknowledgements
We thank the European Research Council (ERC Advanced Grant Agreement No. 692640) for financial support.
Notes and references
- For selected reviews, see:
(a) Z.-L. Li, G.-C. Fang, Q.-S. Gu and X.-Y. Liu, Chem. Soc. Rev., 2020, 49, 32 RSC;
(b) R. K. Dhungana, S. Kc, P. Basnet and R. Giri, Chem. Rec., 2018, 18, 1314 CrossRef CAS PubMed;
(c) J.-S. Zhang, L. Liu, T. Chen and L.-B. Han, Chem.–Asian J., 2018, 13, 2277 CrossRef CAS PubMed;
(d) X.-W. Lan, N.-X. Wang and Y. Xing, Eur. J. Org. Chem., 2017, 5821 CrossRef CAS;
(e) M. P. Plesniak, H.-M. Huang and D. J. Procter, Nat. Rev. Chem., 2017, 1, 0077 CrossRef;
(f) E. Godineau and Y. Landais, Chem.–Eur. J., 2009, 15, 3044 CrossRef CAS PubMed;
(g) T. Pintauer and K. Matyjaszewski, Chem. Soc. Rev., 2008, 37, 1087 RSC;
(h) J. Iqbal, B. Bhatia and N. K. Nayyar, Chem. Rev., 1994, 94, 519 CrossRef CAS.
- For selected reviews on carboamination of alkenes, see:
(a) X. Chen, F. Xiao and W.-M. He, Org. Chem. Front., 2021, 8, 5206 RSC;
(b) H. Jiang and A. Studer, Chem. Soc. Rev., 2020, 49, 1790 RSC;
(c) S. Z. Zard, Chem. Soc. Rev., 2008, 37, 1603 RSC.
- For selected examples of three-component carboamination of alkenes, see:
(a) T. Kang, N. Kim, P. T. Cheng, H. Zhang, K. Foo and K. M. Engle, J. Am. Chem. Soc., 2021, 143, 13962 CrossRef CAS PubMed;
(b) S. Lee and T. Rovis, ACS Catal., 2021, 11, 8585 CrossRef CAS PubMed;
(c) S. N. Gockel, S. Lee, B. L. Gay and K. L. Hull, ACS Catal., 2021, 11, 5166 CrossRef CAS;
(d) L. Ge, H. Zhou, M.-F. Chiou, H. Jiang, W. Jian, C. Ye, X. Li, X. Zhu, H. Xiong, Y. Li, L. Song, X. Zhang and H. Bao, Nat. Catal., 2021, 4, 28 CrossRef CAS;
(e) J. J. Kennedy-Ellis, E. D. Boldt and S. R. Chemler, Org. Lett., 2020, 22, 8365 CrossRef CAS PubMed;
(f) T. Pinkert, T. Wegner, S. Mondal and F. Glorius, Angew. Chem., Int. Ed., 2019, 58, 15041 CrossRef CAS PubMed;
(g) V. A. van der Puyl, J. Derosa and K. M. Engle, ACS Catal., 2019, 9, 224 CrossRef CAS;
(h) X. Bao, T. Yokoe, T. M. Ha, Q. Wang and J. Zhu, Nat. Commun., 2018, 9, 3725 CrossRef PubMed;
(i) Y.-Y. Liu, X.-H. Yang, R.-J. Song, S. Luo and J.-H. Li, Nat. Commun., 2017, 8, 14720 CrossRef PubMed;
(j) B. Qian, S. Chen, T. Wang, X. Zhang and H. Bao, J. Am. Chem. Soc., 2017, 139, 13076 CrossRef CAS PubMed;
(k) D. Wang, L. Wu, F. Wang, X. Wan, P. Chen, Z. Lin and G. Liu, J. Am. Chem. Soc., 2017, 139, 6811 CrossRef CAS PubMed;
(l) F. Wang, X. Qi, Z. Liang, P. Chen and G. Liu, Angew. Chem., Int. Ed., 2014, 53, 1881 CrossRef CAS PubMed;
(m) D. Prasad Hari, T. Hering and B. König, Angew. Chem., Int. Ed., 2014, 53, 725 CrossRef CAS PubMed;
(n) G. Fumagalli, S. Boyd and M. F. Greaney, Org. Lett., 2013, 15, 4398 CrossRef CAS PubMed;
(o) H. Zhang, W. Pu, T. Xiong, Y. Li, X. Zhou, K. Sun, Q. Liu and Q. Zhang, Angew. Chem., Int. Ed., 2013, 52, 2529 CrossRef CAS PubMed;
(p) Y. Yasu, T. Koike and M. Akita, Org. Lett., 2013, 15, 2136 CrossRef CAS PubMed.
- For selected reviews on transformations of vinylboron reagents, see:
(a) N. Miyaura and A. Suzuki, Chem. Rev., 1995, 95, 2457 CrossRef CAS;
(b) A. Suzuki, Angew. Chem., Int. Ed., 2011, 50, 6722 CrossRef CAS PubMed;
(c) T. Hayashi and K. Yamasaki, Chem. Rev., 2003, 103, 2829 CrossRef CAS PubMed;
(d) N. Kumar, R. R. Reddy, N. Eghbarieh and A. Masarwa, Chem. Commun., 2020, 56, 13 RSC;
(e) N. D. C. Tappin and P. Renaud, Chimia, 2020, 74, 33 CrossRef CAS PubMed;
(f) M. Kischkewitz, F. W. Friese and A. Studer, Adv. Synth. Catal., 2020, 362, 2077 CrossRef PubMed;
(g) S. Namirembe and J. P. Morken, Chem. Soc. Rev., 2019, 48, 3464 RSC;
(h) R. J. Armstrong and V. K. Aggarwal, Synthesis, 2017, 49, 3323 CrossRef CAS.
-
(a) J. W. B. Fyfe and A. J. B. Watson, Chem, 2017, 3, 31 CrossRef CAS;
(b) S. Darses and J.-P. Genet, Chem. Rev., 2008, 108, 288 CrossRef CAS PubMed;
(c) R. Larouche-Gauthier, T. G. Elford and V. K. Aggarwal, J. Am. Chem. Soc., 2011, 133, 16794 CrossRef CAS PubMed.
- For selected examples on the synthesis and transformation of β-amino alkylboronic esters, see:
(a) Z. Liu, H.-Q. Ni, T. Zeng and K. M. Engle, J. Am. Chem. Soc., 2018, 140, 3223 CrossRef CAS PubMed;
(b) A. Fawcett, A. Murtaza, C. H. U. Gregson and V. K. Aggarwal, J. Am. Chem. Soc., 2019, 141, 4573 CrossRef CAS PubMed;
(c) R. Oeschger, B. Su, I. Yu, C. Ehinger, E. Romero, S. He and J. Hartwig, Science, 2020, 368, 736 CrossRef CAS PubMed;
(d) Q. Xie and G. Dong, J. Am. Chem. Soc., 2021, 143, 14422 CrossRef CAS PubMed.
- M. Presset, D. Oehlrich, F. Rombouts and G. A. Molander, Org. Lett., 2013, 15, 1528 CrossRef CAS PubMed.
- G. Zweifel, H. Arzoumanian and C. C. Whitney, J. Am. Chem. Soc., 1967, 89, 3652 CrossRef CAS.
-
(a) L. Zhang, G. J. Lovinger, E. K. Edelstein, A. A. Szymaniak, M. P. Chierchia and J. P. Morken, Science, 2016, 351, 70 CrossRef CAS PubMed ; For related examples:;
(b) C. Law, E. Kativhu, J. Wang and J. P. Morken, Angew. Chem., Int. Ed., 2020, 59, 10311 CrossRef CAS PubMed;
(c) Y. Meng, Z. Kong and J. P. Morken, Angew. Chem., Int. Ed., 2020, 59, 8456 CrossRef CAS PubMed;
(d) M. D. Aparece, W. Hu and J. P. Morken, ACS Catal., 2019, 9, 11381 CrossRef CAS PubMed;
(e) C. Law, Y. Meng, S. M. Koo and J. P. Morken, Angew. Chem., Int. Ed., 2019, 58, 6654 CrossRef CAS PubMed;
(f) M. D. Aparece, C. Gao, G. J. Lovinger and J. P. Morken, Angew. Chem., Int. Ed., 2019, 58, 592 CrossRef CAS PubMed;
(g) J. A. Myhill, C. A. Wilhelmsen, L. Zhang and J. P. Morken, J. Am. Chem. Soc., 2018, 140, 15181 CrossRef CAS PubMed;
(h) J. A. Myhill, L. Zhang, G. J. Lovinger and J. P. Morken, Angew. Chem., Int. Ed., 2018, 57, 12799 CrossRef CAS PubMed;
(i) M. Chierchia, C. Law and J. P. Morken, Angew. Chem., Int. Ed., 2017, 56, 11870 CrossRef CAS PubMed;
(j) E. K. Edelstein, S. Namirembe and J. P. Morken, J. Am. Chem. Soc., 2017, 139, 5027 CrossRef CAS PubMed;
(k) G. J. Lovinger, M. D. Aparece and J. P. Morken, J. Am. Chem. Soc., 2017, 139, 3153 CrossRef CAS PubMed.
-
(a) C. Gerleve, M. Kischkewitz and A. Studer, Angew. Chem., Int. Ed., 2018, 57, 2441 CrossRef CAS PubMed;
(b) M. Kischkewitz, C. Gerleve and A. Studer, Org. Lett., 2018, 20, 3666 CrossRef CAS PubMed;
(c) M. Kischkewitz, K. Okamoto, C. Mück-Lichtenfeld and A. Studer, Science, 2017, 355, 936 CrossRef CAS PubMed.
-
(a) R. Davenport, M. Silvi, A. Noble, Z. Hosni, N. Fey and V. K. Aggarwal, Angew. Chem., Int. Ed., 2020, 59, 6525 CrossRef CAS PubMed;
(b) S. Yu, C. Jing, A. Noble and V. K. Aggarwal, Angew. Chem., Int. Ed., 2020, 59, 3917 CrossRef CAS PubMed;
(c) M. Silvi, C. Sandford and V. K. Aggarwal, J. Am. Chem. Soc., 2017, 139, 5736 CrossRef CAS PubMed.
- N. D. C. Tappin, M. Gnägi-Lux and P. Renaud, Chem.–Eur. J., 2018, 24, 11498 CrossRef CAS PubMed.
-
(a) R. J. Armstrong, C. Sandford, C. García-Ruiz and V. K. Aggarwal, Chem. Commun., 2017, 53, 4922 RSC;
(b) Z. Tao, K. A. Robb, J. L. Panger and S. E. Denmark, J. Am. Chem. Soc., 2018, 140, 15621 CrossRef CAS PubMed.
-
(a) D. Zheng, K. Jana, F. A. Alasmary, C. G. Daniliuc and A. Studer, Org. Lett., 2021, 23, 7688 CrossRef CAS PubMed;
(b) H. Jiang, X. Yu, C. G. Daniliuc and A. Studer, Angew. Chem., Int. Ed., 2021, 60, 14399 CrossRef CAS PubMed;
(c) H. Jiang, G. Seidler and A. Studer, Angew. Chem., Int. Ed., 2019, 58, 16528 CrossRef CAS PubMed;
(d) D. Zheng and A. Studer, Angew. Chem., Int. Ed., 2019, 58, 15803 CrossRef CAS PubMed;
(e) H. Jiang and A. Studer, Chem.–Eur. J., 2019, 25, 7105 CrossRef CAS PubMed;
(f) H. Jiang and A. Studer, Chem.–Eur. J., 2019, 25, 516 CAS;
(g) H. Jiang and A. Studer, Angew. Chem., Int. Ed., 2018, 57, 10707 CrossRef CAS;
(h) Y. Li, M. Hartmann, C. G. Daniliuc and A. Studer, Chem. Commun., 2015, 51, 5706 RSC;
(i) J. Guin, C. Mück-Lichtenfeld, S. Grimme and A. Studer, J. Am. Chem. Soc., 2007, 129, 4498 CrossRef CAS PubMed.
-
(a) D. Wang, K. Jana and A. Studer, Org. Lett., 2021, 23, 5879 Search PubMed;
(b) C. You and A. Studer, Angew. Chem., Int. Ed., 2020, 59, 17245 CrossRef CAS PubMed;
(c) D. Wang, C. Mück-Lichtenfeld and A. Studer, J. Am. Chem. Soc., 2020, 142, 9119 CrossRef CAS PubMed;
(d) C. Gerleve and A. Studer, Angew. Chem., Int. Ed., 2020, 59, 15468 CrossRef CAS PubMed;
(e) D. Wang, C. Mück-Lichtenfeld and A. Studer, J. Am. Chem. Soc., 2019, 141, 14126 CrossRef CAS PubMed;
(f) S. Das, C. G. Daniliuc and A. Studer, Angew. Chem., Int. Ed., 2018, 57, 4053 CrossRef CAS PubMed.
- For selected examples on intermolecular chloramination of olefins using N-chloroamides:
(a) R.-Z. Liu, J. Li, J. Sun, X.-G. Liu, S. Qu, P. Li and B. Zhang, Angew. Chem., Int. Ed., 2020, 59, 4428 CrossRef CAS PubMed;
(b) N. Zhu, Y. Li and H. Bao, Org. Chem. Front., 2018, 5, 1303 RSC;
(c) Q. Qin, D. Ren and S. Yu, Org. Biomol. Chem., 2015, 13, 10295 RSC.
- L. De Luca, G. Giacomelli and G. Nieddu, Synlett, 2005, 2, 223 Search PubMed.
- References about single-electron oxidation of boronate complexes, see:
(a) D. Kaiser, A. Noble, V. Fasano and V. K. Aggarwal, J. Am. Chem. Soc., 2019, 141, 14104 CrossRef CAS PubMed;
(b) C. Shu, A. Noble and V. K. Aggarwal, Angew. Chem., Int. Ed., 2019, 58, 3870 CrossRef CAS PubMed;
(c) M. Silvi, R. Schrof, A. Noble and V. K. Aggarwal, Chem.–Eur. J., 2018, 24, 4279 CrossRef CAS PubMed;
(d) R. Rasappan and V. K. Aggarwal, Nat. Chem., 2014, 6, 810 CrossRef CAS PubMed;
(e) F. Clausen, M. Kischkewitz, K. Bergander and A. Studer, Chem. Sci., 2019, 10, 6210 RSC.
- References about direct photolysis of the N–Cl bond, see:
(a) R. K. Quinn, Z. A. Könst, S. E. Michalak, Y. Schmidt, A. R. Szklarski, A. R. Flores, S. Nam, D. A. Horne, C. D. Vanderwal and E. J. Alexanian, J. Am. Chem. Soc., 2016, 138, 696 CrossRef CAS PubMed;
(b) W. Lee, H. J. Jeon, H. Jung, D. Kim, S. Seo and S. Chang, Chem, 2020, 7, 495 CrossRef.
-
(a) A. Studer and D. P. Curran, Nat. Chem., 2014, 6, 765 CrossRef CAS PubMed;
(b) A. Studer and D. P. Curran, Angew. Chem., Int. Ed., 2016, 55, 58 CrossRef CAS PubMed.
- L. Lewis-Borrell, M. Sneha, I. P. Clark, V. Fasano, A. Noble, V. K. Aggarwal and A. J. Orr-Ewing, J. Am. Chem. Soc., 2021, 143, 17191 CrossRef CAS PubMed.
- R. J. Armstrong, W. Niwetmarin and V. K. Aggarwal, Org. Lett., 2017, 19, 2762 CrossRef CAS PubMed.
- A. Bonet, M. Odachowski, D. Leonori, S. Essafi and V. K. Aggarwal, Nat. Chem., 2014, 6, 584 CrossRef CAS PubMed.
Footnote |
† Electronic supplementary information (ESI) available. See DOI: 10.1039/d1sc05811h |
|
This journal is © The Royal Society of Chemistry 2021 |
Click here to see how this site uses Cookies. View our privacy policy here.