DOI:
10.1039/D1SC02212A
(Edge Article)
Chem. Sci., 2021,
12, 10467-10473
A general strategy for the synthesis of α-trifluoromethyl- and α-perfluoroalkyl-β-lactams via palladium-catalyzed carbonylation†
Received
21st April 2021
, Accepted 30th June 2021
First published on 12th July 2021
Abstract
β-Lactam compounds play a key role in medicinal chemistry, specifically as the most important class of antibiotics. Here, we report a novel one-step approach for the synthesis of α-(trifluoromethyl)-β-lactams and related products from fluorinated olefins, anilines and CO. Utilization of an advanced palladium catalyst system with the Ruphos ligand allows for selective cycloaminocarbonylations to give diverse fluorinated β-lactams in high yields.
Introduction
The synthesis of fluorinated organic molecules has become one of the most active and dynamic areas in chemistry.1,2 In fact, the launch of modern pharmaceuticals and plant protection agents as well as precise materials, e.g. for energy technologies (batteries, etc.), would be impossible without appropriate organofluorine compounds. In this respect, the development of new synthetic methodologies for the preparation of such molecules is crucial, too. Although in the past decades, many elegant protocols to directly introduce fluorine atoms or fluoroalkyl groups into a given organic substrate have been disclosed,3–6 there is a continuing interest in complementary and improved procedures, specifically the development of new methods potentially applicable on a practical scale.
Among the established synthetic toolbox for incorporation of fluorine-containing units, in particular processes for introducing CF3-groups are important. Notable examples include trifluoromethylated arenes,7 olefins,8,9 ethers,10 and allylic compounds.11 In addition, especially trifluoromethylated heterocycles11,12 are emerging as promising building blocks for many life science and material applications.
β-Lactam molecules (azetidin-2-ones) belong to a family of heterocycles, which are most known for their antibiotic properties (Scheme 1); however, it has been shown that several members of this group present many other pharmaceutical effects, e.g. neuroprotective, antioxidant, analgesic or immunomodulatory capabilities as well as interesting material properties.13–15 Despite the synthesis of many structural variants, relatively few examples of α-fluoroalkyl-substituted derivatives are known. So far, such products and especially α-(trifluoromethyl)-β-lactams are based on multistep syntheses,21,22 or special methods such as hydrogenolysis of N–O bonds,16 1,3-dipolar cycloadditions of nitrones to fluoroalkenes,17 and metal-catalyzed intramolecular C–H amidations (Scheme 1).18–20 Unfortunately, all these methods have certain shortcomings, which limit their applications to small scale.21
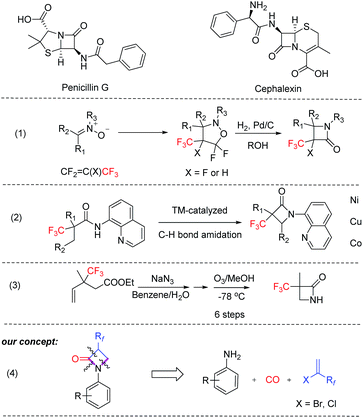 |
| Scheme 1 Selected examples of bio-relevant β-lactams and known synthesis routes for α-(trifluoromethyl)-β-lactams. | |
Based on our long-standing interest in carbonylation reactions22 and inspired by palladium-catalyzed three component coupling reactions,23 we got the idea to build up the central 4-membered ring of α-fluoroalkyl-β-lactams by a novel palladium-catalyzed aminocarbonylation of α-halo-α-fluoroalkylolefins, anilines and CO (Scheme 1). At this point, it is worthwhile mentioning that transition metal-catalyzed carbonylation reactions are not only of value for a variety of organic syntheses, but can be easily upscaled as shown by the industrial production of many fine and even bulk chemicals.24 Following our concept, apart from the inexpensive and readily available C1 source and ubiquitous anilines, also selected α-halo-fluoroalkenes are commercially available and are used to prepare various fluorinated products at present.
Herein, we report useful methodology for a direct synthesis of α-(trifluoromethyl)-β-lactams and related fluoroalkyl derivatives by selective three component carbonylation reaction in the presence of a specific palladium catalyst.
Results and discussion
Initially, the carbonylation of 4-methoxyaniline (1a) with commercially available 2-bromo-3,3,3-trifluoro-1-propene (2) as the trifluoromethylation reagent was used as the model system to study the general reaction sequence and optimize the conditions (Table 1). In first experiments various palladium precursors (2 mol%) were tested in the presence of PCy3 (5 mol%) as the ligand in 1.4-dioxane at 100 °C and comparably low CO pressure (8 bar). To our delight, the desired product 3a was afforded in up to 45% yield demonstrating the feasibility of our approach (Table 1, entries 1–6). Next, several phosphine ligands including monodentate and bidentate ones were tested. Amongst these, the sterically hindered Ru-Phos, introduced by Buchwald and co-workers for Negishi cross-coupling reactions,25 showed the best result, and the reaction yield of 3a increased to 72% (Table 1, entries 7–12). In general, the improved catalytic activity of Ruphos compared to other ligands in this palladium-catalyzed coupling process is attributed to its electron-richness and steric bulk. The specific structural features stabilize highly reactive LPd(0) intermediates during the catalytic cycle and allow for the most efficient activation of 2-bromo-3,3,3-trifluoro-1-propene 2. Changing the base from sodium bicarbonate to triethylamine, the yield of the target compound slightly increased to 78% (Table 1, entries 13–15). Finally, the effect of solvents on this aminocarbonylation process was investigated. Here, dipolar aprotic solvents gave much worse results than non-polar ones indicating that the solvent also plays an important role in the selectivity (Table 1, entries 16–20). With toluene as the optimal solvent, 3a was detected in 89% yield and isolated after separation in 83%.
Table 1 Palladium-catalyzed carbonylation of 4-methoxyaniline with 2-bromo-3,3,3-trifluoro-1-propene: screening of the reaction conditionsa
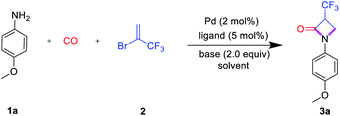 |
Entry |
Catalyst |
Ligand |
Base |
Solvent |
Yieldb (%) |
Reaction conditions: 1a (1.0 mmol), 2 (2.0 mmol), catalyst (5 mol%), base (2.0 mmol), solvent (2.0 mL), CO (8 atm), 100 °C, 12 h. Determined by 19F NMR analysis using (trifluoromethoxy)benzene as internal standard. Isolated yield. A commercially available 3rd generation Buchwald palladacycle (CAS: 1445085-77-7) was used. |
1 |
PdCl2 |
PCy3 |
NaHCO3 |
Dioxane |
6 |
2 |
Pd(OAc)2 |
PCy3 |
NaHCO3 |
Dioxane |
45 |
3 |
Pd(MeCN)2Cl2 |
PCy3 |
NaHCO3 |
Dioxane |
19 |
4 |
Pd(PPh3)2Cl2 |
PCy3 |
NaHCO3 |
Dioxane |
36 |
5 |
Pd(PPh3)4 |
PCy3 |
NaHCO3 |
Dioxane |
31 |
6 |
Pd(TFA)2 |
PCy3 |
NaHCO3 |
Dioxane |
26 |
7 |
Pd(OAc)2 |
DPPP |
NaHCO3 |
Dioxane |
19 |
8 |
Pd(OAc)2 |
X-Phos |
NaHCO3 |
Dioxane |
38 |
9 |
Pd(OAc)2 |
JohnPhos |
NaHCO3 |
Dioxane |
41 |
10 |
Pd(OAc)2 |
Dave-Phos |
NaHCO3 |
Dioxane |
51 |
11 |
Pd(OAc)2 |
S-Phos |
NaHCO3 |
Dioxane |
58 |
12 |
Pd(OAc)2 |
Ru-Phos |
NaHCO3 |
Dioxane |
72 |
13 |
Pd(OAc)2 |
Ru-Phos |
Na2HPO4 |
Dioxane |
16 |
14 |
Pd(OAc)2 |
Ru-Phos |
NEt3 |
Dioxane |
78 |
15 |
Pd(OAc)2 |
Ru-Phos |
K2CO3 |
Dioxane |
49 |
16 |
Pd(OAc)2 |
Ru-Phos |
NEt3 |
MeCN |
23 |
17 |
Pd(OAc)2 |
Ru-Phos |
NEt3 |
THF |
35 |
18 |
Pd(OAc)2 |
Ru-Phos |
NEt3 |
DMF |
14 |
19 |
Pd(OAc)2 |
Ru-Phos |
NEt3 |
MePh |
89, 83c |
20 |
Pd–Ru-Phosd |
Ru-Phos |
NEt3 |
MePh |
72c |
Structure of ligands |
|
Further to the envisioned cycloaminocarbonylation process, several unwanted side-reactions may take place here (Scheme 2, below). Nevertheless, the combination of Pd(OAc)2/Ru-Phos under the optimized conditions gave excellent selectivity for 3a and no amines or β-amino-acrylates were observed.
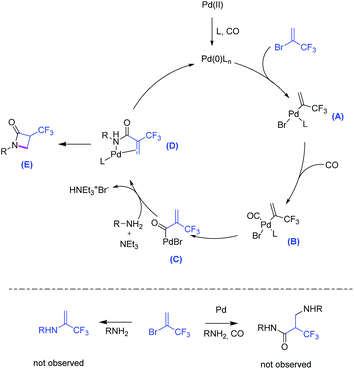 |
| Scheme 2 Proposed mechanism and potential side reactions. | |
We assume that this reaction proceeds via the following steps: first, the active Pd(0) catalyst is generated from the more stable Pd(II) precursor by reduction with phosphine, base or CO. Then, oxidative addition of Pd(0) into 2-bromo-3,3,3-trifluoro-1-propene forms intermediate A. Subsequent CO coordination (intermediate B) and carbonyl insertion through ligand migration forms acyl palladium compound C. Nucleophilic attack of the amine occurs to provide amide D, which finally undergoes an intramolecular Michael addition to obtain β-lactam E.
To explore the mechanism of this novel carbonylation reaction, several control experiments were performed (Scheme 3). First, we synthesized amide 4 by reaction of 2-bromo-1,1,1-trifluoro-1-propene and phenyl isocyanate under microwave conditions. Indeed, 4 easily convert by an intramolecular Michael addition to product 3a in the presence or absence of the palladium catalyst with excellent yield. Next, the potential intermediate 5 was prepared by addition of 4-methoxy-1-iodobenzene to 2-bromo-1,1,1-trifluoropropylamine and subsequently reacted with carbon monoxide in the presence of the palladium catalyst under standard conditions. However, in this case, we did not observe target compound 3a. To investigate the importance of the trifluoromethyl group for this transformation, 2-bromopropene 6 was applied as reagent under standard reaction conditions. Notably, the related β-lactam 7 was not obtained, but instead amide 8 was formed, which shows that fluoroalkyl groups play a pivotal role in this overall transformation.
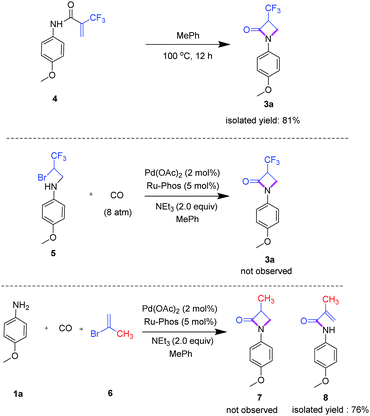 |
| Scheme 3 Control experiments. | |
After having optimal reaction conditions in hand, the selective cyclocarbonylation of several anilines was investigated (Table 2). After completion, the reaction mixtures were purified by column chromatography, and isolated product yields were determined. The results show that the electronic property of substituents on the aniline have no pronounced effect on the conversion and selectivity. In general, anilines with electron-rich substituents in ortho-, para- or meta-position such as alkoxy, alkyl, and amino provided the corresponding lactam products in high yield.
Table 2 Palladium-catalyzed synthesis of α-trifluoromethyl-β-lactams: variation of anilinesa
Reaction conditions: aniline (1.0 mmol), 2 (2.0 mmol), Pd(OAc)2 (2 mol%), Ru-Phos (5 mol%), NEt3 (2.0 eq.) and MePh (2.0 mL), CO (8 atm), 100 °C, 12 h. Isolated yield. |
|
Interestingly, more demanding methylthio, hydroxyl, and amino groups as well as heterocycles (indole, triazole) are well tolerated, too (Table 2, entries 3a–3i, 3q, 3v–3w). Similarly, anilines with electron-deficient substituents in meta- and para-position, e.g. fluorine, chlorine, nitrile, and ester groups, led to the corresponding trifluoromethylated lactams in 63–88% yield (Table 2, entries 3j–3l). Further investigations utilizing di- and tri-substituted anilines proceeded well, too (Table 2, entries 3r–3t). Finally, we investigated the cyclocarbonylations of p-phenylenediamine and m-phenylenediamine. Here, depending on the substitution pattern, selectively mono- or double-β-lactam formation can be observed using an excess amount (4 equiv.) of trifluoromethyl reagent (Table 2, entries 3x, 3y). We explain this behaviour by the decreased nucleophilicity of the second amino group after the first aminocarbonylation reaction in case of 1,4-phenylenediamine.
In addition, we explored the reactivity of alkyl amines, including linear and branched ones as well as N-heterocycles. Unfortunately, none of these amines furnished the desired products. Apparently, the increased nucleophilicity/basicity of these substrates significantly decrease the activity of the catalyst by blocking coordination sides.
After studying the reactions of 2-bromo-3,3,3-trifluoro-1-propene as available fluorinated building block, we investigated reactions of the related 2-bromo-3,3,4,4,4-pentafluoro-1-butene 9 with various aromatic amines. Except for 4-thiomethylaniline 1b, all anilines delivered the corresponding products 10 in good yield (Table 3). Interestingly, in case of 1b partial dehydrofluorination reaction occurred to give the α,β-unsaturated amide 11 as a cis/trans mixture (4
:
1). Furthermore, the domino aminocarbonylation sequence was tested with methyl 2-chloro-acrylate as another olefin. However, no desired product was observed, probably due to polymerization of the highly reactive olefin.
Table 3 Palladium-catalyzed cycloaminoarbonylation of 2-bromo-3,3,4,4,4-pentafluoro-1-butenea
Reaction conditions: aniline (1.0 mmol), 9 (2.0 mmol), Pd(OAc)2 (2 mol%), Ru-Phos (5 mol%), NEt3 (2.0 eq.) and MePh (4.0 mL), CO (8 atm), 100 °C, 12 h. Isolated yield. |
|
Apart from the preparation of novel fluorinated β-lactam building blocks, we envisioned that this methodology would be amenable to more biologically relevant substrates. To demonstrate this potential, two case studies were performed: imatinib mesylate, a synthetic tyrosine kinase inhibitor widely used in the clinical treatment of chronic myeloid leukemia26,27 and prostatic hyperplasia,28 was subjected to the cycloaminocarbonylation reaction with 2 to give the target compound 13 in 78% isolated yield (Scheme 4). In addition, 3-aminoestrone 14, a versatile precursor of various biologically active compounds to treat hormone-sensitive diseases such as prostate and breast cancers,29 led to β-lactam 15 in high isolated yield (84%). Notably, in both cases no further optimization of the reaction conditions was necessary showing the robustness of the procedure.
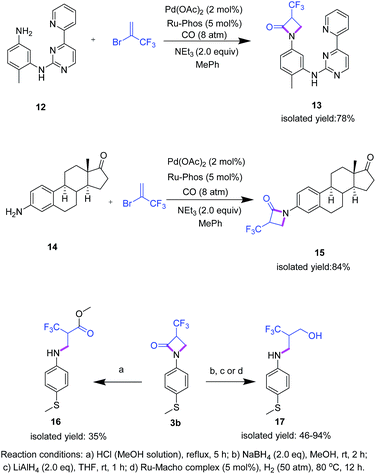 |
| Scheme 4 Synthesis of biologically relevant α-trifluoromethyl-β-lactams and derivatizations. | |
Having a general protocol vide supra for the functionalization of aromatic amines to the corresponding α-trifluoromethylated β-lactams and related compounds in hand, this straightforward and practical methodology offers also access to a variety of other interesting fluoroalkyl building blocks following standard procedures. For example, α-trifluoromethyl-β-arylamino acid derivatives should be easily available by classic amide hydrolysis, while (catalytic) reductions offer an entrée to 2-trifluoromethyl-3-aminopropanols (Scheme 4). Indeed, simply refluxing 3b in an anhydrous hydrogen chloride methanol solution gave 16. Furthermore, using 3b the respective amino alcohol 17 is obtained by reduction with NaBH4 or LiAlH4. Alternatively, 17 can be formed by Ru-catalyzed hydrogenation (for details see ESI†).
Conclusion
In summary, we developed a general and convenient palladium-catalyzed cycloaminocarbonylation of 2-fluoroalkyl-2-halo-olefins. Through this novel reaction, α-CF3– and related fluoroalklyl-substituted lactams can be synthesized in one step from commercially available 3 and anilines. The palladium catalyst system containing the Ru-Phos ligand allows the selective preparation of a variety of interesting functionalized synthetic building blocks in good to high yields.
Author contributions
Yang Li discovered the reaction. Cai-Lin Zhang, Wei-Heng Huang, Ning Sun, Meng Hao were preparing the scope and the experimental data. Helfried Neumann has corrected the article and checked the NMR data and Matthias Beller wrote the article.
Conflicts of interest
The authors declare that there are no conflicts of interest regarding the publication of this paper.
Acknowledgements
We are grateful for the financial support by the National Natural Science Foundation of China (GZ1645), the Key Research & Development Project in Shaanxi Province (2017ZDXM-GY-040), and the Doctoral Scientific Research Foundation of Xi'an Polytechnic University (107020336). In addition, we acknowledge funding by the Federal Ministry BMBF, Germany and the state of Mecklenburg-Western Pomerania.
Notes and references
-
(a) R. D. Chambers, Fluorine in Organic Chemistry; Blackwell Publishing: Oxford, UK, 2004 CrossRef;
(b) P. Kirsch, Modern Fluoroorganic Chemistry; Wiley-VCH: Weinheim, Germany, 2004 CrossRef;
(c) T. Hiyama, K. Kanie, T. Kusumoto, Y. Morizawa and M. Shimizu, Organofluorine Compounds: Chemistry and Applications, Springer-Verlag, Berlin, Germany, 2000 CrossRef.
-
(a) S. Purser, P. R. Moore, S. Swallow and V. Gouverneur, Chem. Soc. Rev., 2008, 37, 320–330 RSC;
(b) P. K. Mykhailiuk, Chem. Rev., 2021, 121, 1670–1715 CrossRef CAS PubMed;
(c) J. Moschner, V. Stulberg, R. Fernandes, S. Huhmann, J. Leppkes and B. Koksch, Chem. Rev., 2019, 119, 10718–10801 CrossRef CAS PubMed;
(d) B. Linclau, A. Ardá, N.-C. Reichardt, M. Sollogoub, L. Unione, S. P. Vincent and J. Jiménez-Barbero, Chem. Soc. Rev., 2020, 49, 3863–3888 RSC.
-
(a) J. Wiedemann, T. Heiner, G. Mloston, G. K. Surya, S. Prakash and G. A. Olah, Angew. Chem., 1998, 110, 880–881 (Angew. Chem., Int. Ed., 1998, 37, 820–821) CrossRef;
(b) N. Aiguabella, C. Pozo, X. Verdaguer, S. Fustero and A. Riera, Angew. Chem., 2013, 125, 5463–5467 (Angew. Chem. Int. Ed, 2013, 52, 5355–5359) CrossRef;
(c) M. Brambilla and M. Tredwell, Angew. Chem, 2017, 129, 12143–12147 (Angew. Chem. Ind. Ed., 2017, 56, 11981–11985) CrossRef;
(d) A. M. Cook and C. Wolf, Angew. Chem., 2016, 128, 2982–2986 (Angew. Chem. Ind. Ed., 2016, 55, 2929–2933) CrossRef.
-
(a) A. Tinoco, V. Steck, V. Tyagi and R. Fasan, J. Am. Chem. Soc., 2017, 139, 5293–5296 CrossRef CAS PubMed;
(b) C.-P. Zhang and D. Vicic, J. Am. Chem. Soc., 2012, 134, 183–185 CrossRef CAS PubMed;
(c) Q.-W. Zhang, A. Brusoe, V. Mascitti, K. D. Hesp, D. C. Blakemore, J. Kohrt and J. Hartwig, Angew. Chem., 2016, 128, 9910–9914 (Angew. Chem., Int. Ed., 2016, 55, 9758–9762) CrossRef;
(d) Q. Wang, C. Ni, M. Hu, Q. Xie, Q. Liu, S. Pan and J. Hu, Angew. Chem., 2020, 132, 8585–8589 (Angew. Chem., Int. Ed., 2020, 59, 8507–8511) CrossRef.
-
(a) J. Nie, H.-C. Guo, D. Cahard and J.-A. Ma, Chem. Rev., 2011, 111, 455–529 CrossRef CAS PubMed;
(b) X. Liu, C. Xu and Q. Liu, Chem. Rev., 2015, 115, 683–730 CrossRef CAS;
(c) Z. Xiong, F. Zhang, Y. Yu, Z. Tan and G. Zhu, Org. Lett., 2020, 22, 4088–4092 CrossRef CAS PubMed;
(d) M. B. Johansen, O. R. Gedde, T. S. Mayer and T. Skrydstrup, Org. Lett., 2020, 22, 4068–4072 CrossRef CAS PubMed;
(e) K. Zhou, J. Geng, D. Wang, J. Zhang and Y. Zhao, Org. Lett., 2020, 22, 5109–5114 CrossRef CAS PubMed;
(f) N. J. Young, V. W. Pike and C. Taddei, ACS Omega, 2020, 5, 19557–19564, DOI:10.1021/acsomega.0c02027;
(g) X. Gao, Y.-L. Xiao, X. Wan and X. Zhang, Angew. Chem., 2018, 130, 3241–3245 (Angew. Chem., Int. Ed., 2018, 57, 3187–3191) CrossRef;
(h) R. Cheng, H.-Y. Zhao, S. Zhang and X. Zhang, ACS Catal., 2020, 10, 36–42 CrossRef CAS.
-
(a) J. Ge, Q. Ding, X. Wang and Y. Peng, J. Org. Chem., 2020, 85, 7658–7665 CrossRef CAS PubMed;
(b) B. M. Trost, Y. Wang and C.-I. Hung, Nat. Chem., 2020, 12, 294–301 CrossRef CAS PubMed;
(c) J. Dong, L. Shi, L. Pan, X. Xu and Q. Liu, Nat. Catal., 2020 DOI:10.1038/srep26957;
(d) P. Sarver, V. Bacauanu, D. M. Schultz, D. A. DiRocco, Y.-H. Lam, E. C. Sherer and D. W. C. MacMillan, Nat. Chem., 2020, 12, 459–467 CrossRef CAS PubMed;
(e) R. C. Simon, E. Busto, N. Richter, V. Resch, K. N. Houk and W. Kroutil, Nat. Commun., 2016, 7, 13323–13328 CrossRef CAS PubMed;
(f) S. Choi, S. M. Kim, J. W. Yang, S. W. Kim and J. Cho, Nat. Commun., 2014, 5, 4881–4887 CrossRef CAS PubMed.
-
(a) E. Mejía and A. Togni, ACS Catal., 2012, 2, 521–552 CrossRef;
(b) T. Liu and Q. Shen, Org. Lett., 2011, 13, 2342–2345 CrossRef CAS PubMed;
(c) L. Chu and F.-L. Qing, Org. Lett., 2010, 12, 5060–5063 CrossRef CAS PubMed;
(d) R. J. Lundgren and M. Stradiotto, Angew. Chem., 2010, 122, 9510–9512 (Angew. Chem., Int. Ed., 2010, 49, 9322–9324) CrossRef;
(e) E. J. Cho, T. D. Senecal, T. Kinzel, Y. Zhang, D. A. Watson and S. L. Buchwald, Science, 2010, 328, 1679–1681 CrossRef CAS PubMed;
(f) V. V. Grushin and W. J. Marshall, J. Am. Chem. Soc., 2006, 128, 12644–12645 CrossRef CAS PubMed;
(g) T. D. Senecal, A. T. Parsons and S. L. Buchwald, J. Org. Chem., 2011, 76, 1174–1176 CrossRef CAS PubMed;
(h) N. D. Ball, J. W. Kampf and M. S. Sanford, J. Am. Chem. Soc., 2010, 132, 2878–2879 CrossRef CAS PubMed;
(i) X. Wang, L. Truesdale and J.-Q. Yu, J. Am. Chem. Soc., 2010, 132, 3648–3649 CrossRef CAS PubMed;
(j) B. Yang, D. Yu, X.-H. Xu and F.-L. Qing, ACS Catal., 2018, 8, 2839–2843 CrossRef CAS;
(k) M. J. Orstein, H. Hou, C. Cheng and J. F. Hartwig, Angew. Chem., 2016, 128, 8186–8189 (Angew. Chem. Int. Ed., 2016, 55, 8054–8057) CrossRef;
(l) F. Ye, F. Berger, H. Jia, J. Ford, A. Wortman, B. J. el, C. Genicot and T. Ritter, Angew. Chem., 2019, 131, 14757–14761 (Angew. Chem. Int. Ed., 2019, 58, 14615–14619) CrossRef;
(m) L. Chang, N. Klipfel, L. Dechoux and S. Thorimbert, Green Chem., 2018, 20, 1491–1498 RSC.
-
(a) A. T. Parsons, T. D. Senecal and S. L. Buchwald, Angew.
Chem., 2012, 124, 3001–3004 (Angew. Chem., Int. Ed., 2012, 51, 2947–2950) CrossRef;
(b) C. Feng and T.-P. Loh, Chem. Sci., 2012, 3, 3458–3462 RSC;
(c) C. Feng and T.-P. Loh, Angew. Chem., 2013, 125, 12640–12643 (Angew. Chem., Int. Ed., 2013, 52, 12414–12417) CrossRef;
(d) X. Wang, Y. Ye, G. Ji, Y. Xu, S. Zhang, J. Feng, Y. Zhang and J. Wang, Org. Lett., 2013, 15, 3730–3733 CrossRef CAS PubMed;
(e) N. O. Ilchenko, P. G. Janson and K. J. Szabó, Chem. Commun., 2013, 49, 6614–6616 RSC;
(f) X.-P. Wang, J.-H. Lin, C.-P. Zhang, J.-C. Xiao and X. Zheng, J. Org. Chem., 2013, 9, 2635–2640 Search PubMed;
(g) Z. Fang, Y. Ning, P. Mi, P. Liao and X. Bi, Org. Lett., 2014, 16, 1522–1525 CrossRef CAS;
(h) A. Prieto, E. Jeamet, N. Monteiro, D. Bouyssi and O. Baudoin, Org. Lett., 2014, 16, 4770–4773 CrossRef CAS PubMed;
(i) N. Iqbal, S. Choi, E. Kim and E. J. Cho, J. Org. Chem., 2012, 77, 11383–11387 CrossRef CAS PubMed;
(j) Z.-B. He, T. Luo, M.-Y. Hu, Y.-J. Cao and J.-B. Hu, Angew. Chem., 2012, 124, 4010–4013 (Angew. Chem., Int. Ed., 2012, 51, 3940–3947) CrossRef.
-
(a) S. Qi, S. Gao, X. Xie, J. Yang and J. Zhang, Org. Lett., 2020, 22, 5229–5234 CrossRef CAS PubMed;
(b) F. Tian, G. Yan and J. Yu, Chem. Commun., 2019, 55, 13486–13505 RSC;
(c) P. V. Ramachandran and B. Otoo, Chem. Commun., 2015, 51, 12388–12390 RSC;
(d) L. Debien, B. Quiclet-Sire and S. S. Zard, Org. Lett., 2012, 14, 5118–5121 CrossRef CAS PubMed;
(e) M. Kawatsura, J. Namioka, K. Kajita, M. Yamamoto, H. Tsuji and T. Itoh, Org. Lett., 2011, 13, 3285–3287 CrossRef CAS PubMed.
- For allylic trifluoromethylation, see:
(a) A. T. Parsons and S. L. Buchwald, Angew. Chem., 2011, 123, 9286–9289 (Angew. Chem., Int. Ed., 2011, 50, 9120–9123) CrossRef;
(b) X. Wang, Y. Ye, S. Zhang, J. Feng, Y. Xu, Y. Zhang and J. Wang, J. Am. Chem. Soc., 2011, 133, 16410–16413 CrossRef CAS PubMed;
(c) R. Shimizu, H. Egami, Y. Hamashima and M. Sodeoka, Angew. Chem., 2012, 124, 4655–4658 (Angew. Chem., Int. Ed., 2012, 51, 4577–4580) CrossRef;
(d) S. Mizuta, O. Galicia-Lopez, K. M. Engle, S. Verhoog, K. Wheelhouse, G. Rassias and V. Gouverneur, Chem.–Eur. J., 2012, 18, 8583–8587 CrossRef CAS;
(e) W. Yang, D. Ma, Y. Zhou, X. Dong, Z. Lin and J. Sun, Angew. Chem., 2018, 130, 12273–12277 (Angew. Chem., Int. Ed., 2018, 57, 12097–12101) CrossRef;
(f) Y. Karuo, T. Hayashi, A. Tarui, K. Sato, K. Kawai and M. Omote, J. Org. Chem., 2020, 85, 5104–5108 CrossRef CAS PubMed.
- For oxytrifluoromethylation, see:
(a) R. Zhu and S. L. Buchwald, J. Am. Chem. Soc., 2012, 134, 12462–12465 CrossRef CAS PubMed;
(b) Y. Li and A. Studer, Angew. Chem., 2012, 124, 8345–8348 (Angew. Chem., Int. Ed., 2012, 51, 8221–8224) CrossRef;
(c) H. Egami, R. Shimizu and M. Sodeoka, Tetrahedron Lett., 2012, 53, 5503–5506 CrossRef CAS;
(d) P. G. Janson, I. Ghoneim, N. O. Ilchenko and K. J. Szabo, Org. Lett., 2012, 14, 2882–2885 CrossRef CAS PubMed;
(e) R. Zhu and S. L. Buchwald, Angew. Chem., 2013, 125, 12887–12890 (Angew. Chem., Int. Ed., 2013, 52, 12655–12658) CrossRef;
(f) D.-F. Lu, C.-L. Zhu and H. Xu, Chem. Sci., 2013, 4, 2478–2482 RSC;
(g) Y.-T. He, L.-H. Li, Y.-F. Yang, Y.-Q. Wang, J.-Y. Luo, X.-Y. Liu and Y.-M. Liang, Chem. Commun., 2013, 49, 5687–5689 RSC;
(h) L. Li, Q.-Y. Chen and Y. Guo, J. Org. Chem., 2014, 79, 5145–5152 CrossRef CAS PubMed;
(i) Y.-P. Xiong, M.-Y. Wu, X.-Y. Zhang, C.-L. Ma, L. Huang, L.-J. Zhao, B. Tan and X.-Y. Liu, Org. Lett., 2014, 16, 1000–1003 CrossRef CAS PubMed;
(j) H. Egami, R. Shimizu, Y. Usui and M. Sodeoka, J. Fluorine Chem., 2014, 167, 172–178 CrossRef CAS;
(k) H. Egami, T. Ide, M. Fujita, T. Tojo, Y. Hamashima and M. Sodeoka, Chem.–Eur. J., 2014, 20, 12061–12065 CrossRef CAS PubMed;
(l) J.-B. Liu, C. Chen, L. Chu, Z.-H. Chen, X.-H. Xu and F.-L. Qing, Angew. Chem., 2015, 127, 12005–12008 (Angew. Chem., Int. Ed., 2015, 54, 11839–11842) CrossRef;
(m) Q.-W. Zhang and J. F. Hartwig, Chem. Commun., 2018, 54, 10124–10127 RSC;
(n) M. Zhou, C. Ni, Y. Zeng and J. Hu, J. Am. Chem. Soc., 2018, 140, 6801–6805 CrossRef CAS PubMed.
-
(a) F. Li, J. Wang, M. Xu, X. Zhao, X. Zhou, W.-X. Zhao and Q. Liu, Org. Biomol. Chem., 2016, 14, 3981–3988 RSC;
(b) L. Zhao, P. Li, X.-Y. Xie and L. Wang, Org. Chem. Front., 2018, 5, 1689–1697 RSC;
(c) X.-M. Ji, W. Liang, C. Fan and R.-Y. Tang, RSC Adv., 2015, 5, 29766–29773 RSC;
(d) C. Fan, J. Song, G. L. Qiu, G. Liu and J. Wu, Org. Chem. Front., 2014, 1, 924–928 RSC;
(e) T. N. Ngo, S. A. Ejaz, T. Q. Hung, T. Dang, J. Iqbal, J. Lecka, J. Sévigny and P. Langer, Org. Biomol. Chem., 2015, 13, 8277–8290 RSC;
(f) X. Zhou, C. Huang, Y. Zeng, J. Xiong, Y. Xiao and J. Zhang, Chem. Commun., 2017, 53, 1084–1087 RSC;
(g) B. Garudachari, A. M. Isloor, M. N. Satyanaraya, K. Ananda and H.-K. Fun, RSC Adv., 2014, 4, 30864–30875 RSC;
(h) B. Jismy, M. Akssira, D. Knez, G. Guillaumet, S. Gobec and M. Abarbri, New J. Chem., 2019, 43, 9961–9968 RSC.
-
(a) W.-Q. Zuo, N.-Y. Wang, Y.-X. Zhu, L. Liu, K.-J. Xiao, L.-D. Zhang, C. Gao, Z.-H. Liu, X.-Y. You, Y.-J. Shi, C.-T. Peng, K. Ran, H. Tang and L.-T. Yu, RSC Adv., 2016, 6, 40277–40286 RSC;
(b) C. Masusai, D. Soorukram, C. Kuhakarn, P. Tuchinda, C. Pakawatchai, S. Saithong, V. Reutrakal and M. Pohmakotr, Org. Biomol. Chem., 2013, 11, 6650–6658 RSC;
(c) A. Rahman and X. Lin, Org. Biomol. Chem., 2018, 16, 1367–1374 RSC;
(d) K. Clapham, A. S. Batsanow, M. R. Bryce and B. Tarbit, Org. Biomol. Chem., 2009, 7, 2155–2161 RSC;
(e) F.-Z. Xu, Y.-Y. Wang, D.-X. Luo, G. Yu, S.-X. Guo, H. Fu, Y. H. Zhao and J. Wu, RSC Adv., 2018, 8, 6306–6314 RSC;
(f) V. M. Tkachuk, V. A. Sukach, K. V. Kovalchuk, M. V. Vovk and V. G. Nenajdenko, Org. Biomol. Chem., 2015, 13, 1420–1428 RSC;
(g) M. B. Johansen and A. T. Lindhardt, Org. Biomol. Chem., 2020, 18, 1417–1425 RSC;
(h) A. T. Davies, P. M. Pickett, A. M. Z. Slawin and A. D. Smith, ACS Catal., 2014, 4, 2696–2700 CrossRef CAS;
(i) H.-Y. Li, I. Delucca, S. Drummond and G. A. Boswell, J. Org. Chem., 1997, 62, 2550–2554 CrossRef CAS PubMed;
(j) H. Uno, T. Imai, K. Harada and N. Shibata, ACS Catal., 2020, 10, 1454–1459 CrossRef CAS;
(k) G. Masson, S. Rioton, D. Pardo and G. J. Cossy, Org. Lett., 2018, 20, 5019–5022 CrossRef CAS PubMed;
(l) M. A. Honey, R. Pasceri, W. Lewis and C. L. Moody, J. Org. Chem., 2012, 77, 1396–1405 CrossRef CAS PubMed;
(m) L. Qin and S. Z. Zard, Org. Lett., 2015, 17, 1577–1580 CrossRef CAS PubMed.
-
(a) A. K. Bose, J. C. Kapur, B. Dayal and M. S. Manhas, J. Org. Chem., 1974, 39, 312–315 CrossRef CAS;
(b) A. K. Bose, M. S. Manhas, S. D. Kapur and S. G. Amin, J. Med. Chem., 1974, 17, 541–544 CrossRef CAS PubMed;
(c) M. S. Manhas, M. Ghosh and A. K. Bose, J. Org. Chem., 1990, 55, 575–580 CrossRef CAS;
(d) G. M. Torres, M. L. H. Macias and J. S. Quesnel, J. Org. Chem., 2016, 81, 12106–12115 CrossRef CAS PubMed;
(e) X.-F. Ren, M. I. Konaklieva, H. Shi, S. Dickey, D. V. Lim, J. Gonzalez and E. Turos, J. Org. Chem., 1998, 63, 8898–8917 CrossRef CAS;
(f) R. M. Williams, B. H. Lee, M. M. Miller and O. P. Anderson, J. Am. Chem. Soc., 1989, 111, 1073–1081 CrossRef CAS;
(g) A. Bonardi, M. Costa, B. Gabriele, G. Salerno and G. P. Chiusoli, Tetrahedron Lett., 1995, 36, 7495–7498 CrossRef CAS.
-
(a) S. Dekeukeleire, M. D. hooghe and N. D. Kimpe, J. Org. Chem., 2009, 74, 1644–1649 CrossRef CAS PubMed;
(b) R. F. Pratt, J. Med. Chem., 2016, 59, 8207–8220 CrossRef CAS PubMed;
(c) C. González-Bello, D. Rodríguez, M. Pernas, Á. Rodríguez and E. Colchón, J. Med. Chem., 2020, 63, 1859–1881 CrossRef;
(d) G. M. Torres, M. L. H. Macias, J. S. Quesnel, O. P. Williams, V. Yempally, A. A. Bengali and B. A. Arndtsen, J. Org. Chem., 2016, 81, 12106–12115 CrossRef CAS PubMed.
-
(a) C. R. Pitts and T. Lectka, Chem. Rev., 2014, 114, 7930–7953 CrossRef CAS;
(b) M. Szostak and J. Aubé, Chem. Rev., 2013, 113, 5701–5765 CrossRef CAS PubMed;
(c) R. J. Worthington and C. Melander, J. Org. Chem., 2013, 78, 4207–4213 CrossRef CAS PubMed;
(d) M. S. Lall, Y. Tao, J. T. Arcari, D. C. Boyles, M. F. Brown, D. B. Damon, S. C. Lilley, M. J. Mitton-Fry, J. Starr III, A. M. Stewart and J. Sun, Org. Process Res. Dev., 2018, 22, 212–218 CrossRef CAS;
(e) M. E. Piotti and H. Alper, J. Am. Chem. Soc., 1996, 118, 111–116 CrossRef CAS;
(f) J. A. Deyrup and S. C. Clough, J. Am. Chem. Soc., 1969, 91, 4590–4591 CrossRef CAS;
(g) T. Kaneko, J. Am. Chem. Soc., 1985, 107, 5490–5492 CrossRef CAS;
(h) H. Alper, C. P. Perera and F. R. Ahmed, J. Am. Chem. Soc., 1981, 103, 1289–1291 CrossRef CAS;
(i) A. Yada, S. Okajima and M. Murakami, J. Am. Chem. Soc., 2015, 137, 8708–8711 CrossRef CAS PubMed;
(j) M. M. C. Lo and G. C. Fu, J. Am. Chem. Soc., 2002, 124, 4572–4573 CrossRef CAS PubMed;
(k) D. Dailler, R. Rocaboy and O. Baudoin, Angew. Chem. Int. Ed., 2017, 56, 7218–7222 CrossRef CAS PubMed;
(l) T. Shu, L. Zhao, S. Li, X.-Y. Chen, C. Essen, K. Rissanen and D. Enders, Angew. Chem., Int. Ed., 2018, 57, 10985–10988 CrossRef CAS PubMed;
(m) W. Li, C. Liu, H. Zhang, K. Ye, G. Zhang, W. Zhang, Z. Duan, S. You and A. Lei, Angew. Chem., 2014, 126, 2475–2478 (Angew. Chem., Int. Ed., 2014, 53, 2443–2446) CrossRef.
- J. Jakowiecki, R. Loska and M. Makosza, J. Org. Chem., 2008, 73, 5436–5441 CrossRef CAS PubMed.
- X. Wu, Y. Zhao and H. Ge, Chem.–Eur. J., 2014, 20, 9530–9533 CrossRef CAS PubMed.
- Z. Wang, J. Ni, Y. Kuninobu and M. Kanai, Angew. Chem., 2014, 126, 3564–3567 (Angew. Chem., Int. Ed., 2014, 53, 3496–3499) CrossRef.
- X. Xu, K. Yang, Y. Zhao, H. Sun, G. Li and H. Ge, Nat. Commun., 2015, 6, 6462–6471 CrossRef.
-
(a) V. Jurčík, A. M. Z. Slawin and D. O'Hagan, Beilstein J. Org. Chem., 2011, 7, 759–766 CrossRef PubMed;
(b) T. Kiyoshi, M. Takami and M. Keiryo, Chem. Lett., 1989, 18, 1115–1118 CrossRef.
-
(a) J. Yang, J. Liu, H. Neumann, R. Franke, R. Jackstell and M. Beller, Science, 2019, 366, 1514–1517 CrossRef CAS PubMed;
(b) J. Liu, J. Yang, W. Baumann, R. Jackstell and M. Beller, Angew. Chem., 2019, 131, 10793–10797 (Angew. Chem. Int. Ed, 2019, 58, 10683–10687) CrossRef;
(c) J. Liu, J. Yang, C. Schneider, R. Franke, R. Jackstell and M. Beller, Angew. Chem., 2020, 132, 9117–9125 (Angew. Chem., Int. Ed., 2020, 59, 9032–9040) CrossRef;
(d) J. Yang, J. Liu, Y. Ge, W. Huang, F. Ferretti, H. Neumann, H. Jiao, R. Franke, R. Jackstell and M. Beller, Angew. Chem., Int. Ed., 2021, 60, 9527–9533 CrossRef CAS PubMed.
-
(a) R. H. Munday, J. R. Martinelli and S. L. Buchwald, J. Am. Chem. Soc., 2008, 130, 2754–2755 CrossRef CAS PubMed;
(b) J. E. R. Sadig, R. Foster, F. Wakenhut and M. C. Wills, J. Org. Chem., 2012, 77, 9473–9486 CrossRef CAS PubMed;
(c) B. Nguyen, E. J. Emmett and M. C. Wills, J. Am. Chem. Soc., 2010, 132, 16372–16373 CrossRef CAS PubMed;
(d) Y.-H. Yao, H.-Y. Yang, M. Chen, F. Wu, X.-X. Xu and Z.-H. Guan, J. Am. Chem. Soc., 2021, 143, 85–91 CrossRef CAS PubMed.
-
(a) G. Kiss, Chem. Rev., 2001, 101, 3435–3456 CrossRef CAS PubMed;
(b) X.-F. Wu, H. Neumann and M. Beller, Chem. Rev., 2013, 113, 1–35 CrossRef CAS PubMed;
(c) J.-B. Peng, F.-P. Wu and X.-F. Wu, Chem. Rev., 2019, 119, 2090–2127 CrossRef CAS PubMed;
(d) Y. Li, Y. Hu and X.-F. Wu, Chem. Soc. Rev., 2018, 47, 172–194 RSC;
(e) C. Zhu, J. Liu, M. B. Li and J.-E. Bäckvall, Chem. Soc. Rev., 2020, 49, 341–353 RSC;
(f) Z. Yin, J. –X. Xu and X. –F. Wu, ACS Catal., 2020, 10, 6510–6531 CrossRef CAS;
(g) S. Perrone, L. Troisi and A. Salomone, Eur. J. Org. Chem., 2019, 4626–4643 CrossRef CAS;
(h) R. Mancuso, N. Della Ca, L. Veltri, I. Ziccarelli and B. Gabriele, Catalysis, 2019, 9, 610–611 Search PubMed;
(i) G. Albano and L. A. Aronica, Eur. J. Org. Chem., 2017, 48, 7204–7221 CrossRef;
(j) K. Ma, B. S. Martin, X. Yin and M. Dai, Nat. Prod. Rep., 2019, 36, 174–219 RSC;
(k) C. Shen and X.-F. Wu, Chem.–Eur. J., 2017, 23, 2973–2987 CrossRef CAS PubMed;
(l) B. Gabriele, R. Mancuso and G. Salerno, Eur. J. Org. Chem., 2012, 35, 6825–6839 CrossRef.
- J. E. Milne and S. L. Buchwald, J. Am. Chem. Soc., 2004, 126, 13028–13032 CrossRef CAS PubMed.
-
(a) J. M. Goldman, J. V. Melo and N. Engl, J.
Med., 2003, 349(15), 1451–1464 CAS;
(b) S. G. O'Brien and M. W. Deininger, Semin. Hematol., 2003, 40, 26–30 CrossRef PubMed;
(c) F. Cervantes and M. Mauro, Cancer, 2011, 117, 4343–4354 CrossRef PubMed.
-
(a) G. W. Krystal, Leuk. Res., 2004, 28, 53–59 CrossRef PubMed;
(b) I. El Hajj Dib, M. Gallet, R. Mentaverri, N. Sevenet, M. Brazier and S. Kamel, Eur. J. Pharmacol., 2006, 551, 27–33 CrossRef CAS PubMed.
-
(a) F. Labrie, A. Bélanger, L. Cusan, C. Labrie, J. Simard, V. Luu-The, P. Diamond, J.-L. Gomez and B. Candas, Endocr. Relat. Canc., 1996, 3, 243–278 CrossRef CAS;
(b) S. M. Singh, S. Gauthier and F. Labrie, Curr. Med. Chem., 2000, 7, 211–247 CrossRef CAS PubMed.
- I.-I. Radu, D. Poirier and L. Provencher, Tetrahedron Lett., 2002, 43, 7617–7619 CrossRef CAS.
Footnote |
† Electronic supplementary information (ESI) available. See DOI: 10.1039/d1sc02212a |
|
This journal is © The Royal Society of Chemistry 2021 |
Click here to see how this site uses Cookies. View our privacy policy here.