DOI:
10.1039/D0SC07042D
(Edge Article)
Chem. Sci., 2021,
12, 4126-4131
Palladium-catalyzed remote para-C–H activation of arenes assisted by a recyclable pyridine-based template†
Received
27th December 2020
, Accepted 1st February 2021
First published on 2nd February 2021
Abstract
Direct para-selective C–H functionalization of arenes remains a daunting challenge and is still significantly restricted to a few scaffolds. Herein, we report an unprecedented pyridine-based para-directing template (DT) assisted, Pd-catalyzed para-C–H alkenylation of three classes of arenes, i.e. phenylpropanoic acids, 2-phenyl benzoic acids and benzyl alcohols, with a series of alkenes including perfluoroalkenes. Notably, the pyridine-based para-DT could be easily synthesized and readily recycled under mild conditions. These results may find application in rapid construction of para-substituted arenes and stimulate the exploration of novel methods for para-C–H functionalization of arenes.
Introduction
Transition-metal-catalyzed C–H activation assisted by directing groups (DGs) has proven to be a powerful strategy for achieving regioselective C–H functionalizations in a general manner, which is able to differentiate C–H bonds with similar electronic and steric properties in an organic molecule. The DG strategy has enabled the development of a large number of ortho-C–H and some meta-C–H transformations of arenes.1,2 In contrast, although quite a few para-C–H transformations of arenes have been disclosed by making use of arene's electronic and steric biases,3,4 the para-C–H transformation beyond the control of such biases remains a daunting challenge and is still significantly restricted to a few scaffolds. In this endeavor, Nakao and co-workers reported a breakthrough in para-C–H alkylation and borylation of arenes by cooperative nickel/aluminum and iridium/aluminum catalysis (Scheme 1a, left).5 Chattopadhyay,6 Phipps7a and Maleczka7b also independently realized elegant Ir-catalyzed, noncovalent interactions assisted para-C–H borylation of arenes (Scheme 1a, left). A series of para-C–H alkylations of arenes through alkyl radical addition have also been developed by Li,8a Frost,8b Zhao,8c–e,i Zhou,8f Zeng,8g and others (Scheme 1a, right).8 However, these non-directed para-C–H functionalizations are restricted in transformation patterns and substrate classes. Alternatively, Maiti and co-workers developed the first D-shaped nitrile-based para-directing template (DT) to assist several para-C–H functionalizations of toluene and phenol derivatives, demonstrating the possibility of using the DT strategy as a general means to achieve diverse para-C–H transformations (Scheme 1b, left).9 Dai and Yu also reported a para-C–H acetoxylation of benzoic acid derivatives assisted by an amide linked DT (Scheme 1b, right).10 Unfortunately, this DT strategy is restricted to very limited substrate classes at present. Most recently, by using the cooperation of a meta-DT and norbornene relay palladation, Yu and Houk11a as well as Maiti11b independently reported two examples of para-C–H arylation of arenes such as the phenylpropanoic acids. However, 2,6-disubstitution of the substrate is unavoidable for this method to achieve the desired selectivity, making it not a general solution. Due to the aforementioned limitations and the good performance of pyridine-based DGs in promoting ortho-1 and meta-C–H activations,2d,12 the development of a versatile method for para-C–H activation of arenes by taking the advantage of the directing ability of pyridines is highly desirable.
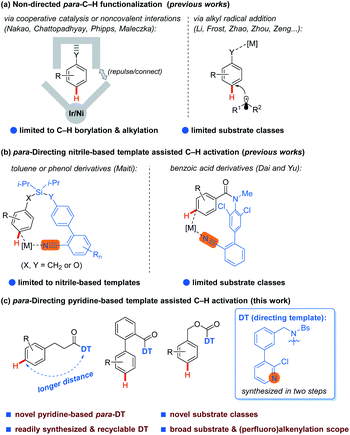 |
| Scheme 1
Para-C–H activation beyond steric & electronic control. | |
Herein, we report an unprecedented Pd-catalyzed para-selective C–H alkenylation of three classes of arenes, i.e. phenylpropanoic acids, 2-phenyl benzoic acids and benzyl alcohols, with a series of alkenes including perfluoroalkenes assisted by a novel pyridine-based para-DT (Scheme 1c). Notably, the distance between the DT and the target C–H bond is longer than previous examples using the nitrile-based para-DT,9,10 and the pyridine-based para-DT could be easily synthesized in two steps and readily recycled under mild conditions.
Results and discussion
Phenylpropionic acids are highly important structural motifs in drug molecules such as baclofen, and p-phenylenedipropionic acids is a class of important molecular unit of functional materials such as the gelator molecule of nanofiber.13 Therefore, phenylpropionic acid was selected as the model substrate for our investigation of para-C–H activation of arenes. Based on our recent work on meta-C–H activation of phenylpropionic acid14 and inspired by recent research on meta-C–H activation assisted by pyridine-based DTs,12 we designed a series of potential pyridine-based para-DTs with different substitutions to attach with phenylpropionic acid, leading to the amide substrates (Table 1, see ESI† for more DTs evaluated), where the nitrogen atom of pyridine could coordinate to the transition metal to induce site-selective C–H activation. The design of the structure of the template was also inspired by previous reported nitrile-based biphenyl containing para-directing template.9,10 After selecting C–H alkenylation as the model reaction, it was found simple template without a substituent led to low yield and moderate selectivity (1a1). Introducing an electron-donating methoxy group resulted in no desired product (1a2). It was found an electron-withdrawing substituent, which could help to reduce the coordination ability of the pyridine group, was beneficial for the reaction to produce good yield of desired product (1a3–1a7). Since the amide group could coordinate with the Pd catalyst to possibly influence the efficiency and selectivity of the reaction, the templates bearing an electron-withdrawing protecting group of the amide linkage to reduce the coordination ability were prepared, leading to higher selectivities (1a5vs.1a3 and 1a8vs.1a7). Thus, the best preliminary results were obtained with substrate 1a8 using Pd(OAc)2 as the catalyst with 3-(trifluoromethyl)pyridin-2-ol (L1) as the ligand in HFIP (hexafluoroisopropanol) at 80 °C for 12 h, affording desired para-olefinated phenylpropionic acid derivative 3a8 as the major product with good site-selectivity.
Table 1 Evaluation of pyridine-based para-DTa
Reaction conditions: 1a (0.1 mmol), 2a (2 equiv.), Pd(OAc)2 (0.01 mmol), 3-(trifluoromethyl)pyridin-2-ol (L1) (0.02 mmol), AgOAc (0.3 mmol), HFIP (1.0 mL), 80 °C, 12 h. Ratio = p : others; Ts: tosyl; Bs: phenylsulfonyl.
|
|
With the optimal pyridine-based para-DTs, we continued to optimize the reaction conditions. After extensive investigation of the reaction conditions (see ESI† for details), desired product 3a8 was produced in 79% isolated yield with elevated temperature at 90 °C and lowered concentration based on the preliminary reaction conditions (Table 2, entry 1), together with excellent site-selectivity. The yield was decreased when other pyridine-based ligands were tested (entries 2 and 3). We then tried to use a cheaper copper salt as the oxidant, and Cu(OAc)2 was found to be promising (entries 4–7). Pleasingly, a substoichiometric amount of Cu(OAc)2 could be enough to produce higher yields of 3a8 with good site-selectivity (entries 8–11) in the presence of air as the terminal oxidant and two equivalents of AcOH as the additive (see ESI† for the evaluation of other acids) using L4 as the ligand for 48 h, and the best results using Cu(OAc)2 were obtained at 75 °C (entry 10). Notably, the mount of AcOH was also important, since slightly lower yields of 3a8 would be received with lower (entry 12) of higher (entry 13) loading of this additive.
Table 2 Optimization of reaction conditions.a,b
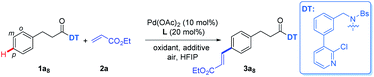
|
Entry |
Oxidant (equiv.) |
Additive (equiv.) |
Yield (%) (p : others) |
1a8 (0.1 mmol), Pd(OAc)2 (0.01 mmol), 2a (2 equiv.), L1 (0.02 mmol), oxidant, additive, HFIP (1.5 mL), 90 °C, air (1 atm), 12 h.
Yield was determined by 1H NMR with CH2Br2 as internal standard; products ratios were determined from crude 1H NMR, and products of others were mainly m- and o-isomers.
L2 (20 mol%).
L3 (20 mol%).
18 h.
2a (3 equiv.), L4 (20 mol%), 60 °C, 48 h.
70 °C.
75 °C.
80 °C.
Isolated yield of desired product in parentheses.
|
1
|
AgOAc (3)
|
— |
90(79)
/15 : 1
|
2c |
AgOAc (3) |
— |
70/11 : 1 |
3d |
AgOAc (3) |
— |
76/12 : 1 |
4e |
Cu(TFA)2 (3) |
— |
21/7 : 1 |
5e |
CuCl2 (3) |
— |
— |
6e |
CuI (3) |
— |
11/5 : 1 |
7e |
Cu(OAc)2 (3) |
— |
40/8 : 1 |
8f |
Cu(OAc)2 (0.5) |
AcOH (2.0) |
68/12 : 1 |
9f,g |
Cu(OAc)2 (0.5) |
AcOH (2.0) |
72/12 : 1 |
10
,
|
Cu(OAc)
2
(0.5)
|
AcOH (2.0)
|
80(72)
/12 : 1
|
11f,i |
Cu(OAc)2 (0.5) |
AcOH (2.0) |
53/12 : 1 |
12f,h |
Cu(OAc)2 (0.5) |
AcOH (1.0) |
76/12 : 1 |
13f,h |
Cu(OAc)2 (0.5) |
AcOH (5.0) |
73/12 : 1 |
|
With the optimized reaction conditions in hand, the generality of this reaction was investigated with a range of phenylpropionic acids as well as some representative olefins (Table 3). It was found that a number of substituents that are electron-donating such as methyl and methoxy groups or electron-withdrawing such as choloro, trifluoromethoxy, trifluoromethyl and fluoro groups at the ortho- or meta-positions were tolerated in this reaction, delivering the target products in generally moderate to good yields with overall high para-selectivity (3a–3j). Notably, several di-substituted substrates were also viable in this reaction to afford generally good yields of desired products (3k–3p). Moreover, substitution at the benzylic position with a methyl (3q) or ethyl (3r) groups was tolerated. Interestingly, para-C–H functionalization of the phenylalanine derivative was also feasible (3s). Finally, a series of electron-deficient alkenes were examined to give generally good yields of the desired products with substrate 1h (3ha–3hi), albeit electron-rich alkenes were not viable.
Table 3 Scope of phenylpropionic acids and alkenesa
Reaction conditions A: 1 (0.1 mmol), Pd(OAc)2 (10 mol%), 2 (3 equiv.), Cu(OAc)2 (50 mol%), L4 (20 mol%), AcOH (2 equiv.), air (1 atm), HFIP (1.5 mL), 75 °C, 48 h.
Conditions B: Oxidant: AgOAc (3 equiv.), ligand: L1 (20 mol%), 24 h; no AcOH added.
2 (2 equiv.), 90 °C.
O2 (1 atm).
2 (3 equiv.), HFIP (1.0 mL), 100 °C.
3ha1 (51%, terminal alkene), 3ha2 (22%, internal alkene). Ratio = p : others. Isolated yield of pure desired para-isomer was reported herein.
|
|
Subsequently, perfluoroalkenes were also evaluated in our study since fluorine-containing compounds play an important role in drug development and material sciences.15 Although meta-C–H perfluoroalkenylation of arenes had been disclosed,16 there was no report of para-C–H perfluoroalkenylation of arenes. We were glad to find that this reaction was compatible with several perfluoroalkenes including brominated perfluoroalkene (Table 4). Phenylpropionic acid derivatives bearing mono-substitution at the benzylic position or on the aryl group were well tolerated to react with tridecafluorooct-1-ene to give desired products with excellent para-selectivity (4a–4h). Di-substituted substrates were also feasible to produce desired products (4i–4l). In addition, other fluorinated alkenes were also evaluated to give moderate to good yields of the target molecules (4m–4p).
Table 4 Reaction with perfluoroalkenesa
Reaction conditions: 1 (0.1 mmol), Pd(OAc)2 (10 mol%), 2 (3 equiv.), Cu(OAc)2 (50 mol%), L4 (20 mol%), AcOH (2 equiv.), HFIP (1.5 mL), air (1 atm), 70 °C, 48 h.
AgOAc (3 equiv.), L1 (20 mol%), 80 °C, 24 h; no AcOH added. Ratio = p : others. Isolated yield of pure desired para-isomer was reported herein.
|
|
The generality of the newly designed pyridine-based para-DT was then tested with other types of molecular structures. Pleasingly, 2-phenyl benzoic acid derivatives bearing several substitution patterns were also smoothly alkenylated at the remote para-position with acrylate or fluorinated alkenes (Table 5, 6a–6i). Moreover, several benzyl alcohol derivatives were also tested, and good yields of desired products could be produced with good to excellent para-selectivity (6j–6p). It should also be mentioned that the scopes of these two types substrates were not as good as that of phenylpropionic acids at current stage.
Table 5 Scope of 2-phenyl benzoic acids and benzyl alcoholsa
Reaction conditions: 5 (0.1 mmol), 2 (3 equiv.), Pd(OAc)2 (10 mol%), L1 (20 mol%), AgOAc (3 equiv.), HFIP (1.0 mL), 100 °C, 24 h.
2 (2 equiv.), HFIP (1.5 mL), 90 °C. Ratio = p : others. Isolated yield of pure desired para-isomer was reported herein.
|
|
Finally, the synthetic potential of our method was investigated briefly. The DT could be readily removed and recycled with excellent yield of para-alkenylated phenylpropionic acid under mild reaction conditions (Scheme 2a). The recovered DT (7) was identical to that synthesized from Suzuki coupling reaction (see ESI†). The reaction was able to be scaled up to 3 mmol to give about one gram of 3h using lower loading of Pd(OAc)2 (5 mol%) (Scheme 2b). In addition, p-phenylenedipropionic acids, which could be synthesized from our para-alkenylated-products after hydrolysis and hydrogenation, were employed to synthesize gelator of nanofiber, including uncommon ones (Scheme 2c).13a
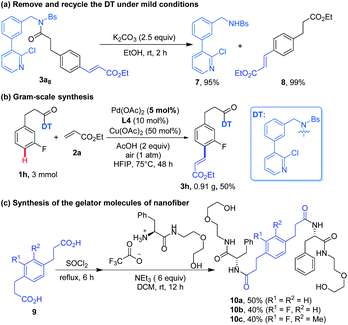 |
| Scheme 2 Synthetic Potential Investigation. | |
Conclusions
In conclusion, we have developed an unprecedented pyridine-based para-DT assisted, Pd-catalyzed para-selective C–H alkenylation of phenylpropanoic acids with a series of alkenes including perfluoroalkenes. This protocol was also compatible with 2-phenyl benzoic acids and benzyl alcohols with high para-selectivity. Notably, air could be used as the terminal oxidant for this reaction and the pyridine-based para-DT could be easily synthesized and readily recycled under mild conditions. It is expected these results would find application in rapid construction of para-substituted arenes and stimulate the exploration of novel methods for para-C–H functionalization of arenes.
Author contributions
X. C. and G. L. conceived the project. X. C. performed the experiments and developed the method. S. F. and M. Z. prepared some of the substrates. S. F., Y. G. and S. L. repeated some of the reactions and checked the data. G. L. directed the project and wrote the manuscript with the feedback from other authors.
Conflicts of interest
There are no conflicts to declare.
Acknowledgements
We gratefully thank the financial supports from NSFC (Grant No. 22022111, 22071248, 21871257), the Natural Science Foundation of Fujian Province (2020J02008), the Strategic Priority Research Program of the Chinese Academy of Sciences (Grant No. XDB20000000), and the Youth Innovation Promotion Association of the Chinese Academy of Sciences (No. 2020306).
Notes and references
- For recent reviews:
(a) T. W. Lyons and M. S. Sanford, Chem. Rev., 2010, 110, 1147 CrossRef CAS;
(b) T. Satoh and M. Miura, Chem.–Eur. J., 2010, 16, 11212 CrossRef CAS;
(c) D. A. Colby, R. G. Bergman and J. A. Ellman, Chem. Rev., 2010, 110, 624 CrossRef CAS;
(d) C. S. Yeung and V. M. Dong, Chem. Rev., 2011, 111, 1215 CrossRef CAS;
(e) B.-J. Li and Z.-J. Shi, Chem. Soc. Rev., 2012, 41, 5588 RSC;
(f) G. Song, F. Wang and X. Li, Chem. Soc. Rev., 2012, 41, 3651 RSC;
(g) K. M. Engle, T.-S. Mei, M. Wasa and J.-Q. Yu, Acc. Chem. Res., 2012, 45, 788 CrossRef CAS;
(h) S. A. Girard, T. Knauber and C.-J. Li, Angew. Chem., Int. Ed., 2014, 53, 74 CrossRef CAS;
(i) O. Daugulis, J. Roane and L. D. Tran, Acc. Chem. Res., 2015, 48, 1053 CrossRef CAS;
(j) Z. Chen, B. Wang, J. Zhang, W. Yu, Z. Liu and Y. Zhang, Org. Chem. Front., 2015, 2, 1107 RSC;
(k) T. Gensch, M. N. Hopkinson, F. Glorius and J. Wencel-Delord, Chem. Soc. Rev., 2016, 45, 2900 RSC;
(l) G. He, B. Wang, W. A. Nack and G. Chen, Acc. Chem. Res., 2016, 49, 635 CrossRef CAS;
(m) R. Shang, L. Ilies and E. Nakamura, Chem. Rev., 2017, 117, 9086 CrossRef CAS;
(n) Y. Park, Y. Kim and S. Chang, Chem. Rev., 2017, 117, 9247 CrossRef CAS;
(o) P. Gandeepan, T. Muller, D. Zell, G. Cera, S. Warratz and L. Ackermann, Chem. Rev., 2019, 119, 2192 CrossRef CAS;
(p) Q. Zhang and B.-F. Shi, Chin. J. Chem., 2019, 37, 647 CrossRef CAS;
(q) Y. Hu and C. Wang, Acta Phys.-Chim. Sin., 2019, 35, 913 Search PubMed;
(r) S. Rej, Y. Ano and N. Chatani, Chem. Rev., 2020, 120, 1788 CrossRef CAS;
(s) A. Trowbridge, S. M. Walton and M. J. Gaunt, Chem. Rev., 2020, 120, 2613 CrossRef CAS;
(t) Y. Qiu, C. Zhu, M. Stangier, J. Struwe and L. Ackermann, CCS, 2020, 2, 1529 Search PubMed.
-
(a) S. R. Neufeldt and M. S. Sanford, Acc. Chem. Res., 2012, 45, 936 CrossRef CAS;
(b) J. Li, S. De Sarkar and L. Ackermann, Top. Organomet. Chem., 2015, 55, 217 CrossRef;
(c) L. Ackermann and J. Li, Nat. Chem., 2015, 7, 686 CrossRef CAS;
(d) C. G. Frost and A. J. Paterson, ACS Cent. Sci., 2015, 1, 418 CrossRef CAS;
(e) J. A. Leitch and C. G. Frost, Chem. Soc. Rev., 2017, 46, 7145 RSC;
(f) M. Font, J. M. Quibell, G. J. P. Perry and I. Larrosa, Chem. Commun., 2017, 53, 5584 RSC;
(g) Y. Gao and G. Li, Aldrichimica Acta, 2017, 50, 61 CAS;
(h) M. T. Mihai, G. R. Genov and R. J. Phipps, Chem. Soc. Rev., 2018, 47, 149 RSC;
(i) C. Haldar and B. Emdadul Chattopadhyay, Tetrahedron
Lett., 2018, 59, 1269 CrossRef CAS;
(j) M. Ghosh and S. De Sarkar, Asian J. Org. Chem., 2018, 7, 1236 CrossRef CAS;
(k) A. Dey, S. K. Sinha, T. K. Achar and D. Maiti, Angew. Chem., Int. Ed., 2019, 58, 10820 CrossRef CAS;
(l) J. Wang and G. Dong, Chem. Rev., 2019, 119, 7478 CrossRef CAS;
(m) G. Meng, N. Y. S. Lam, E. L. Lucas, T. G. Saint-Denis, P. Verma, N. Chekshin and J.-Q. Yu, J. Am. Chem. Soc., 2020, 142, 10571 CrossRef CAS;
(n) G. Liao, Y.-J. Wu and B.-F. Shi, Acta Chim. Sin., 2020, 78, 289 CrossRef.
-
(a) A. Dey, S. Maity and D. Maiti, Chem. Commun., 2016, 52, 12398 RSC;
(b) X. Wang, D. Leow and J.-Q. Yu, J. Am. Chem. Soc., 2011, 133, 13864 CrossRef CAS;
(c) J. Karthikeyan and C. H. Cheng, Angew. Chem., Int. Ed., 2011, 50, 9880 CrossRef CAS;
(d) C. L. Ciana, R. J. Phipps, J. R. Brandt, F. M. Meyer and M. J. Gaunt, Angew. Chem., Int. Ed., 2011, 50, 458 CrossRef CAS;
(e) K. Sun, Y. Li, T. Xiong, J. Zhang and Q. Zhang, J. Am. Chem. Soc., 2011, 133, 1694 CrossRef CAS;
(f) X. C. Cambeiro, T. C. Boorman, P. Lu and I. Larrosa, Angew. Chem., Int. Ed., 2013, 52, 1781 CrossRef CAS;
(g) W. Liu and L. Ackermann, Org. Lett., 2013, 15, 3484 CrossRef CAS;
(h) A. M. Wagner, A. J. Hickman and M. S. Sanford, J. Am. Chem. Soc., 2013, 135, 15710 CrossRef CAS;
(i) Z. Wu, F. Luo, S. Chen, Z. Li, H. Xiang and X. Zhou, Chem. Commun., 2013, 49, 7653 RSC;
(j) Z. Yu, B. Ma, M. Chen, H. H. Wu, L. Liu and J. Zhang, J. Am. Chem. Soc., 2014, 136, 6904 CrossRef CAS;
(k) Y. Saito, Y. Segawa and K. Itami, J. Am. Chem. Soc., 2015, 137, 5193 CrossRef CAS;
(l) N. A. Romero, K. A. Margrey, N. E. Tay and D. A. Nicewicz, Science, 2015, 349, 1326 CrossRef CAS;
(m) G. B. Boursalian, W. S. Ham, A. R. Mazzotti and T. Ritter, Nat. Chem., 2016, 8, 810 CrossRef CAS;
(n) Y. Ma, B. Wang, L. Zhang and Z. Hou, J. Am. Chem. Soc., 2016, 138, 3663 CrossRef CAS;
(o) K. Naksomboon, J. Poater, F. M. Bickelhaupt and M. A. Fernandez-Ibanez, J. Am. Chem. Soc., 2019, 14, 6719 CrossRef;
(p) K. Liu, S. Tang, T. Wu, S. Wang, M. Zou, H. Cong and A. Lei, Nat. Commun., 2019, 10, 639 CrossRef;
(q) F. Berger, M. B. Plutschack, J. Riegger, W. Yu, S. Speicher, M. Ho, N. Frank and T. Ritter, Nature, 2019, 567, 223 CrossRef CAS;
(r) S. C. Fosu, C. M. Hambira, A. D. Chen, J. R. Fuchs and D. A. Nagib, Chem, 2019, 5, 417 CrossRef CAS;
(s) F. de Azambuja, M. H. Yang, T. Feoktistova, M. Selvaraju, A. C. Brueckner, M. A. Grove, S. Koley, P. H. Cheong and R. A. Altman, Nat. Chem., 2020, 12, 489 CrossRef CAS.
-
(a) H. Xu, M. Shang, H. X. Dai and J.-Q. Yu, Org. Lett., 2015, 17, 3830 CrossRef CAS;
(b) Y. X. Luan, T. Zhang, W. W. Yao, K. Lu, L. Y. Kong, Y. T. Lin and M. Ye, J. Am. Chem. Soc., 2017, 139, 1786 CrossRef CAS.
-
(a) Y. Nakao, Y. Yamada, N. Kashihara and T. Hiyama, J. Am. Chem. Soc., 2010, 132, 13666 CrossRef CAS;
(b) S. Okumura, S. Tang, T. Saito, K. Semba, S. Sakaki and Y. Nakao, J. Am. Chem. Soc., 2016, 138, 14699 CrossRef CAS;
(c) S. Okumura and Y. Nakao, Org. Lett., 2017, 19, 584 CrossRef CAS;
(d) L. Yang, K. Semba and Y. Nakao, Angew. Chem., Int. Ed., 2017, 56, 4853 CrossRef CAS.
- M. E. Hoque, R. Bisht, C. Haldar and B. Chattopadhyay, J. Am. Chem. Soc., 2017, 139, 7745 CrossRef CAS.
-
(a) M. T. Mihai, B. D. Williams and R. J. Phipps, J. Am. Chem. Soc., 2019, 141, 15477 CrossRef CAS;
(b) J. R. Montero Bastidas, T. J. Oleskey, S. L. Miller, M. R. Smith 3rd and R. E. Maleczka Jr, J. Am. Chem. Soc., 2019, 141, 15483 CrossRef CAS.
-
(a) X. Guo and C.-J. Li, Org. Lett., 2011, 13, 4977 CrossRef CAS;
(b) J. A. Leitch, C. L. McMullin, A. J. Paterson, M. F. Mahon, Y. Bhonoah and C. G. Frost, Angew. Chem., Int. Ed., 2017, 56, 15131 CrossRef CAS;
(c) C. Yuan, L. Zhu, R. Zeng, Y. Lan and Y. Zhao, Angew. Chem., Int. Ed., 2018, 57, 1277 CrossRef CAS;
(d) G. Tu, C. Yuan, Y. Li, J. Zhang and Y. Zhao, Angew. Chem., Int. Ed., 2018, 57, 15597 CrossRef CAS;
(e) C. Yuan, L. Zhu, C. Chen, X. Chen, Y. Yang, Y. Lan and Y. Zhao, Nat. Commun., 2018, 9, 1189 CrossRef;
(f) Z. Jiao, L. H. Lim, H. Hirao and J. S. Zhou, Angew. Chem., Int. Ed., 2018, 57, 6294 CrossRef CAS;
(g) P. Liu, C. Chen, X. Cong, J. Tang and X. Zeng, Nat. Commun., 2018, 9, 4637 CrossRef;
(h) K. D. Mane, A. Mukherjee, K. Vanka and G. Suryavanshi, J. Org. Chem., 2019, 84, 2039 CrossRef CAS;
(i) W. T. Fan, Y. Li, D. Wang, S. J. Ji and Y. Zhao, J. Am. Chem. Soc., 2020, 142, 20524 CrossRef CAS;
(j) W. K. Tang, F. Tang, J. Xu, Q. Zhang, J. J. Dai, Y. S. Feng and H. J. Xu, Chem. Commun., 2020, 56, 1497 RSC;
(k) J. Zhou, F. Wang, Z. Lin, C. Cheng, Q. Zhang and J. Li, Org. Lett., 2020, 22, 68 CrossRef CAS.
-
(a) S. Bag, T. Patra, A. Modak, A. Deb, S. Maity, U. Dutta, A. Dey, R. Kancherla, A. Maji, A. Hazra, M. Bera and D. Maiti, J. Am. Chem. Soc., 2015, 137, 11888 CrossRef CAS;
(b) T. Patra, S. Bag, R. Kancherla, A. Mondal, A. Dey, S. Pimparkar, S. Agasti, A. Modak and D. Maiti, Angew. Chem., Int. Ed., 2016, 55, 7751 CrossRef CAS;
(c) A. Maji, S. Guin, S. Feng, A. Dahiya, V. K. Singh, P. Liu and D. Maiti, Angew. Chem., Int. Ed., 2017, 56, 14903 CrossRef CAS;
(d) A. Maji, A. Dahiya, G. Lu, T. Bhattacharya, M. Brochetta, P. Liu, G. Zanoni and D. Maiti, Nat. Commun., 2018, 9, 3582 CrossRef;
(e) U. Dutta, S. Maiti, S. Pimparkar, S. Maiti, L. R. Gahan, E. H. Krenske, D. W. Lupton and D. Maiti, Chem. Sci., 2019, 10, 7426 RSC;
(f) S. Pimparkar, T. Bhattacharya, A. Maji, A. Saha, R. Jayarajan, U. Dutta, G. Lu, D. W. Lupton and D. Maiti, Chem.–Eur. J., 2020, 26, 11558 CrossRef CAS.
- M. Li, M. Shang, H. Xu, X. Wang, H.-X. Dai and J.-Q. Yu, Org. Lett., 2019, 21, 540 CrossRef CAS.
-
(a) H. Shi, Y. Lu, J. Weng, K. L. Bay, X. Chen, K. Tanaka, P. Verma, K. N. Houk and J.-Q. Yu, Nat. Chem., 2020, 12, 399 CrossRef CAS;
(b) U. Dutta, S. Porey, S. Pimparkar, A. Mandal, J. Grover, A. Koodan and D. Maiti, Angew. Chem., Int. Ed., 2020, 59, 20831 CrossRef CAS.
-
(a) L. Chu, M. Shang, K. Tanaka, Q. Chen, N. Pissarnitski, E. Streckfuss and J.-Q. Yu, ACS Cent. Sci., 2015, 1, 394 CrossRef CAS;
(b) Z. Jin, L. Chu, Y. Q. Chen and J.-Q. Yu, Org. Lett., 2018, 20, 425 CrossRef CAS;
(c) H. Xu, M. Liu, L. J. Li, Y. F. Cao, J.-Q. Yu and H. X. Dai, Org. Lett., 2019, 21, 4887 CrossRef CAS;
(d) M. Liu, L.-J. Li, J. Zhang, H. Xu and H.-X. Dai, Chin. Chem. Lett., 2020, 31, 1301 CrossRef CAS; For pyrimidine-based meta-DT:
(e) S. Bag, R. Jayarajan, R. Mondal and D. Maiti, Angew. Chem., Int. Ed., 2017, 56, 3182 CrossRef CAS;
(f) R. Jayarajan, J. Das, S. Bag, R. Chowdhury and D. Maiti, Angew. Chem., Int. Ed., 2018, 57, 7659 CrossRef CAS;
(g) S. Fang, X. Wang, F. Yin, P. Cai, H. Yang and L. Kong, Org. Lett., 2019, 21, 1841 CrossRef CAS;
(h) B. Jiao, Z. Peng, Z.-H. Dai, L. Li, H. Wang and M.-D. Zhou, Eur. J. Org. Chem., 2019, 2019, 3195 CrossRef CAS.
-
(a) J. Liu, F. Yuan, X. Ma, D. Y. Auphedeous, C. Zhao, C. Liu, C. Shen and C.-L. Feng, Angew. Chem., Int. Ed., 2018, 57, 6475 CrossRef CAS;
(b) X. Wang, H. Alshammary, R. Zhang, A. Seifpour, J. T. Villalva, Z. Xu, C. Zheng, J. Li and X.-Y. Huang, Polyhedron, 2008, 27, 3439 CrossRef CAS;
(c) S. Tanaka, A. Yagyu, M. Kikugawa, M. Ohashi, T. Yamagata and K. Mashima, Chem.–Eur. J., 2011, 17, 3693 CrossRef CAS;
(d) S. Sanram, J. Boonmak and S. Youngme, Polyhedron, 2016, 119, 151 CrossRef CAS;
(e) S. Roelens and R. Torriti, J. Am. Chem. Soc., 1998, 120, 12443 CrossRef CAS.
- S. Li, H. Wang, Y. Weng and G. Li, Angew. Chem., Int. Ed., 2019, 58, 18502 CrossRef CAS.
-
(a) K. Muller, C. Faeh and F. Diederich, Science, 2007, 317, 1881 CrossRef;
(b) R. Berger, G. Resnati, P. Metrangolo, E. Weber and J. Hulliger, Chem. Soc. Rev., 2011, 40, 3496 RSC;
(c) J. Wang, M. Sanchez-Rosello, J. L. Acena, C. del Pozo, A. E. Sorochinsky, S. Fustero, V. A. Soloshonok and H. Liu, Chem. Rev., 2014, 114, 2432 CrossRef CAS.
- M. Brochetta, T. Borsari, S. Bag, S. Jana, S. Maiti, A. Porta, D. B. Werz, G. Zanoni and D. Maiti, Chem.–Eur. J., 2019, 25, 10323 CrossRef CAS.
Footnote |
† Electronic supplementary information (ESI) available: Experimental procedures and data for new compounds. See DOI: 10.1039/d0sc07042d |
|
This journal is © The Royal Society of Chemistry 2021 |
Click here to see how this site uses Cookies. View our privacy policy here.