DOI:
10.1039/D1RA08268J
(Paper)
RSC Adv., 2021,
11, 39518-39522
Cp2ZrCl2 – Et3Al reagent system in the homo-coupling of trimethylsilyl-substituted alkynes†
Received
11th November 2021
, Accepted 15th November 2021
First published on 13th December 2021
Abstract
The reaction of trimethylsilyl-substituted alkynes with 0.5 equivalents of Cp2ZrCl2 and 1 equivalent of Et3Al in toluene at room temperature for 18 hours gives, after hydrolysis/deuterolysis or iodination, functionalized products of the homo-coupling of silyl-substituted alkynes in good yield. Trimethylsilyl-substituted α,ω-diynes react with the Cp2ZrCl2 – Et3Al reagent system to give (1Z,2Z)-1,2-bis(iodo(trimethylsilyl)methylene)cycloalkanes after iodinolysis.
1 Introduction
The reaction of homo-coupling of alkynes using zirconocene reagents is an important basis for the efficient synthesis of polymers, oligomers and macrocycles.1 It is generally accepted that the transformation takes place through the intermediate formation of “Cp2Zr” species that can be generated by the interaction of Cp2ZrCl2 with alkali and alkaline earth metals, as well as with alkyl derivatives of metals. The most widely used reagents include the Negishi reagent (“Cp2ZrBu2”),2,3 Takahashi reagent (“Cp2ZrEt2”),4 and Rosenthal reagent (Cp2Zr(py)TMSC
CTMS).5–7 Despite detailed studies of the reaction of Et3Al with Cp2ZrCl2,8–12 the resulting zirconocene complexes could not be successfully used for intramolecular coupling of alkynes. Negishi has demonstrated that the reaction of diphenylacetylene with Et3Al in the presence of 10 mol% of Cp2ZrCl2 in benzene at 55 °C gave a mixture of substituted alkene and diene in a 1
:
1 ratio with a total yield of 90%.13 However, the reaction with 5-decyne under similar conditions resulted in the formation of only 5-ethyl-5-decene. By replacing Et3Al with Pr3Al, it was possible to convert 5-decyne into tetrasubstituted 1,3-diene in 38% yield with simultaneous formation of reduction products in the amount of 63%.14 Thus, attempts to develop a synthetically useful methodology for the coupling of alkynes using trialkylaluminums and Cp2ZrCl2 were unsuccessful. However, from our early observations of the alkyne cycloalumination reaction with Et3Al in the presence of catalytic amounts of Cp2ZrCl2, we noticed that trimethylsilyl substituted 1-hexyne under the reaction conditions gave 1,4-diene hydrocarbons as a by-product in up to 20% yield. This gave hope for the possibility of selecting the optimal conditions for the implementation of the homo-coupling reaction of silicon containing alkynes. The importance of this transformation was due to the possibility of its use for the preparation of macrocyclic zirconacyclopentadiene compounds through the combination of diyne molecules of the TMS-C
C-R-C
C-TMS type.
2 Results and discussion
It was found that the reaction of silyl-substituted alkynes with 1 equivalent of Cp2ZrCl2 and 2 equivalents of Et3Al in toluene at 23 °C for 18 hours after hydrolysis/deuterolysis or iodinolysis gives homo-coupling products 2–4 in good yield (Table 1). A remarkable feature of the reaction is the complete absence of products of cyclic carbometallation 5. In the case of 1-alkyl-2-(trimethylsilyl)acetylenes, the reaction proceeds with a high regioselectivity to give 3,4-dialkyl substituted 1,4-bis(trimethylsilyl)buta-1,3-diene. However, the reaction with trimethyl(phenylethynyl)silane under similar conditions gives a mixture ((1E,3E)-2,3-diphenylbuta-1,3-diene-1,4-diyl)bis(trimethylsilane) 2e and ((1E,3Z)-2,4-diphenylbuta-1,3-diene-1,3-diyl)bis(trimethylsilane) 2e′ in a 5
:
1 ratio. The reaction of trimethylsilyl-substituted α,ω-diynes (1,7-octadiyne, 1,8-nonadiyne) with Cp2ZrCl2 – Et3Al reagent system after iodinolysis gives (1Z,2Z)-1,2-bis(iodo(trimethylsilyl)methylene)cycloalkanes 6 and 7 in good yield (Scheme 1). We were unable to obtain the products of the homo-coupling of alkynes in the case of 5-decyne and hex-1-yn-1-ylbenzene. As a result of the reaction, these alkynes gave after deuterolysis only products of cyclic carbometallation 5f and 5g. We failed to involve in the reaction acetylene derivatives of more sterically hindered silanes: hex-1-yn-1-yltriisopropylsilane, tert-butyl(hex-1-yn-1-yl)dimethylsilane and 1,2-bis(trimethylsilyl)ethyne. The above-mentioned acetylenic compounds were inert under the reaction conditions.
Table 1 The homo-coupling of silyl-substituted alkynes by Cp2ZrCl2 – Et3Al reagent
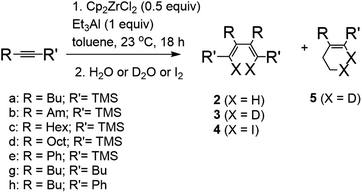
|
Entry |
R |
R′ |
X |

|

|
1 |
Bu |
TMS |
H |
86% (2a) |
nd |
2 |
Bu |
TMS |
I |
88% (4a) |
nd |
3 |
Am |
TMS |
H |
83% (2b) |
nd |
4 |
Am |
TMS |
D |
85% (3b) |
nd |
5 |
Am |
TMS |
I |
69% (4b) |
nd |
6 |
Hex |
TMS |
H |
78% (2c) |
nd |
7 |
Oct |
TMS |
H |
84% (2d) |
nd |
8 |
Oct |
TMS |
I |
69% (4d) |
nd |
9 |
Ph |
TMS |
H |
61% (2e) + 12% (2e′) |
nd |
10 |
Bu |
Bu |
D |
nd |
82% (5f) |
11 |
Bu |
Ph |
D |
nd |
69% (5g) |
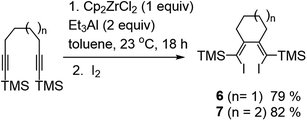 |
| Scheme 1 The reaction of trimethylsilyl-substituted α,ω-diynes with Cp2ZrCl2 – Et3Al reagent system. | |
The solvent has a significant effect on the reaction. When replacing toluene with hexane, in the case of a reaction with hept-1-yn-1-yltrimethylsilane, we obtained after deuterolysis the mixture of the compound 3b in 60% yield with the product of cyclic carbometallation 5b in 20% yield. In diethyl ether and diisopropyl ether, the reaction does not take place.
We assume that the reaction proceeds through the stage of intermediate formation of the metalcyclopentadiene, which can be of a ziconocene or organoaluminum nature. The formation of a non-cyclic bimetallic intermediate is not excluded. Due to the high reactivity of organoaluminum compounds in the reaction mixture with respect to oxygen, the isolation and identification of this metalcyclopentadiene is difficult. Scheme 2 depicts the proposed mechanism for the formation of zirconacyclopentadienes and zirconacyclopentenes under the reaction conditions. Intermediate A, formed by mixing 1 equivalent of Cp2ZrCl2 and 2 equivalents of Et3Al, was previously well characterized.5–9
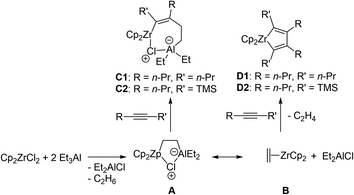 |
| Scheme 2 The proposed mechanism of the formation of zirconacyclopentadienes. | |
Scheme 2 depicts the proposed mechanism of the reaction. Intermediate A, formed by mixing 1 equivalent of Cp2ZrCl2 and 2 equivalents of Et3Al, was previously well characterized.8–12 The further reaction of intermediate A with dialkyl substituted alkynes proceeds as an alkyne insertion as it was describes by Negishi.13 It can be assumed that the insertion of trialkylsilyl-substituted acetylenes is more difficult, since the rate of cycloalumination of trialkylsilyl-substituted acetylenes is lower compared to dialkyl-substituted acetylenes.14 According to quantum chemical calculations at B3LYP/6-31G*/LANL2DZ level of theory in gas phase, the standard Gibbs energy of the insertion reaction is lower in the case of 4-octyne (A→C1) by 11.6 kcal mol−1 compared to the insertion of trimethyl(pent-1-yn-1-yl)silane (A→C2). At the same time, according to the calculations, the formation of zirconacyclopentadiene D from intermediate A is thermodynamically more efficient process in the case of 4-octyne (A→D1) rather than trimethyl(pent-1-yn-1-yl)silane (A→D2). The standard Gibbs energies of the corresponding hypothetical reactions are −9.4 and 8.9 kcal mol−1, respectively. Thus, there are no kinetic or thermodynamic grounds for the predominant formation of metallacyclopentadiene intermediates in the case of trimethylsilyl-substituted acetylenes. Then we assumed that there is an equilibrium between intermediate A and zirconocene–ethylene complex B. We have already postulated the existence of such an equilibrium in the cycloalumination of 1-alkynylphosphines15 and olefins.16 The reaction of alkynes with intermediate B can be responsible for the formation of zirconacyclopentadiene intermediates. The following hypothesis was proposed to explain experimental results: If alkyne is sufficiently reactive for the insertion into Zr–C bond of intermediate A then it will be consumed mainly for the formation of the product of cyclic carbometallation, or else alkyne will react with the zirconocene–ethylene complex to give homo-coupling product. In the case of trimethylsilyl-substituted acetylenes, bulky trimethylsilyl group humpers the insertion of alkyne into Zr–C bond of intermediate A and the formation of homo-coupling product is observed. However, when carrying out the reaction with hept-1-yn-1-yltrimethylsilane in hexane instead of toluene, we observed the side formation of the product of cyclic carbometallation 5b, which should be formed as a result of the interaction of intermediate A with alkyne. This fact can also be explained within the framework of the proposed hypothesis. The point is that the use of a less polar solvent decreases the degree of solvation, increasing the effective charges of the atoms of the reaction center in intermediate A, which in turn increases the probability of the insertion of trimethylsilyl-substituted acetylene into the Zr–C bond to give the product of cyclic carbometallation after hydrolysis.
3 Conclusions
It was found that Cp2ZrCl2 – Et3Al reagent system in toluene allows to prepare the products of the homo-coupling of silyl-substituted alkynes in good yield.
4 Experimental section
4.1. General data
The reagents were obtained from Sigma-Aldrich or Acros. Toluene was distilled over Na. Silyl-substituted alkynes were prepared by the reaction of terminal acetylenes with EtMgBr and trialkylchlorosilanes.17 IR spectra were recorded on Bruker VE Vertex 70v spectrometer as liquid films or in Nujol and are reported in wavenumbers (cm−1). Nuclear magnetic resonance spectroscopy was performed on a Brucker Avance 500. The 1H NMR spectra were recorded at 500 MHz and 13C-{1H} NMR spectra at 125 MHz in CDCl3. The chemical shifts are reported in ppm relative to tetramethylsilane (TMS) as the internal standard. The numbering of atoms in the 13C and 1H NMR spectra of the compounds 2a-e, 3b, 4a, 4b, 4d, 6, 7 is shown in Fig. 1. Mass spectra were obtained on a Finnigan 4021 instrument. All quantum-chemical calculations were performed using B3LYP/6-31G(d)/LanL2DZ basis set as implemented in Gaussian 09 software.18
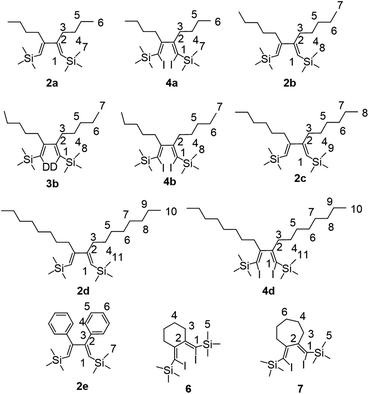 |
| Fig. 1 The numbering of atoms in the reported 13C- and 1H-NMR spectral data of the compounds 2a-e, 3b, 4a, 4b, 4d, 6, 7. | |
4.2. Homo-coupling of trimethylsilyl-substituted alkynes by Cp2ZrCl2 – Et3Al reagent
A suspension of Cp2ZrCl2 (292 mg, 1.00 mmol) in toluene (3 mL) in a 25 mL round bottom flask was cooled with an ice-bath and then Et3Al (0.3 mL, 2.00 mmol) was added. After stirring the mixture at 0 °C for 30 minutes, 1 mmol of trimethylsilyl-substituted alkyne or 0.5 mmol of trimethylsilyl-substituted α,ω-diyne was added. The mixture was stirred at 23 °C for 18 h. Then the mixture was diluted with hexane (5 mL) and H2O (3 mL) (to prepare 2a-e) or D2O (3 mL) (to prepare 3b) was added dropwise while cooling the reactor flask with an ice-bath. The precipitate was filtered on a filter paper. To prepare the compounds 4a, 4b, 4d, a solution of I2 (787.5 mg, 6.25 mmol) in THF (5 mL) was added to the reaction mixture while cooling the reactor flask with an ice-bath and stirred at 23 °C for 1 h. To prepare the compounds 6, 7, a solution of I2 (787.5 mg, 6.25 mmol) in THF (5 mL) was used. The aqueous layer was extracted with diethyl ether (3 × 5 mL). The combined organic layers were washed with brine (10 mL), dried over anhydrous CaCl2. Evaporation of solvent and purification of the residue by column chromatography (hexane) gave a colourless oil.
4.2.1. ((1E,3E)-2,3-Dibutylbuta-1,3-diene-1,4-diyl)bis(trimethylsilane) (2a)19. Yield: 133 mg (86%); Rf = 0.8 (hexane). IR (liquid film): 2956, 2873, 2861, 1593, 1561, 1465, 1248, 839, 769, 689, 619 cm−1. 1H NMR (500 MHz, CDCl3): δ = 0.14 (s, 9H, C(7)H3), 0.92 (t, J = 7.0 Hz, 3H, C(6)H3), 1.28–1.36 (m, 4H, C(4, 5)H2), 2.29 (t, J = 6.4 Hz, 2H, C(3)H2), 5.48 (s, 1H, C(1)H). 13C-{1H} NMR (500 MHz, CDCl3): δ = 0.36 (C(7)), 14.06 (C(6)), 22.93 (C(5)), 31.69 (C(4)), 33.74 (C(3)), 125.07 (C(1)), 160.67 (C(2)). 29Si-{1H} (500 MHz, CDCl3): −10.79. MS (EI): m/z, % = 311 (3) [M+], 295 (5), 268 (9), 237 (15), 207 (10), 165 (7), 138 (6), 73 (100), 45 (9).
4.2.2. ((1Z,3Z)-2,3-Dibutyl-1,4-diiodobuta-1,3-diene-1,4-diyl)bis(trimethylsilane) (4a)20. Yield: 247 mg (88%); Rf = 0.6 (hexane). 1H NMR (500 MHz, CDCl3): δ = 0.36 (s, 9H, C(7)H3), 0.94 (t, J = 7.3 Hz, 1H, C(6)H3), 1.34 (q, J = 7.3 Hz, J = 14.5 Hz, 2H, C(5)H2), 1.46–1.55 (m, 1H(A), 1H(B), C(4)H2), 2.21 (t, J = 13.7 Hz, 1H(A), C(3)H2), 2.52 (t, J = 13.7 Hz, 1H(B), C(3)H3). 13C-{1H} NMR (500 MHz, CDCl3): δ = 1.89 (C(7)), 13.83 (C(6)), 23.26 (C(5)), 30.84 (C(4)), 36.25 (C(3)), 108.67 (C(1)), 163.54 (C(2)). MS (EI): m/z, % = 562 (<1) [M+], 435 (84), 185 (14), 161 (26), 139 (7), 73 (100), 45 (13).
4.2.3. ((1E,3E)-2,3-Dipentylbuta-1,3-diene-1,4-diyl)bis(trimethylsilane) (2b). Yield: 141 mg (83%); Rf = 0.8 (hexane). IR (liquid film): 2956, 2926, 2856, 1593, 1560, 1466, 1248, 839, 772, 689, 620 cm−1. 1H NMR (500 MHz, CDCl3): δ = 0.14 (s, 9H, C(8)H3), 0.91 (t, J = 7.0 Hz, 3H, C(7)H3), 1.28–1.35 (m, 6H, C(4, 5, 6)H2), 2.29 (t, J = 6.0 Hz, 2H, C(3)H2), 5.48 (s, 1H, C(1)H). 13C-{1H} NMR (500 MHz, CDCl3): δ = 0.35 (C(8)), 14.03 (C(7)), 22.54 (C(6)), 29.13 (C(4)), 32.03 (C(5)), 33.95 (C(3)), 125.10 (C(1)), 160.69 (C(2)). 29Si-{1H} (500 MHz, CDCl3): −10.82. MS (EI): m/z, % = 339 (4) [M+], 338 (11), 282 (15), 265 (30), 209 (8), 191 (10), 73 (100), 45 (8). C20H40D2Si2 calc. C 70.92, H 12.50; CHN analysis, C 70.4, H 12.8.
4.2.4. ((1E,3E)-2,3-Dipentylbuta-1,3-diene-1,4-diyl-1,4-d2)bis(trimethylsilane) (3b). Yield: 145 mg (85%); Rf = 0.8 (hexane). IR (liquid film): 2956, 2928, 2859, 1589, 1556, 1466, 1379, 1248, 1097, 1042, 837, 761, 689, 616 cm−1. 1H NMR (500 MHz, CDCl3): δ = 0.14 (s, 9H, C(8)H3), 0.91 (t, J = 6.7 Hz, 3H, C(7)H3), 1.28–1.33 (m, 6H, C(4, 5, 6)H2), 2.28 (t, J = 7.4 Hz, 2H, C(3)H2), 5.48 (s, 1H, C(1)H). 13C-{1H} NMR (500 MHz, CDCl3): δ = 0.35 (C(8)), 14.04 (C(7)), 22.54 (C(6)), 29.14 (C(4)), 32.03 (C(5)), 32.95 (C(3)), 125.09 (C(1)), 160.60 (C(2)). 29Si-{1H} (500 MHz, CDCl3): −10.84. MS (EI): m/z, % = 341 (<1) [M+], 339 (4), 283 (5), 266 (10), 210 (4), 192 (5), 73 (100), 45 (6). C20H42Si2 calc. C 70.50; CHN analysis, C 70.1.
4.2.5. ((1Z,3Z)-1,4-Diiodo-2,3-dipentylbuta-1,3-diene-1,4-diyl)bis(trimethylsilane) (4b). Yield: 204 mg (69%); Rf = 0.7 (hexane). IR (liquid film): 2956, 2930, 2873, 1970, 1462, 1249, 1116, 841, 759, 693, 588 cm−1. 1H NMR (500 MHz, CDCl3): δ = 0.36 (s, 9H, C(8)H3), 0.92 (t, J = 7.1 Hz, 3H, C(7)H3), 1.28–1.31 (m, 2H, C(5)H2), 1.33–1.37 (m, 2H, C(6)H2), 1.50–1.56 (m, 1H(A), C(4)H2), 1.62–1.67 (m, 1H(B), C(4)H2), 2.22 (t, J = 13.2 Hz, 1H(A), C(3)H2), 2.50 (t, J = 13.3 Hz, 1H(B), C(3)H2). 13C-{1H} NMR (500 MHz, CDCl3): δ = 1.88 (C(8)), 14.04 (C(7)), 22.38 (C(6)), 28.50 (C(4)), 32.35 (C(5)), 36.49 (C(3)), 108.58 (C(1)), 163.68 (C(2)). 29Si-{1H} (500 MHz, CDCl3): 0.93. MS (EI): m/z, % = 591 (<1) [M+], 463 (13), 189 (10), 95 (3), 73 (100), 45 (4). C20H40I2Si2 calc. C 40.68, H 6.83; CHN analysis, C 40.5, H 6.6.
4.2.6. ((1E,3E)-2,3-Dihexylbuta-1,3-diene-1,4-diyl)bis(trimethylsilane) (2c)19. Yield: 143 mg (78%); Rf = 0.7 (hexane). IR (liquid film): 2956, 2927, 2857, 1592, 1561, 1466, 839, 771, 749, 689, 619 cm−1. 1H NMR (50 MHz, CDCl3): δ = 0.14 (s, 9H, C(9)H3), 0.91 (t, J = 6.9 Hz, 3H, C(8)H3), 1.28–1.33 (m, 8H, C(4, 5, 6, 7)H2), 2.29 (t, J = 7.3 Hz, 2H, C(3)H2), 5.48 (s, 1H, C(1)H). 13C-{1H} NMR (500 MHz, CDCl3): δ = 0.35 (C(9)), 14.06 (C(8)), 22.59 (C(7)), 29.40 (C(4)), 29.47 (C(5)), 31.73 (C(6)), 34.00 (C(3)), 125.10 (C(1)), 160.71 (C(2)). 29Si-{1H} (500 MHz, CDCl3): −10.84. MS (EI): m/z, % = 367 (2) [M+], 293 (14), 263 (5), 219 (6), 138 (4), 73 (100), 45 (6).
4.2.7. ((1E,3E)-2,3-Dioctylbuta-1,3-diene-1,4-diyl)bis(trimethylsilane) (2d). Yield: 186 mg (84%); Rf = 0.7 (hexane). IR (liquid film): 2955, 2926, 2855, 1736, 1465, 1378, 1248, 840, 722, 690, 620 cm−1. 1H NMR (500 MHz, CDCl3): δ = 0.14 (s, 9H, C(11)H3), 0.91 (t, J = 7.2 Hz, 3H, C(10)H3), 1.29 (s, 12H, C(4–9)H2), 2.29 (t, J = 7.8 Hz, 2H, C(3)H2), 5.48 (s, 1H, C(1)H). 13C-{1H} NMR (500 MHz, CDCl3): δ = 0.36 (C(11)), 14.12 (C(10)), 22.69 (C(9)), 29.26 (C(4)), 29.44 (C(5)), 29.49 (C(7)), 29.80 (C(6)), 31.89 (C(8)), 33.99 (C(3)), 125.08 (C(1)), 160.71 (C(2)). 29Si-{1H} (500 MHz, CDCl3): −10.82. MS (EI): m/z, % = 423 (6) [M+], 422 (<1), 349 (15), 324 (9), 275 (6), 251 (7), 226 (5), 73 (100), 45 (3). C26H54Si2 calc. C 73.85, H 12.87; CHN analysis, C 74.3, H 12.5.
4.2.8. ((1Z,3Z)-1,4-Diiodo-2,3-dioctylbuta-1,3-diene-1,4-diyl)bis(trimethylsilane) (4d). Yield: 233 mg (69%); Rf = 0.7 (hexane). 1H NMR (500 MHz, CDCl3): δ = 0.36 (s, 9H, C(11)H3), 0.91 (t, J = 6.9 Hz, 3H, C(10)H3), 1.29 (s, 8H, C(5,7–9)H2), 1.51–1.56 (m, 2H, C(6)H2), 1.51–1.56 (m, 1H(A), C(4)H2), 1.61–1.66 (m, 1H(B), C(4)H2), 2.22 (t, J = 0.0 Hz, 1H(A), C(3)H2), 2.47–2.54 (m, 1H(B), C(3)H2). 13C-{1H} NMR (500 MHz, CDCl3): δ = 1.88 (C(11)), 14.12 (C(10)), 22.68 (C(9)), 28.83 (C(4)), 29.26 (C(5)), 29.28 (C(7)), 30.18 (C(6)), 31.84 (C(8)), 36.54 (C(3)), 100.56 (C(1)), 163.72 (C(2)). MS (EI): m/z, % = 675 (<1) [M+], 547 (7), 273 (11), 207 (100), 191 (11), 133 (9), 96 (16), 73 (88), 40 (13). C26H52I2Si2 calc. C 46.29, H 7.77; CHN analysis, C 46.5, H 7.7.
4.2.9. ((1E,3E)-2,3-Diphenylbuta-1,3-diene-1,4-diyl)bis(trimethylsilane) (2e)21. Yield: 106 mg (61%); Rf = 0.7 (hexane). 1H NMR (500 MHz, CDCl3): δ = −0.28 (s, 9H, C(7)H3), 5.48 (s, 1H, C(1)H), 7.22 (d, J = 6.9 Hz, 1H, C(4)H), 7.31–7.38 (m, 2H, C(5,6)H). 13C-{1H} NMR (500 MHz, CDCl3): δ = −0.28 (C(7)), 126.88 (C(6)), 127.57 (C(5)), 129.91 (C(4)), 133.90 (C(1)), 142.16 (C(3)), 159.87 (C(2)). MS (EI): m/z, % = 351 (7) [M+], 350 (20), 335 (7), 276 (13), 262 (21), 247 (28), 135 (18), 73 (100), 45 (12).
4.2.10. (1Z,2Z)-1,2-Bis(iodo(trimethylsilyl)methylene)cyclohexane (6)22. Yield: 199 mg (79%); Rf = 0.6 (hexane). IR (liquid film): 2953, 2930, 2896, 2854, 1567, 1460, 1442, 1421, 1406, 1250, 1075, 963, 936, 842, 758, 692, 627, 499 cm−1. 1H NMR (500 MHz, CDCl3): δ = 0.33 (s, 9H, C(5)H3), 1.52 (t, J = 9.2 Hz, 1H(A), C(4)H3), 1.92 (s, 1H(B), C(4)H2), 2.21 (t, J = 12.0 Hz, 1H(A), C(3)H2), 2.88 (d, J = 12.0 Hz, 1H(B), C(3)H2). 13C-{1H} NMR (500 MHz, CDCl3): δ = 1.74 (C(5)), 29.13 (C(4)), 36.42 (C(3)), 101.65 (C(1)), 165.99 (C(2)). 29Si-{1H} (500 MHz, CDCl3): 0.95. MS (EI): m/z, % = 504 (<1) [M+], 377 (59), 289 (16), 161 (20), 97 (13), 73 (100), 45 (12).
4.2.11. (1Z,2Z)-1,2-Bis(iodo(trimethylsilyl)methylene)cycloheptane (7). Yield: 279 mg (82%); Rf = 0.6 (hexane). IR (liquid film): 2952, 2926, 2852, 1557, 1442, 1408, 1249, 1182, 1038, 899, 865, 839, 758, 693, 627, 480 cm−1. 1H NMR (500 MHz, CDCl3): δ = 0.34 (s, 9H, C(5)H3), 1.47–1.52 (m, 2H(A), C(4,6)H3), 1.56–1.63 (m, 2H(B), C(4,6)H2), 2.39–2.47 (m, 1H(A), C(3)H2), 2.62–2.68 (m, 1H(B), C(3)H2). 13C-{1H} NMR (500 MHz, CDCl3): δ = 1.64 (C(5)), 26.91 (C(6)), 28.31 (C(4)), 34.26 (C(3)), 104.51 (C(1)), 165.88 (C(2)). MS (EI): m/z, % = 518 (1). 29Si-{1H} (500 MHz, CDCl3): 0.96. [M+], 391 (54), 358 (4), 303 (8), 175 (17), 131 (17), 97 (20), 73 (100), 40 (55). C15H28I2Si2 calc. C 34.76, H 5.44; CHN analysis, C 35.1, H 5.7.
Conflicts of interest
The authors declare no competing financial interest.
Acknowledgements
The study was supported by a grant from the Russian Science Foundation (project No. 19-73-20128). The synthesis of the starting substrates – 1-alkynylsilanes was carried out within the RF state assignment, reg. no. AAAA-A19-119022290009-3.
References
- V. H. Gessner, J. F. Tannaci, A. D. Miller and T. D. Tilley, Assembly of macrocycles by zirconocene-mediated, reversible carbon-carbon bond formation, Acc. Chem. Res., 2011, 44, 435–446 CrossRef CAS PubMed.
- E. Negishi, S. J. Holmes, J. M. Tour, J. A. Miller, F. E. Cederbaum, D. R. Swanson and T. Takahashi, J. Am. Chem. Soc., 1989, 111, 3336–3346 CrossRef CAS.
- E. Negishi, Dalton Trans., 2005, 827–848 RSC.
- E. Negishi, F. E. Cederbaum and T. Takahashi, Tetrahedron Lett., 1986, 27, 2829–2832 CrossRef CAS.
- S. Urrego-Riveros, I. Ramirez y Medina, D. Duvinage, E. Lork, F. D. Sönnichsen and A. Staubitz, Chem.–Eur. J., 2019, 25, 13318–13328 CrossRef CAS PubMed.
- J. Linshoeft, Synlett, 2014, 25, 2671–2672 CrossRef CAS.
- U. Rosenthal and V. V. Burlakov, Synthesis, 2002, 355–389 CAS.
- H. Sinn and G. Oppermann, Angew. Chem., Int. Ed. Engl., 1966, 5, 962–963 CrossRef CAS.
- H. Sinn and E. Kolk, J. Organomet. Chem., 1966, 6, 373–382 CrossRef CAS.
- W. Kaminsky and H. Sinn, Justus Liebigs Ann. Chem., 1975, 1975, 424–437 CrossRef.
- W. Kaminskly and H.-J. Vollmer, Justus Liebigs Ann. Chem., 1975, 1975, 438–448 CrossRef.
- W. Kaminsky, J. Kopf, H. Sinn and H.-J. Vollmer, Angew. Chem., Int. Ed. Engl., 1976, 15, 629–630 CrossRef.
- E. Negishi, D. Y. Kondakov, D. Choueiry, K. Kasai and T. Takahashi, J. Am. Chem. Soc., 1996, 118, 9577–9588 CrossRef CAS.
- I. R. Ramazanov and R. N. Kadikova, Kinet. Catal., 2020, 61, 106–111 CrossRef CAS.
- I. R. Ramazanov, R. N. Kadikova, Z. R. Saitova and U. M. Dzhemilev, Asian J. Org. Chem., 2015, 4, 1301–1307 CrossRef CAS.
- T. V. Tyumkina, D. N. Islamov, L. V. Parfenova, S. G. Karchevsky, L. M. Khalilov and U. M. Dzhemilev, Organometallics, 2018, 37, 2406–2418 CrossRef CAS.
- L. Brandsma and H. D. Verkuijsse, Synthesis of acetylenes, allenes, cumulenes: a laboratory manual, Elsevier SPC, Amsterdam-Oxford-New York, 1981 Search PubMed.
- M. J. Frisch, et al., Gaussian 09, Revision A.1, Gaussian, Inc., Wallingford, CT, 2009 Search PubMed.
- U. M. Dzhemilev, A. G. Ibragimov, V. A. D’yakonov and R. A. Zinnurova, Russ. J. Org. Chem., 2007, 43, 176–180 CrossRef CAS.
- W. You, X. Yan, Q. Liao and C. Xi, Org. Lett., 2010, 12, 3930–3933 CrossRef CAS PubMed.
- Z. Xi, X. Liu, J. Lu, F. Bao, H. Fan, Z. Li and T. Takahashi, J. Org. Chem., 2004, 69, 8547–8549 CrossRef CAS PubMed.
- V. A. D'Yakonov, R. A. Tuktarova and U. M. Dzhemilev, Russ. Chem. Bull., 2011, 60, 1633–1639 CrossRef.
Footnote |
† Electronic supplementary information (ESI) available. See DOI: 10.1039/d1ra08268j |
|
This journal is © The Royal Society of Chemistry 2021 |