DOI:
10.1039/D1RA07761A
(Paper)
RSC Adv., 2021,
11, 37354-37365
Comparison of drug release behavior of bacterial cellulose loaded with ibuprofen and propranolol hydrochloride†
Received
20th October 2021
, Accepted 8th November 2021
First published on 19th November 2021
Abstract
The aim of this study was to investigate the drug release behavior from bacterial cellulose (BC). Ibuprofen and propranolol hydrochloride were used as model drugs to represent low and highly water soluble drugs. The drug was loaded into the BC by immersing the partially swollen BC in a solution of drug concentrations ranging from 0.05 to 0.5 mg mL−1 and then drying by two different methods: air-drying and freeze-drying. The results showed that the type of drug and the drying method influenced the drug loading efficiency and drug release behavior. For ibuprofen, high drug loading efficiency was found when loading the drug into BC at low concentration and vice versa for propranolol hydrochloride. The drug-loaded BC prepared by the freeze-drying method showed a sustained release regardless of drug type and drug-loaded amount. The sustained release followed the Higuchi and Korsmeyer–Peppas models. On the other hand, when using the air-drying method, BC loaded with ibuprofen showed immediate release at every drug-loaded amount. However, BC loaded with propranolol hydrochloride showed immediate release at the high drug-loaded amount but showed sustained release at the low drug-loaded amount. The release of drug from a drug-loaded BC prepared by air-drying method tended to follow first-order kinetics. In conclusion, the drug loading concentration and the drying method in the drug-loaded BC preparation influenced the drug release characteristics of the BC-based drug delivery system.
Introduction
Bacterial cellulose (BC) is bacteria-derived cellulose that can be produced from a variety of bacteria genera, such as Acetobacter, Komagataeibacter, Agrobacterium, Rhizobium, and Sarcina.1 Its chemical structure is a linear polysaccharide of glucose ((C6H12O6)n) linked by β-1,4-glycosidic bonds, similar to plant-derived cellulose.2 BC consists of a very fine network of cellulose fibers (3–8 nm), giving it a soft tissue-like appearance. Since BC is not contaminated with hemicellulose or lignin compared with plant cellulose, BC is of high purity. These positive properties are beneficial for use on human tissues.3–5 Studies have confirmed that BC has good skin tolerance and does not induce hypersensitivity reactions to various human body tissues.6,7 Therefore, BC can be considered biocompatible and suitable for medical applications.
The application of BC for drug delivery has been reported. Initially, efforts were made for topical and transdermal drug delivery. It was later expanded to cover oral and other drug delivery systems in various routes of administration. Because BC has a three-dimensional nanoporous structure, it can hold all kinds of pharmaceutical active ingredients including small molecules, large molecules, and nanoparticles.8 The chemical structure of BC contains a large number of OH groups, giving it relatively hydrophilic properties.9 Therefore, the water solubility of the drugs is an important factor in the design of BC-based drug delivery systems. Generally, to use BC for drug delivery, it has been mixed with other excipients such as gelatin, pectin, carrageenan, and chitosan to increase its ability for drug-controlled release.10–13 The controlled releases obtained were then not from pure BC. Since each material has its properties, combinations of different materials possibly promote or interfere with each other in controlling drug release.14–16 In addition, the properties of the drug itself can affect the release controlling ability of the prepared drug delivery system.17 The controlled release performance of pure BC for each drug type should be understood before combining with other materials so that the most effective controlled-release behavior for the intended drug use can be achieved.
Several studies have examined BC as a single excipient for drug delivery. The drug-loaded BC is usually prepared by immersing the BC membrane, either swollen or dried membranes in a drug solution, and dried by air drying or freeze-drying methods. Trovatti et al. (2012) investigated the controlled released behavior of BC loaded with two types of drugs; lipophilic (ibuprofen) and hydrophilic (lidocaine hydrochloride). The air-drying method was used in the preparation of drug-loaded BC. They reported that BC loaded with ibuprofen showed higher but BC loaded with lidocaine hydrochloride showed lower permeation rates than those obtained from the conventional formulation.18 Badshah et al. (2017) investigated the effect of drying methods (air drying and freeze-drying) in the preparation of drug-loaded BC on the controlled release behavior of famotidine (low soluble model drug) or tizanidine (highly soluble model drug) loaded BC. They found that the drying method did not affect the drug release properties of both types of drugs.19 However, the study of Adepu and Khandelwal (2020) showed a contrasting trend, showing that the drying method affected the release behavior of the hydrophilic drug, diclofenac sodium.20 It should be noted that the type of drug and concentration found in those studies are different, which might yield different results. To better understand the controlled release behavior of drugs loaded BC, the effect of drug type and concentration, as well as the drying method, should be investigated in a single work.
This work aimed to provide a comprehensive study on the controlled released behavior of low soluble or highly soluble drugs loaded with pure BC. Ibuprofen and propranolol hydrochloride were used as model drugs, representing low and highly soluble drugs, respectively. Effects of drug loading concentration and drying methods (air drying and freeze-drying) in the preparation of drug-loaded BC on the controlled release behavior were investigated. The release kinetics of the BC membrane loaded with the drug was then explored.
Materials and methods
Materials
A fully swollen BC sheet with a thickness of about 1 cm was purchased from a local nata de coco manufacturer in Thasala district, Nakhon Si Thammarat, Thailand. It was produced by growing bacteria in coconut juice fortified with 4% (w/v) sucrose and left statically to culture for 7 days. The cellulose structure was in the form of extra-cellular pellicle corresponding to cellulose produced by Acetobacter xylinum, generally used for the production of nata de coco.21,22 Using that culture medium provided BC with pore size and pore distribution similar to using standard Schramm & Hestrin's medium.23 From microscopic structure, chemical constituents, and crystallinity pattern of the obtained BC could indicate its conformity with BC produced with Acetobacter xylinum.24,25 BC used in this study was obtained from the same batch, thus, the material characteristics were supposed to be the same. A fully swollen BC sheet was cut into size 6 cm × 6 cm × 1 cm (width × length × thickness, respectively), then rinsed thoroughly with distilled water until the pH of the rinsing water was close to 7. The fully swollen BC sheet was then placed in distilled water and stored in the refrigerator (4 °C) until use. Ibuprofen (≥98%) and propranolol hydrochloride (≥99%) were purchased from Sigma-Aldrich (St. Louis, MO, USA). All other chemicals and reagents used in this study were of analytical grade or HPLC grade.
Preparation of BC loaded with drug
Bacterial cellulose (BC) loaded with the drug was prepared by immersion and solvent evaporation technique. The fully swollen BC sheet (6 cm × 6 cm × 1 cm) was placed on an aluminum tray and gently pressed with a spatula to drain the water to obtain the remaining weight of the swollen BC sheet about 50% of the initial weight. The obtained BC was called partially swollen BC. Ibuprofen solution and propranolol hydrochloride solution at three concentrations of 0.05, 0.1, and 0.5 mg mL−1 were prepared using a similar preparation procedure as follows: the stock solution (10 mg mL−1) of each drug was prepared by dissolving the drug in ethanol, then, the stock solution was diluted with 20% ethanol to obtain the desired concentrations.
A partially swollen BC sheet was immersed in 40 mL of drug solution to load the drug into BC. It was performed in duplicate for each drug concentration for being used in two different drying methods. The drug solution was stirred at 50 rpm at room temperature (25 ± 1 °C) for 24 h to ensure that equilibrium was achieved. The swollen BC sheets were then taken from the immersing solution and the excess solution on the surface was removed by blotting with tissue paper before drying.
To dry the drug-loaded BC sheet, two different methods, air drying, and freeze-drying were used in the comparison. For the air-drying method, the swollen drug-loaded BC sheet was put on an aluminum tray and dried in a hot air oven (UFE600; Memmert GmbH Co. KG, Buchenbach, Germany) at 45 °C for 12 h. For the freeze-drying method, the swollen drug-loaded BC sheet was pre-frozen at −80 °C for 12 h before put in the freeze dryer (Gamma 2-16 LSC; Martin Christ Gefriertrocknungsanlagen GmbH, Osterode am Harz, Germany) until dried (about 24 h). The blank BC including air-dried BC (BC-AD) and freeze-dried BC (BC-FD) to be used as the control were also prepared using the same procedure as the preparation of drug-loaded BC but without the drug loading. The given name of all prepared BC loaded with drugs and their controls is shown in Table 1.
Table 1 Name, composition, and drying method of each prepared BC loaded with the drug and the control BC
Name |
Type of drug loading |
Concentration of drug solution (mg mL−1) |
Drying method |
BC-AD |
— |
— |
Air-drying |
BC-FD |
— |
— |
Freeze-drying |
BC-I1-AD |
Ibuprofen |
0.05 |
Air-drying |
BC-I2-AD |
Ibuprofen |
0.1 |
Air-drying |
BC-I3-AD |
Ibuprofen |
0.5 |
Air-drying |
BC-I1-FD |
Ibuprofen |
0.05 |
Freeze-drying |
BC-I2-FD |
Ibuprofen |
0.1 |
Freeze-drying |
BC-I3-FD |
Ibuprofen |
0.5 |
Freeze-drying |
BC-P1-AD |
Propranolol HCl |
0.05 |
Air-drying |
BC-P2-AD |
Propranolol HCl |
0.1 |
Air-drying |
BC-P3-AD |
Propranolol HCl |
0.5 |
Air-drying |
BC-P1-FD |
Propranolol HCl |
0.05 |
Freeze-drying |
BC-P2-FD |
Propranolol HCl |
0.1 |
Freeze-drying |
BC-P3-FD |
Propranolol HCl |
0.5 |
Freeze-drying |
Swelling study
The swelling ability of dried BC prepared by air-drying and freeze-drying methods (BC-AD and BC-FD) were evaluated in phosphate buffer saline (PBS) pH 7.4 and citrate–phosphate buffer pH 5.5 which were used as the medium in the drug release study in order to evaluate the correlation between BC swelling and drug release behavior. The hydrophilic/hydrophobic character of drug loading into BC might affect the swelling ability of BC,26 the drug-loaded BC were then also evaluated in comparison with the blank BC. The highest amount of drug-loaded BC i.e., BC-I3-AD, BC-I3-FD, BC-P3-AD, and BC-P3-FD were used for this purpose. The membrane sample at a surface size of 2 cm × 2 cm was cut from the original dried membrane, weighed, and placed in 100 mL buffer which was stirred at 50 rpm and set the temperature at 37 °C. The membrane was taken from the medium to measure the weight at specified times during 5 min to 6 h and at 24 h, which is the saturation point. The wet membranes were gently blotted with a tissue paper to remove the excess medium on the surface before weighing. The experiment was performed in triplicate for each type of membranes. The swelling of BC and drug-loaded BC was calculated as the swelling ratio using the following equation: |
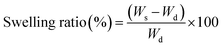 | (1) |
where, Ws is the wet weight of the swollen membrane (g), and Wd is the weight of dried membrane before being placed in the medium (g).
Drug loading evaluation
The amount of drug-loaded in the BC membrane was evaluated by the direct extraction method. The drug-loaded membrane was cut from the original dried membrane with a cutter to a surface size of 2 cm × 2 cm, then immersed in 4 mL of ethanol and extracted the loaded drug using an ultrasonic sonicator (E300H Elmasonic, USA) at room temperature (25 ± 1 °C) for 20 min. The membrane was lifted from the solution and rinsed with 1 mL of ethanol before discarding. The experiment was performed in triplicate for each type of membranes. The obtained solution was adjusted to 5 mL with ethanol in a volumetric flask. The solution was filtered through a 0.45 μm filter paper and diluted with ethanol to the appropriate concentration for analysis. The drugs were analyzed by a UV-vis spectrophotometer (V-630; JASCO Deutschland GmbH, Pfungstadt, Germany) at 265 nm for ibuprofen27 and 290 nm for propranolol hydrochloride.28 The analytical methods had been optimized and validated in specificity, linearity and range, accuracy, and precision according to ICH guidelines29 prior to use. Ethanol (solvent), standard drug solution in ethanol, and ethanol after blank BC extraction were scanned in the range of 200–400 nm. It was found that the λmax for ibuprofen and propranolol hydrochloride were 265 nm and 290 nm, respectively, and were not disturbed by solvent or any traces from the BC. Calibration standards were obtained by dissolving the standard drug in ethanol and found linearity in the application range of 20–400 μg mL−1 and 5–40 μg mL−1 for ibuprofen and propranolol hydrochloride, respectively, where R2 > 0.999. For evaluating accuracy and precision, standard drug solution was spiked in ethanol in 3 concentrations and 3 replicates (9 determinations). The methods were found to be accurate and precise, where recovery in the range of 98–103%, and RSD within ±2% for both drugs analysis. Details of the analytical method validation results are shown in the ESI (S1 and S2).† The amount of drug-loaded in the BC membrane at the original size (surface size of 6 cm × 6 cm) was calculated using the rule of three in arithmetic. The drug loading was calculated as the percentage of entrapment efficiency (EE) using the following equation: |
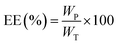 | (2) |
where WP is the practical drug content in the BC membrane size 6 cm × 6 cm (mg), and WT is the theoretical drug content in the immersing solution (mg) initially.
Characterization of BC loaded with drug
Scanning electron microscopy (SEM) study. The surface and morphology of BC loaded with the drug were observed using a scanning electron microscope (Zeiss Merlin FEG-SEM; Carl Zeiss, Oberkochen, Germany). A small piece of the drug-loaded BC membrane was sputter-coated with a thin layer of gold with a sputter coater (108 Auto; Cressington, Watford, UK) before the examination. All images were taken at an accelerating voltage of 5 kV.
Fourier transform infrared (FTIR) spectroscopy study. FTIR was used to evaluate whether drugs loaded in BC, ibuprofen, or propranolol hydrochloride, have a chemical bond with BC. The KBr technique was adopted for this study. The drug-loaded BC membrane was cut into small pieces and mixed with KBr about 1
:
50 sample to KBr ratio (by weight), then pressed to form a disk using 10 tons pressing load. Samples were examined using the FTIR spectrophotometer (Tensor 27; Bruker, Ettlingen, Germany) in the wavenumber region of 4000–400 cm−1 at a resolution of 4 cm−1.
Differential scanning calorimetry (DSC) study. The DSC thermograms of BC loaded with the drug were determined using a differential scanning calorimeter (DSC 6000; PerkinElmer, Waltham, MA, USA). A drug-loaded BC membrane of about 3–5 mg was sealed in an aluminum pan and scanned at a temperature range of 50–300 °C with a heating rate of 5 °C min−1 under nitrogen flow of 20 mL min−1.
X-ray diffractometry (XRD) study. XRD was used to evaluate how crystallinity changes of the drug and BC after the drug-loaded BC preparation. The XRD patterns of BC loaded with the drug were measured using an X-ray diffractometer (Empyrean; PANalyticical, Netherlands) at the Cu Kα radiation wavelength of 0.154 nm, generated voltage of 40 kV and filament emission of 30 mA. Samples were scanned in the 2θ range of 5–90° with a step size of 0.026°.
Drug release study
The release behavior of BC loaded with the drug was studied in phosphate buffer saline (PBS) pH 7.4 using in vitro dissolution method. The membrane was cut with a cutter to a surface size of 2 cm × 2 cm and placed in a vessel of dissolution apparatus 2 (708-DS; Agilent Technologies, Santa Clara, CA, USA) containing 500 mL PBS pH 7.4. The medium was set at 37 °C and stirred at 50 rpm throughout the study. The two milliliter medium was withdrawn with the same volume of fresh medium replaced over 5 min to 6 h. The effect of pH and ionic strength of the dissolution medium were also evaluated. Citrate–phosphate buffer pH 5.5 was used in comparison with PBS pH 7.4, and PBS pH 7.4 at the ionic strength (μ) of 0.19 and 0.33 M adjusted with NaCl were used for evaluation of ionic strength effect. The experiment was performed in triplicate for each type of membranes in each condition. The drug content was analyzed using high-performance liquid chromatography (HPLC) connected with a diode-array detector (1290 Infinity LC System; Agilent Technologies, Santa Clara, CA, USA) and a C18 analytical column (4.6 × 100 mm, 3.5 μm, Zorbax Eclipse Plus; Agilent Technologies, Santa Clara, CA, USA) was used. The mobile phase and detection wavelength for ibuprofen analysis were phosphate buffer pH 6.8/acetonitrile (65
:
35) and 220 nm, respectively, and those for propranolol hydrochloride analysis were phosphate buffer pH 3.5/acetonitrile (66
:
34) and 290 nm, respectively. The analytical methods had been optimized and validated in specificity, linearity and range, accuracy, precision, limit of detection (LOD), and limit of quantitation (LOQ) according to ICH guidelines29 prior to use. From the HPLC chromatogram of the sample, which corresponded to the standard solution chromatogram and did not interfere with other peaks, indicated the specificity of the analytical method. The linearity was achieved in the application calibration curves ranging from 0.05 to 10 μg mL−1 for ibuprofen and from 0.1 to 10 μg mL−1 for propranolol hydrochloride, where R2 > 0.999. The accuracy and precision were determined by spiking standard solution in dissolution medium in 3 concentrations and 3 replicates (9 determinations), and found that the analytical methods were accurate and precise, where the recovery between 97 and 103% and RSD within ±2% for both drugs analysis. The LOD and LOQ were calculated according to the equation LOD = 3.3 × σ/S and LOQ = 10 × σ/S, where σ is the standard deviation of the response and S is the slope of the calibration curve. The LOD and LOQ for ibuprofen analysis were 0.014 μg mL−1 and 0.045 μg mL−1, respectively, and for propranolol hydrochloride were 0.030 μg mL−1 and 0.091 μg mL−1, respectively. Details of the analytical method validation results are shown in the ESI (S3 and S4).†
The release kinetics were analyzed using four release kinetic models including zero-order, first-order, Higuchi, and Korsmeyer–Peppas models. The equations of these models are as follows:30
|
Zero-order kinetics: Ft = K0t
| (3) |
where
Ft is the fraction of drug released in time
t and
K0 is the zero-order release constant.
|
First-order kinetics: ln(1 − F) = −K1t
| (4) |
where
F is the fraction of drug released in time
t and
K1 is the first-order release constant.
|
Higuchi model: F = KHt1/2
| (5) |
where
F is the fraction of drug released in time
t and
KH is the Higuchi release constant.
|
Korsmeyer–Peppas model: F = Ktn
| (6) |
where
F is the fraction of drug dissolved in time
t,
K is the release rate constant and
n is the release exponent, indicating the drug release mechanism. The release data at <60% were used for this model.
The release data were plotted according to each release kinetic equation and the release kinetics correlation coefficient (R2), release rate constant (K0, K1, and KH) and n were reported.
Statistical analysis
Data are presented as mean ± standard deviation (SD). One-way ANOVA was used to assess differences. A p-value of less than 0.05 was considered statistically significant. Linear regression was used to assess the correlation between swelling and drug release, as well as to study the release kinetics.
Results and discussion
Preparation of BC loaded with drug
Both ibuprofen and propranolol hydrochloride, which are representative of low and highly soluble drugs, respectively, could be successfully loaded in the BC by immersion and solvent evaporation technique. BC exhibits amphiphilic properties that have both hydrophilic and hydrophobic properties,31 resulting in binding to both low and highly soluble drugs. The different drying methods used in this study – air drying and freeze-drying – resulted in membranes with different physical characteristics. Fig. 1 shows a representation of the physical appearances of BC loaded with the drug and dried by air drying compared with freeze-drying methods. By using an air-drying method, the obtained membrane was relatively thin, paper-like (Fig. 1A) with a thickness of about 0.3 mm measured by Vernier caliper. While using a freeze-drying method, the obtained membrane was flossy, resembling a foam pad (Fig. 1B) with the thickness was about 0.5 cm and could be collapsed with finger force. The weight of the dried membranes prepared by air-drying and freeze-drying methods were not different. It was about 0.3 g for the original dried membrane with a surface size of 6 cm × 6 cm and when cut into a surface size of 2 cm × 2 cm, the weight of each piece was about 0.03 g. The thickness and weight of individual type of membranes are shown in Table 2. The thickness and weight were not different between un-loaded and drug-loaded membranes. This would be due to very small amount of drug was loaded into membrane compared to the mass of cellulose, and would be below the sensitivity of the instruments to detect. From the same weight but different thickness of air-dried and freeze-dried membranes, it was implied that the density of these two type of membranes was different. The calculated densities of the air-dried and freeze-dried membranes were approximately 0.2 g mL−1 and 0.016 g mL−1, respectively, which differed approximately 13 times. The freeze-drying membrane had lower density because it had highly porous structure. Solvent evaporation during air drying induced capillary pressure of the solvent meniscus, exerted a compression force in the pores of the membrane, resulting in modifications to the structure, density, and porosity of the BC membrane.32 In a freeze-drying process, free water was frozen into ice crystals which were sublimated and eventually represented pores in the BC membrane. The freeze-drying method made the fibrils of BC more resistant to compactness during drying and finally obtaining a more spongy structure.33,34 Loading of different drugs (ibuprofen or propranolol hydrochloride) when using the same drying method resulted in no difference in physical appearance. Using different drug concentrations also did not affect physical appearance.
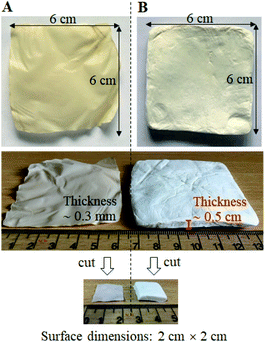 |
| Fig. 1 Representative of the physical appearances of BC loaded with a drug which dried by (A) air drying and (B) freeze-drying methods. | |
Table 2 Thickness, weight and swelling ratio at 6 h and 24 h of different membranes with a surface size of 2 cm × 2 cm. Results shown are mean and SD are in the bracket, n = 3
Type of membrane |
Thickness (mm) |
Weight (g) |
Swelling ratio at 6 h (%) |
Swelling ratio at 24 h (%) |
pH 7.4 |
pH 5.5 |
pH 7.4 |
pH 5.5 |
BC-AD |
0.34 (0.04) |
0.028 (0.002) |
118.74 (4.08) |
109.87 (7.79) |
122.76 (3.82) |
110.53 (5.87) |
BC-FD |
4.97 (0.18) |
0.028 (0.002) |
3579.63 (456.00) |
3727.19 (400.01) |
4280.53 (408.30) |
4028.18 (317.85) |
BC-I3-AD |
0.35 (0.02) |
0.028 (0.002) |
120.37 (2.64) |
111.49 (4.77) |
121.46 (2.45) |
112.99 (3.25) |
BC-I3-FD |
5.09 (0.12) |
0.029 (0.001) |
3426.59 (126.47) |
3544.48 (250.93) |
4117.79 (100.63) |
3887.27 (154.24) |
BC-P3-AD |
0.34 (0.01) |
0.029 (0.002) |
117.89 (6.05) |
109.60 (5.85) |
118.98 (6.59) |
111.09 (4.76) |
BC-P3-FD |
4.99 (0.03) |
0.029 (0.002) |
3414.56 (371.29) |
3536.32 (125.83) |
4100.10 (383.26) |
3885.84 (141.15) |
Swelling
From the swelling study, the results are shown in Table 2 and Fig. 2. It was found that the swelling ability between air-dried and freeze-dried membranes was enormously different. For the air-dried BC membrane after being immersed in the aqueous medium, the swelling ratio changed from 0 to about 100% within 15 min and saturation point was reached after 30 min and 2 h in the buffer pH 5.5 and pH 7.4, respectively. The swelling ratio at the saturation point was about 110% and 120% in buffer pH 5.5 and pH 7.4, respectively (Fig. 2A). The swelling ratio of air-dried BC membrane was at the same level as the report of Adepu and Khandelwal.20 The swelling of air-dried BC membrane after saturation point was found to be pH-dependent. In buffer pH 5.5 the swelling of BC after saturation was significantly lower (p < 0.05) than in buffer pH 7.4. This was due to the characteristics of cellulose causing swelling in an acidic medium (pH < 5) lower than in alkali medium (pH > 7).26 For freeze-drying BC membrane, its swelling ratio was much higher than that of air-dried BC membrane; it was more than 1000%. The swelling ratio increased rapidly during the first 15 min and gradually increased thereafter throughout the 6 h. There was no difference in swelling ratio in pH 7.4 and pH 5.5 buffers during the 6 h study period. At 24 h, swelling ratio in pH 7.4 buffer seemed to be higher than in pH 5.5 buffer (Table 2), however, they were not statistically significantly different (p > 0.05). The difference in swelling at pH 5.5 and 7.4 of the freeze-dried BC membrane might not be apparent because the swelling during the study time did not reach the saturation point. The effect of drug loading on BC swelling was also evaluated and results are shown in Fig. 2B and C. The maximum drug loading used in this study had no effect on BC swelling being dried in different methods or in different pH media. BC swelling behavior may influence drug release behavior.20 The relationship between BC swelling and drug release will be discussed in the drug release section.
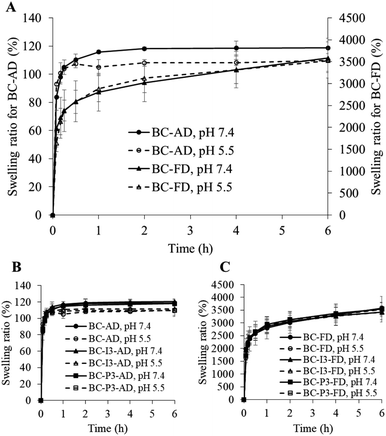 |
| Fig. 2 Swelling ratio of (A) blank BC dried by air-drying and freeze-drying methods in pH 7.4 and pH 5.5 buffers, and ibuprofen-loaded BC at the highest drug loading which dried by (B) air-drying method and (C) freeze-drying method in pH 7.4 and pH 5.5 buffers. Results shown are mean ± SD, n = 3. | |
Drug loading
From the drug loading study, the results are shown in Fig. 3. The amount of drug-loaded in the BC increased when the drug loading concentration increased for both two types of drugs. However, at the same concentration, the amount of drug-loaded was different between these two drugs. At low concentration, the amount of ibuprofen loaded was higher than that of propranolol hydrochloride (p < 0.05). It could be observed clearly at the lowest concentration, 0.05 mg mL−1, the amount of ibuprofen loaded was about 2 times higher than that of propranolol hydrochloride. This could be explained by the ‘like dissolves like rule’35 as that propranolol hydrochloride, a highly soluble drug, is more likely to dissolve in a drug solution, which is an aqueous solution, than to diffuse into BC which is naturally amphiphilic.31
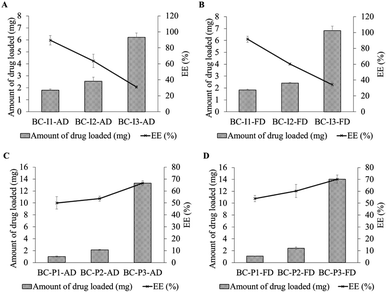 |
| Fig. 3 Amount of drug-loaded in BC size 6 cm × 6 cm (mg) and EE (%) using different drug loading concentrations and drying methods; (A) and (B) are for ibuprofen and (C) and (D) are for propranolol hydrochloride using air-drying and freeze-drying methods, respectively. Results shown are mean ± SD, n = 3. | |
When the drug concentration increased, the amount of drug-loaded for both two drugs increased. This positive correlation between drug loading concentration and the drug-loaded amount was commonly observed in drug-loaded BC preparations.36–38 At higher concentrations, the drug molecules could be more driven from drug solution to BC compared with at lower concentrations. However, when drug concentration increased, the amount of ibuprofen loaded was increased less than that of propranolol hydrochloride. It was found that at the middle studied concentration, 0.1 mg mL−1, the amount of drug-loaded for ibuprofen and propranolol hydrochloride was at a relatively similar level, but at the highest concentration, 0.5 mg mL−1, the amount of propranolol hydrochloride loaded was about 2 times higher than that of ibuprofen (p < 0.05). This might be explained by the binding capacity of BC. The binding capacity of BC would be related to the physicochemical properties of BC and drug molecules. Since BC is composed of glucose units, therefore, it is relatively polar and could be well absorbed for polar drug molecules.39 Since propranolol hydrochloride molecule has a higher polar surface area (41.5 Å2) than ibuprofen (37.3 Å2), therefore propranolol hydrochloride molecules could be more entrapped in the BC compared with ibuprofen molecules.
For entrapment efficiency (EE), the trend of EE from low to high drug loading concentration compared between ibuprofen and propranolol hydrochloride was different; the trend for ibuprofen decreased, while for propranolol hydrochloride increased. The result implied that the amount of drug loading tended to be more dependent on the drug-BC like than to the drug-medium like when high drug loading is required. These results were consistent with the results of Badshah et al., 2017. It was seen that the percentage drug binding of highly soluble drug with BC increased with increasing immersion time while low soluble drug showed the opposite direction.19 Furthermore, the results showed that the amount of drug loading and EE trends of each drug were similar from different drying methods. It could be indicated that the drying method did not affect the amount of drug loading and EE. Although the density and fiber entanglement of the BC membrane play an important role in the drug holding capacity,40 this was not observed in this study as the drug was loaded in the membrane prior to drying. Different morphological features appear after the drug loading process, which was not related to drug loading capacity.
Characterization of BC loaded with drug
SEM. From SEM images (Fig. 4), it had been revealed that BC consisted of networked nanofibrils. There were no additional particles on the drug-loaded BC membrane, indicating that the loading of drug in the BC by immersion and solvent evaporation technique allowed the drug to simultaneously distribute into the fibers. The morphology of pure BC dried by air-drying and freeze-drying methods was different (Fig. 4A and B). Their morphology corresponded to their physical appearance. The air-drying method caused the solvent to evaporate rapidly, resulting in a greatly reduced membrane thickness, resulting in a noticeably greater density of fiber entanglement. On the other hand, freeze-drying allowed the original structure and characteristics of the BC to be preserved, resulting in the appearance of the nanofibrils as a three-dimensional network structure. The freeze-dried BC membrane was therefore highly porous with slightly thinner fibers than the air-dried BC membrane. The morphology of BC is an important factor affecting drug release behavior.3,41
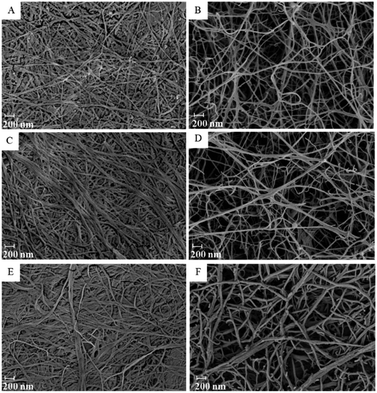 |
| Fig. 4 SEM images of blank BC dried by (A) air drying and (B) freeze-drying methods and BC loaded with ibuprofen and propranolol hydrochloride and dried by air-drying and freeze-drying methods: (C, D, E and F) are BC-I3-AD, BC-I3-FD, BC-P3-AD, and BC-P3-FD, respectively. | |
When the BC was loaded with the drug, it could be observed that the fibers were slightly thicker compared to the unloaded (blank) membrane especially in the air-dried membrane (Fig. 4C and E). According to the capillary pressure of the solvent meniscus and compression force in the pore of the membrane from the air-drying process, the fibers of the membrane were collapsed to pack as a thin film.32 The loaded drug would be compressed and rearranged the position during the drying process. Due to the confined space of the thin film, the loaded drug would be extruded and distributed along the compressed fibers, allowing thicker fibers to be observed. For freeze-dried membrane, the drug would be distributed into the whole three dimensions of the membrane, the thickness of the fibers was, therefore, smaller (Fig. 4D and F).
FTIR analysis. According to the content of BC compared to that of the drug in the drug-loaded BC was very high, the highest amount of drug-loaded BC membrane (BC-I3- and BC-P3-AD and FD) was used in this study, otherwise, the signal of BC obscured all the signal of the drug. The FTIR spectra is shown in the ESI (S5).† The apparent FTIR peak of ibuprofen appeared at 1695 cm−1, corresponding to C
O stretching. This functional group could be able to form hydrogen bonds with the OH group of cellulose. If the occurrence of hydrogen bonds, a shift of C
O stretching frequency would be observed and a new peak corresponding to the hydrogen bonding according to the C
O group would also appear.42,43 In this study, however, no shift of C
O group stretching could be observed from the membrane loaded with ibuprofen from both air drying and freeze-drying methods.For BC loaded with propranolol hydrochloride, it was also observed in the same phenomena as found in BC loaded with ibuprofen. The major characteristic peaks of pure propranolol show at 3280 cm−1, which are attributed to the NH stretching of secondary amine; at 1579 cm−1, which are associated with aryl C
C stretching; around 1030–1240 cm−1, which are associated with the aryl O–CH2 stretching; and around 771–797 cm−1, which were assigned to alpha-substituted naphthalene.44 The shift in frequency of NH stretching would be important for determining hydrogen bond formation,45 however, the peak of NH group stretching of propranolol hydrochloride could not be observed in the drug-loaded BC membrane because it was obscured by the peak of the OH group of BC. Some other characteristic peaks and some major peaks in the fingerprint region of pure propranolol could be still recognized at the same wavenumber in the propranolol hydrochloride loading membrane.
Results obtained from the FTIR studies should indicate that no chemical interaction occurred between ibuprofen or propranolol hydrochloride and BC from drug-loaded BC preparation.
DSC analysis. DSC study provides thermal characteristics of samples. The obtained data can be used to support the FTIR result in the explanation of molecular interaction. From DSC chromatograms (Fig. 5), pure ibuprofen and propranolol hydrochloride showed a sharp endothermic peak at 76 and 160 °C correspondings to their melting point. Blank BC showed a broad endothermic peak around 80–90 °C according to dehydration.46 The freeze-dried BC might be more sensitive to moisture, making the dehydration peak more visible. Another endothermic peak of BC could be observed around 250–270 °C corresponding to the degradation point.46 The BC loaded with the drug showed an endothermic peak that correlated with the peak of the pure drug for both ibuprofen and propranolol hydrochloride loaded membrane but slightly shifted to a lower temperature. The shift of the DSC peak may indicate the presence of molecular interactions or may be due to the change in the crystalline form.47 Considered in conjunction with the results of FTIR, the shift of the drug peak when loaded in the membrane as that observed in DSC was likely due to the change in crystalline form. Since in the preparation of the drug-loaded membrane, the drug dissolved and dried up again after being loaded into the membrane, the crystallinity of the drug can be therefore changed.
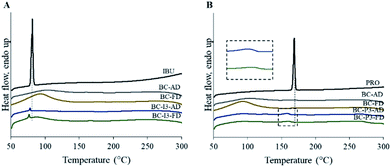 |
| Fig. 5 DSC patterns of the pure drug, blank BC (BC) using two different drying methods, and BC loaded with related drug using two different drying methods; (A) is for ibuprofen (IBU) and (B) is for propranolol hydrochloride (PRO). | |
XRD analysis. Fig. 6 shows XRD patterns of pure drugs (ibuprofen and propranolol hydrochloride), pure BC, and drug-loaded BC. It was found that both ibuprofen and propranolol hydrochloride showed numerous characteristic sharp and intense peaks, indicating that these two pure drugs were in crystalline form. BC showed three characteristic diffraction peaks at 14.5, 16.6, and 22.6°. These peaks could be attributed to the presence of both Iα and Iβ crystal cellulose.48 Since the intensity of the 14.5° peak is larger than that of the 16.6°, the crystal cellulose should contain mostly Iα.49 The XRD patterns of air-dried and freeze-dried BC were similar. This implied that the drying method did not affect the change of crystallinity of BC. When the drug either ibuprofen or propranolol hydrochloride was loaded into BC, some small diffraction peaks appeared in addition to the peak of BC. These peaks would correspond to the peaks of the drug, however, the position of the peaks was changed from the peaks of the pure drug. This might be due to the change of crystalline form of the drug according to dissolution that changes the solid-state form of drug to liquid and reform to a solid-state again within the membrane. This process could be able to change the crystalline form of the solid substance.50 In addition, as a result of FTIR and DSC indicating that there was no chemical interaction between drug and BC, it was likely another explanation that the change of crystalline form of the drug in the membrane was due to a drug-state transformation, not from a chemical interaction.
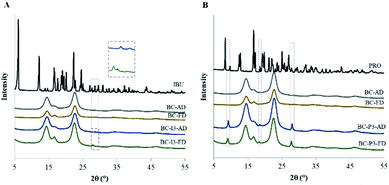 |
| Fig. 6 XRD patterns of the pure drug, blank BC (BC) using two different drying methods, and BC loaded with related drug using two different drying methods; (A) is for ibuprofen (IBU) and (B) is for propranolol hydrochloride (PRO). | |
Drug release
From the release study, it was found that the drug-loaded BC membranes prepared by the freeze-drying method, whether loaded with low or highly soluble drugs, ibuprofen or propranolol hydrochloride, released drugs slower than the membranes prepared by the air-drying method. As shown in Fig. 7A1 and A2, the air-dried BC membrane released the drug very fast from the beginning to reach the plateau within 0.5 h, while the freeze-dried BC membrane had a burst release at the initial stage (first 15 min) and later sustained-release until reached about 100% at the time about 4–6 h. It had been stated that the denser structure of the drug delivery system led to a slower and sustained release rate,10 however, the result from this study revealed that the looser structure freeze-dried membrane had released the drug slower than the denser structure air-dried membrane. This might be due to the rearrangement of drug molecules in the prepared membrane. By the air-drying method, the solvent gradually evaporated from the swollen membrane during the drying process, allowing the membrane to collapse as a flat sheet. The drug molecules inside would be therefore driven closely to each other and finally composed as a dried state in the flat membrane especially at the edge of the membrane surface as observed from the SEM. The drug molecule would be therefore ready to contact the dissolution medium, leading to obtaining a very fast release. For freeze-dried membranes, the three dimensions of the membrane did not change after dried, resulting in a large surface area.51 This might make the drug molecules bound with BC stronger, therefore, sustain the drug release. The initial burst release observed from the freeze-dried membrane might be from the drug adhered to the fibrous outer surface of the membrane, readily released upon contact with the dissolution medium.
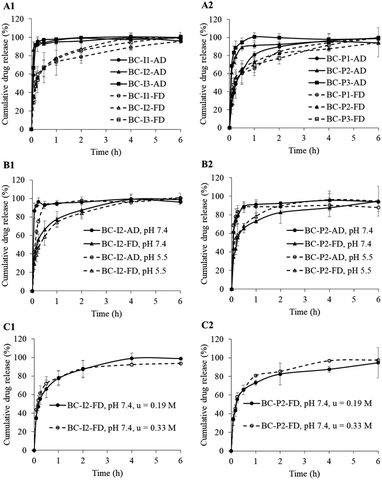 |
| Fig. 7 The drug-release profiles of BC loaded with ibuprofen and propranolol hydrochloride dried by air-drying and freeze-drying methods when the drug loading dose was different ((A1) for ibuprofen; (A2) for propranolol hydrochloride) in a medium with different pH ((B1) for ibuprofen; (B2) for propranolol hydrochloride) and a medium with different ionic strength ((C1) for ibuprofen; (C2) for propranolol hydrochloride). Results shown are mean ± SD, n = 3. | |
The results observed in this study supported the statement that BC morphology influenced drug release behavior. The use of different bacterial strains or culture media can lead to different BC morphology, resulting in different physicochemical properties.40,41 The presence of BC in the form of sheets in which the fibers are in a web-like structure has an advantage over plant-derived cellulose in that it has a high water-holding capacity.3 The use of culture media leading to the production of fibers with low thickness but high fiber density resulted in high drug retention properties and sustained drug release capability.40 The morphology of BC could be controlled by preparation such as using different drying methods like those used in this study. Since the original swollen BC used in this study had high thickness (about 1 cm), the result air-dried and freeze-dried membranes had a large difference in thickness. It meant that the porosity, density and three-dimensional structure within the membrane differ greatly. This would be the reason to obtain sustained drug release from freeze-dried membrane instead of the air-dried membrane.
The sustained release was also observed from the air-dried membrane loaded with propranolol hydrochloride at the lowest drug loading concentration. The sustained-release behavior might be due to the less amount of drug-loaded, leading to being able to control the rate of drug release.52 However, could not be observed the sustained release from the air-dried membrane loaded with ibuprofen even at the lowest drug loading level. This might be because the amount of ibuprofen loaded in the membrane was about twice higher than propranolol hydrochloride at the same low drug loading concentrations, causing ibuprofen to be released more easily. It might also be that the lipophilic property of ibuprofen and the hydrophilic environment of BC led to weaker interaction between ibuprofen and BC, resulting in fast drug release.18 In addition, ibuprofen is a weak acid with the pKa is about 4.4, it almost fully ionized in the dissolution medium, PBS pH 7.4, therefore, ionized ibuprofen could be released from the BC to the dissolution medium very fast.53
The sustained release of ibuprofen from the membrane prepared by air-drying method could be observed only when the drug release was performed in the buffer pH 5.5 (Fig. 7B1). This might be due to the ionization of the weak acid ibuprofen being reduced when in the acidic dissolution medium compared to in the alkaline medium. The release rate of ibuprofen in an acidic medium was then slower than in an alkaline medium.
Reports were stating that the ionic strength could affect the BC swelling by decreasing the osmotic pressure of the medium, resulting in decreased swelling, then would affect the release behavior of drug from BC.54,55 In this study, the effect of ionic strength was tested only with a freeze-dried membrane loaded with a drug. It was observed that no change in drug release when the ionic strength of the dissolution medium was changed between 0.19 M and 0.33 M (Fig. 7C). This might be explained that the freeze-dried membranes were already fully swelled according to their preparation process, the swell would not be therefore affected by the ionic strength.
In addition, correlation between swelling and drug release was investigated using a linear regression method. Fig. 8 shows linear fitting between those two parameters of BC membranes loaded with the same drug in the same amount but different in drying method (BC-I3-AD and BC-I3-FD). It was found that the correlation coefficient of freeze-dried membrane (R2 = 0.9968) was higher than that of air-dried membrane (R2 = 0.8392), indicating that swelling was associated with drug release of the freeze-dried membrane than with the air-dried membrane. This result was consistent with the study by Adepu and Khandelwal.20 The presence of a wide swelling range of the freeze-dried membrane could influence in a high correlation between swelling and drug release. As could be observed in the Fig. 8B, the release of the drug is gradually increasing, accompanied by swelling of the membrane. On the other hand, because the air-dried membrane had a narrow range of swelling, occurred rapidly and was almost complete in a short time, swelling was less effective at controlling drug release.
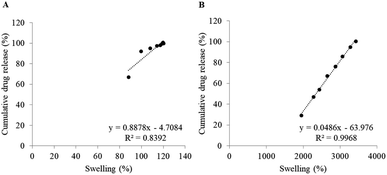 |
| Fig. 8 Linear fitting between swelling and cumulative drug release of (A) BC-I3-AD and (B) BC-I3-FD in PBS pH 7.4. | |
From the release kinetics analysis (Table 3), it was found that the air-dried BC membrane loaded with ibuprofen tended to release the drug following first-order kinetics rather than zero-order kinetics. This means that the rate of drug release is dependent on the amount of drug-loaded within the membrane.20
Table 3 Release kinetics correlation coefficient (R2) and release rate constant from the use of zero-order, first-order, Higuchi, and Korsmeyer–Peppas models of BC loaded with ibuprofen and propranolol hydrochloride dried by different drying methods. Results shown are mean and SD are in the bracket, n = 3
Type of membrane |
Zero-order |
First-order |
Higuchi |
Korsmeyer–Peppas |
R2 |
K0 (μg h−1) |
R2 |
K1 (h−1) |
R2 |
KH (h−1/2) |
R2 |
n |
Is for the first 15 min. Is for 30 min to 6 h. |
BC-I1-AD |
0.896 (0.010) |
199.240 (12.420) |
0.998 (0.014) |
6.515 (0.382) |
0.937 (0.020) |
161.950 (2.984) |
0.957 (0.028) |
0.391 (0.022) |
BC-I2-AD |
0.870 (0.015) |
175.027 (14.362) |
0.918 (0.022) |
5.662 (0.244) |
0.916 (0.013) |
146.261 (19.980) |
0.948 (0.026) |
0.336 (0.053) |
BC-I3-AD |
0.845 (0.002) |
170.675 (17.890) |
0.931 (0.023) |
4.913 (0.101) |
0.895 (0.001) |
137.810 (16.712) |
0.928 (0.001) |
0.340 (0.063) |
BC-I1-FDa |
0.969 (0.010) |
152.570 (14.366) |
0.986 (0.010) |
1.278 (0.054) |
0.989 (0.018) |
120.930 (19.049) |
0.992 (0.003) |
0.509 (0.064) |
BC-I1-FDb |
0.977 (0.027) |
4.506 (0.933) |
0.977 (0.031) |
0.158 (0.033) |
0.990 (0.005) |
15.734 (1.040) |
— |
— |
BC-I2-FDa |
0.965 (0.001) |
118.923 (17.228) |
0.985 (0.004) |
0.971 (0.290) |
0.979 (0.001) |
92.529 (11.152) |
0.976 (0.001) |
0.489 (0.060) |
BC-I2-FDb |
0.922 (0.054) |
5.096 (0.614) |
0.965 (0.022) |
0.353 (0.020) |
0.964 (0.002) |
18.135 (0.488) |
— |
— |
BC-I3-FDa |
0.949 (0.034) |
149.850 (14.351) |
0.968 (0.039) |
1.182 (0.052) |
0.974 (0.053) |
119.230 (18.567) |
0.979 (0.026) |
0.578 (0.019) |
BC-I3-FDb |
0.932 (0.023) |
4.644 (0.511) |
0.986 (0.013) |
0.332 (0.016) |
0.976 (0.025) |
16.463 (0.753) |
— |
— |
BC-P1-AD |
0.944 (0.034) |
140.800 (9.261) |
0.945 (0.019) |
0.976 (0.048) |
0.948 (0.002) |
110.958 (7.906) |
0.953 (0.005) |
0.411 (0.037) |
BC-P2-AD |
0.956 (0.026) |
169.130 (11.218) |
0.994 (0.012) |
2.708 (0.210) |
0.982 (0.002) |
134.430 (10.243) |
0.989 (0.025) |
0.373 (0.043) |
BC-P3-AD |
0.945 (0.036) |
121.256 (12.970) |
0.992 (0.012) |
3.019 (0.755) |
0.973 (0.025) |
96.641 (14.836) |
0.985 (0.017) |
0.237 (0.037) |
BC-P1-FDa |
0.998 (0.001) |
137.720 (14.478) |
0.982 (0.001) |
1.012 (0.045) |
0.999 (0.011) |
108.121 (16.996) |
0.999 (0.001) |
0.590 (0.035) |
BC-P1-FDb |
0.820 (0.045) |
6.257 (0.704) |
0.876 (0.035) |
0.274 (0.021) |
0.882 (0.004) |
22.584 (0.844) |
— |
— |
BC-P2-FDa |
0.986 (0.002) |
125.950 (9.912) |
0.971 (0.004) |
1.003 (0.056) |
0.962 (0.010) |
97.623 (5.352) |
0.961 (0.003) |
0.428 (0.026) |
BC-P2-FDb |
0.912 (0.010) |
6.393 (0.354) |
0.863 (0.026) |
0.085 (0.026) |
0.831 (0.002) |
21.276 (0.516) |
— |
— |
BC-P3-FDa |
0.970 (0.004) |
86.000 (2.385) |
0.980 (0.007) |
0.704 (0.027) |
0.990 (0.001) |
68.159 (7.663) |
0.996 (0.001) |
0.290 (0.029) |
BC-P3-FDb |
0.965 (0.023) |
6.115 (0.534) |
0.985 (0.021) |
0.219 (0.039) |
0.976 (0.031) |
21.374 (1.441) |
— |
— |
For freeze-dried BC membrane loaded with ibuprofen, the release of the drug seemed to follow the Higuchi and Korsmeyer–Peppas models. This indicated that the drug release was controlled by diffusion.56 In addition, study of the correlation between swelling and drug release revealed that swelling was also involved in the drug release mechanism for freeze-dried BC membranes. It seemed to have greater diffusion and swelling-controlled at low drug loading concentration (R2 ≈ 0.99) than at high drug loading concentration (R2 ≈ 0.97). The release of drug from BC occurs when the amount of drug exceeds the solubility in the BC and surrounding fluid as Higuchi explained.57,58 Since the n value of the Korsmeyer–Peppas model was about 0.5, it was implied that the diffusion of the drug from the freeze-dried membrane was considered as Fickian diffusion; the drug diffused was gradual and steady.56 At the initial stage (first 15 min) of drug release, since the difference of drug concentration in the membrane and surrounding fluid was high, the diffusion of the drug was rather fast, then sustained release at the following time.
For air-dried BC membrane loaded with propranolol hydrochloride, it tended to release the drug following the first-order kinetics. The release mechanism was similar to ibuprofen loaded, but the release rate constant (K1) was lower. The value of K1 was increased by drug loading concentration, while for ibuprofen loaded, it was rather constant. These results were correlated with the release profiles as shown in Fig. 7.
For freeze-dried BC membrane loaded with propranolol hydrochloride, the release mechanism of the drug at low drug loading concentration tended to approach the zero-order kinetics (R2 > 0.99) as well as Higuchi and Korsmeyer–Peppas models. At low drug-loaded amounts, the BC would be more controllable of the rate of drug release due to the occurrence of high binding efficacy between drug and BC,38,52,53 then the drug gradually released independence with concentration. However, the release mechanism of drug at higher concentrations tended to follow the Higuchi and Korsmeyer–Peppas models rather than zero-order kinetics, indicating that the release of drug was diffusion controlled.56
The release of drug from freeze-dried BC membrane in the latter period, whether loaded with ibuprofen or propranolol hydrochloride, was still more following the Higuchi model than the zero-order or first-order models. However, the release rate constant (KH) was about 3–4 times decreased compared with that at the initial stage. This result could be assumed that the drug loaded BC prepared by freeze-drying method would release the drug by diffusion and swelling control.
Conclusions
This research provided a comprehensive study on the drug release behavior of BC loaded with low or highly soluble drugs. Ibuprofen and propranolol hydrochloride were used as model drugs for low and highly soluble drugs, respectively. Effects of drug loading concentration and drying method used for the drug-loaded BC preparation on characteristics and release behavior of the prepared drug-loaded BC were investigated. It was found that the drug loading concentration significantly affected the amount of drug loaded in the BC for both types of drugs; the amount of drug-loaded increased with increasing drug loading concentration. At low drug loading concentration, the amount of low soluble drug (ibuprofen) was loaded in the BC higher than that of the highly soluble drug but this trend of the result was opposite at high drug loading concentration. Entrapment efficiency (amount of drug in the BC to amount of drug in immersing solution at initial ratio) of low soluble drug tended to decrease but that of highly soluble drug tended to increase with drug loading concentration.
From the drug release study, it was found that the drying method in the preparation of BC loaded with drug influenced BC morphology and swelling played an important role in determining the drug release behavior. By the freeze-drying method, BC loaded with both types of drugs at all drug loading concentrations showed the same drug release behavior that was a sustained release whose release mechanism followed the Higuchi and Korsmeyer–Peppas models. However, the release behavior of drug-loaded BC prepared by the air-drying method was found to be different from that of drug-loaded BC prepared by using freeze-drying method. The immediate release was obtained from both types of drugs at every drug loading concentration except propranolol hydrochloride at the lowest drug loading concentration in which its release behavior was a sustained release. The release mechanism of drug-loaded BC prepared by air-drying method tended to follow first-order kinetics.
Overall conclusion, BC, as a single excipient, can provide immediate or sustained drug release depending on the type of drug used, drug loading concentration, and drying method. The application of BC in drug delivery requires consideration of relevant factors. For example, if a sustained release of highly soluble drugs is desired, the drug-loaded BC should be prepared using the freeze-drying method, however, if the air-drying method is to be used, the drug must be loaded at a low concentration.
Author contributions
CJ: funding acquisition, project administration, conceptualization, methodology, data curation, investigation, visualization, formal analysis, writing – original draft, writing – reviewing and editing; PM: methodology, investigation, formal analysis, writing – reviewing and editing; SS: methodology, visualization, formal analysis, writing – original draft, writing – reviewing and editing; AN: investigation, formal analysis, writing – reviewing and editing; KP: investigation, formal analysis, writing – reviewing and editing. All authors approved the final version of the manuscript before submission.
Conflicts of interest
There are no conflicts to declare.
Acknowledgements
The authors would like to thank Walailak University for financial support (grant number WU-IRG-62-014).
References
- S. P. Lin, I. L. Calvar and J. M. Catchmark, et al., Cellulose, 2013, 20(5), 2191–2219 CrossRef CAS.
- D. T. Reis, A. K. D. S. Pereira and G. N. Scheidt, et al., Orbital: Electron. J. Chem., 2019, 11(5), 321–329 CAS.
- M. M. Abeer, M. C. I. M. Amin and C. Martin, J. Pharm. Pharmacol., 2014, 66(8), 1047–1061 CrossRef CAS PubMed.
- V. Raghavendran, E. Asare and I. Roy, Adv. Microb. Physiol., 2020, 77, 89–138 CrossRef PubMed.
- H. Ullah, H. A. Santos and T. Khan, Cellulose, 2016, 23(4), 2291–2314 CrossRef CAS.
- I. F. Almeida, T. Pereira and N. Silva, et al., Eur. J. Pharm. Biopharm., 2014, 86(3), 332–336 CrossRef CAS PubMed.
- F. G. Torres, S. Commeaux and O. P. Troncoso, J. Funct. Biomater., 2012, 3(4), 864–878 CrossRef CAS PubMed.
- N. H. Silva, J. P. Mota and T. S. D. Almeida, et al., Int. J. Mol. Sci., 2020, 21(4), 1262 CrossRef CAS PubMed.
- J. Kucińska-Lipka, I. Gubanska and H. Janik, Polym. Bull., 2015, 72(9), 2399–2419 CrossRef.
- W. Treesuppharat, P. Rojanapanthu and C. Siangsanoh, et al., Biotechnol. Rep., 2017, 15, 84–91 CrossRef CAS.
- M. L. Cacicedo, G. A. Islan and M. F. Drachemberg, et al., New J. Chem., 2018, 42(9), 7457–7467 RSC.
- F. A. Ngwabebhoh, R. Patwa and O. Zandraa, et al., J. Drug Delivery Sci. Technol., 2021, 63, 102415 CrossRef CAS.
- M. L. Cacicedo, G. Pacheco and G. A. Islan, et al., Int. J. Biol. Macromol., 2020, 147, 1136–1145 CrossRef CAS PubMed.
- G. Juncu, A. Stoica-Guzun and M. Stroescu, et al., Int. J. Pharm., 2016, 510(2), 485–492 CrossRef CAS PubMed.
- R. D. Pavaloiu, A. Stoica-Guzun and M. Stroescu, et al., Int. J. Biol. Macromol., 2014, 68, 117–124 CrossRef CAS PubMed.
- M. C. I. M. Amin, N. Ahmad and N. Halib, et al., Carbohydr. Polym., 2012, 88(2), 465–473 CrossRef.
- D. Plackett, K. Letchford and J. Jackson, et al., Nord. Pulp Pap. Res. J., 2014, 29(1), 105–118 CrossRef CAS.
- E. Trovatti, C. S. Freire and P. C. Pinto, et al., Int. J. Pharm., 2012, 435(1), 83–87 CrossRef CAS PubMed.
- M. Badshah, H. Ullah and S. A. Khan, et al., Cellulose, 2017, 24(11), 5041–5052 CrossRef CAS.
- S. Adepu and M. Khandelwal, Carbohydr. Polym., 2020, 249, 116816 CrossRef CAS PubMed.
- R. Jonas and L. F. Farah, Polym. Degrad. Stab., 1998, 59(1–3), 101–106 CrossRef CAS.
- S. Gorgieva and J. Trček, Nanomaterials, 2019, 9(10), 1352 CrossRef CAS PubMed.
- P. Wanichapichart, S. Kaewnopparat and K. Buaking, et al., Songklanakarin J. Sci. Technol., 2002, 24, 855–862 CAS.
- J. Juntaro, S. Ummartyotin and M. Sain, et al., Carbohydr. Polym., 2012, 87(4), 2464–2469 CrossRef CAS.
- N. Chunshom, P. Chuysinuan and S. Techasakul, et al., J. Sci.: Adv. Mater. Devices, 2018, 3(3), 296–302 Search PubMed.
- A. J. Silvestre, C. S. Freire and C. P. Neto, Expert Opin. Drug Delivery, 2014, 11(7), 1113–1124 CrossRef CAS PubMed.
- F. O. Costa, J. J. S. Sousa and A. A. C. C. Pais, et al., J. Controlled Release, 2003, 89(2), 199–212 CrossRef CAS.
- J. Sahoo, P. N. Murthy and S. Biswal, et al., AAPS PharmSciTech, 2008, 9(2), 577–582 CrossRef CAS PubMed.
- International Conference on Harmonisation of Technical Requirements for Registration of Pharmaceuticals for Human Use, ICH Harmonised Tripartite Guideline—Validation of Analytical Procedures: Text and Methodology Q2(R1), Current Step 4 version, 2005 Search PubMed.
- T. Hayashi, H. Kanbe and M. Okada, et al., Int. J. Pharm., 2005, 304(1–2), 91–101 CrossRef CAS PubMed.
- K. Löbmann and A. J. Svagan, Int. J. Pharm., 2017, 533(1), 285–297 CrossRef.
- M. Zeng, A. Laromaine and A. Roig, Cellulose, 2014, 21(6), 4455–4469 CrossRef CAS.
- N. Chunshom, P. Chuysinuan and S. Techasakul, et al., J. Sci.: Adv. Mater. Devices, 2018, 3(3), 296–302 Search PubMed.
- C. C. Sun, J. Pharm. Sci., 2005, 94(10), 2132–2134 CrossRef CAS PubMed.
- M. M. Lübtow, M. S. Haider and M. Kirsch, et al., Biomacromolecules, 2019, 20(8), 3041–3056 CrossRef PubMed.
- M. Badshah, H. Ullah and A. Khan, et al., Surface modification and evaluation of bacterial cellulose for drug delivery, Int. J. Biol. Macromol., 2018, 113, 526–533 CrossRef CAS PubMed.
- M. Ul-Islam, W. A. Khattak and M. Kang, et al., Cellulose, 2013, 20(1), 253–263 CrossRef CAS.
- B. V. Mohite, R. K. Suryawanshi and S. V. Patil, Cellul. Chem. Technol., 2016, 50, 219–223 CAS.
- A. H. Tayeb, E. Amini and S. Ghasemi, et al., Molecules, 2018, 23(10), 2684 CrossRef PubMed.
- S. C. Lazarini, R. de Aquino and A. C. Amaral, et al., Cellulose, 2016, 23(1), 737–748 CrossRef CAS.
- I. Reiniati, A. N. Hrymak and A. Margaritis, Crit. Rev. Biotechnol., 2017, 37(4), 510–524 CrossRef CAS PubMed.
- Y. Ryabenkova, N. Jadav and M. Conte, et al., Langmuir, 2017, 33(12), 2965–2976 CrossRef CAS PubMed.
- A. Martinez-Felipe, F. Brebner and D. Zaton, et al., Molecules, 2018, 23(9), 2278 CrossRef PubMed.
- M. Saeedi, K. Morteza-Semnani and M. Sagheb-Doust, Acta Pharm., 2013, 63(1), 99–114 CAS.
- S. Takka, Farmaco, 2003, 58(10), 1051–1056 CrossRef CAS PubMed.
- R. L. Oliveira, J. G. Vieira and H. S. Barud, et al., J. Braz. Chem. Soc., 2015, 26(9), 1861–1870 CAS.
- K. V. Drozd, A. N. Manin and A. V. Churakov, et al., CrystEngComm, 2017, 19(30), 4273–4286 RSC.
- E. Tsouko, C. Kourmentza and D. Ladakis, et al., Int. J. Mol. Sci., 2015, 16(7), 14832–14849 CrossRef CAS PubMed.
- C. M. Lee, J. Gu and K. Kafle, et al., Carbohydr. Polym., 2015, 133, 270–276 CrossRef CAS PubMed.
- M. Sarkar, O. P. Perumal and R. Panchagnula, Indian J. Pharm. Sci., 2008, 70(5), 619 CrossRef PubMed.
- M. P. Illa, C. S. Sharma and M. Khandelwal, J. Mater. Sci., 2019, 54(18), 12024–12035 CrossRef CAS.
- R. D. Pavaloiu, A. Stoica and M. Stroescu, et al., Cent. Eur. J. Chem., 2014, 12(9), 962–967 CAS.
- T. G. Volova, A. A. Shumilova and I. P. Shidlovskiy, et al., Polym. Test., 2018, 65, 54–68 CrossRef CAS.
- M. Pandey, M. C. I. M. Amin and N. Mohamad, et al., Polym.-Plast. Technol. Eng., 2013, 52(14), 1510–1518 CrossRef CAS.
- M. Pandey, M. C. I. M. Amin and N. Ahmad, et al., Int. J. Polym. Sci., 2013, 905471 Search PubMed.
- M. P. Paarakh, P. A. Jose and C. Setty, et al., Int. J. Pharm. Res. Technol., 2018, 8(1), 12–20 Search PubMed.
- D. R. Paul, Int. J. Pharm., 2011, 418(1), 13–17 CrossRef CAS PubMed.
- J. H. Petropoulos, K. G. Papadokostaki and M. Sanopoulou, Int. J. Pharm., 2012, 437(1–2), 178–191 CrossRef CAS PubMed.
Footnote |
† Electronic supplementary information (ESI) available. See DOI: 10.1039/d1ra07761a |
|
This journal is © The Royal Society of Chemistry 2021 |