DOI:
10.1039/D1RA07534A
(Paper)
RSC Adv., 2021,
11, 36707-36718
Effect of diluent on the extraction of europium(III) and americium(III) with N,N,N′,N′-tetraoctyl diglycolamide (TODGA)
Received
12th October 2021
, Accepted 8th November 2021
First published on 16th November 2021
Abstract
Solvent extraction of Eu3+ and Am3+ via N,N,N′,N'-tetraoctyl diglycolamide (TODGA) dissolved in different molecular diluents was studied. The diluent types used in this work were primary and secondary alcohols, secondary ketones and alkanes. Effects of concentration of extracting agent, temperature, diluent type and its carbon chain length on the extractions were determined. Distribution ratios of Eu3+ and Am3+ showed high dependence on the diluent type as well as the carbon chain length within the same type of diluent. The highest distribution ratios for both Eu3+ and Am3+ as well as the separation factors of Eu3+ over Am3+ were observed in the alkane diluents. Unexpectedly high distribution ratios for Eu3+ and Am3+ were observed in polar diluents with 5 carbon atoms in the chain, clearly standing out against the general trends. It was found that Eu3+ and Am3+ extraction via TODGA is enthalpy driven in all the studied diluents and that extraction is more exothermic in alkane diluents. Analysis of the stoichiometry of the extracted complexes shows that the average ligand number of TODGA molecules in the extracted complex is lower for Am3+ compared to Eu3+ except for with alkane diluents.
1 Introduction
Solvent extraction is a separation technique commonly used in many various industries including mining and nuclear fuel reprocessing, as well as being one of the foreseen methods for recycling rare earth elements from nickel-metal hydride batteries, permanent magnets or modern electronics.1–4 Its ease of use for industrial application offers efficient application and high purity of extracted metal. A tremendous effort during the last decades has been put into the development of new, more selective, extracting agents for the extraction processes. At the same time basic phenomena in the particular solvent extraction processes are poorly understood. One of them is the effect of the diluent on the extraction process. Diluent is defined as: “the liquid or homogeneous mixture of liquids in which extractant(s) and possible modifier(s) may be dissolved to form the solvent phase.”5 The organic diluent is the major constituent of the organic phase in the solvent extraction systems. It was already shown in the past that diluent has a substantial effect on the outcome of the extraction with respect to both distribution ratios as well as separation factors between different elements.6–15 Despite the strong experimental evidence of diluent effect, its full theoretical explanation is challenging and predictions are still very uncertain. Few theoretical explanations for the diluent effect were proposed. The first introduction of diluent effect to the predictions of distribution ratios in solvent extraction was proposed by Schmidt.15 An empirical diluent parameter was included to predict the distribution ratios of zinc extracted by tri-n-octyl-amine in different diluents. Unfortunately, the proposed predictions were valid only for a limited number of diluents even with only one extracting agent. Another explanation of diluent effect is based on the energetic balance between the cost of cavity formation within the diluent into which the extracted molecule is placed and the energetic gain from interaction between the diluent and the extracted molecule.3,16 In order to get a deeper understanding into the energetic cost of the cavity creation Hildebrand used the heat of vaporization to predict the cohesive forces within liquids. The model is based on the proportionality of the heat of vaporization to the intermolecular binding energy.17,18 This idea was further developed by Hansen dividing the solubility parameter into three vectors consisting of the hydrogen bonding, polar and dispersion interactions.19 Hansen's model has been successfully applied on mutual solubility of polymers in solvents or plasticizers. However, in solvent extraction, simple correlations of extraction constants (Kd) against solubility parameters are not sufficient for predictions of the extraction outcome.20 At the same time systematic experimental studies on the diluent effect are very scarce.21
In order to further investigate the effects of diluent on extraction, the aim of this study is the systematic evaluation of the molecular diluent effect on the extraction of trivalent europium and americium from nitric acid solutions with N,N,N′,N′-tetraoctyl diglycolamide as an extracting agent. The family of diglycolamides are known extracting agents for trivalent lanthanides and actinides in solvent extraction, with expected application in partitioning and transmutation of a nuclear fuel22–25 and in radiopharmaceutical industry for 225Ac production.26–29 While the behavior of diglycolamides with respect to equilibrium distribution ratios, acid extraction and kinetics was extensively evaluated,30–42 the effect of the diluent on the extraction was evaluated only in a few studies.42–47 From the available data, correlations of diluent properties against the distribution ratios seems rather difficult. It was shown that in nonpolar diluents benzene rings give decrease in the extraction of americium.32,43 At the same time, distribution ratios of americium and lanthanides in the polar nitrobenzene media are high.32,48
Correlations of distribution ratios against dielectric constants of used diluents seem to be also non-conclusive.49 Dielectric constant of the diluent appears to have effect on the stoichiometry of the extracted americium complex giving lower ligand number in diluents with higher dielectric constants.32,43,44,48,49 In the diluents with higher polarity (e.g. dichloroethane, octanol) 1
:
2 metal
:
TODGA complex was observed whereas in low polarity diluents (e.g. chloroform, toluene, dodecane) 1
:
3 or 1
:
4 metal
:
TODGA molecules were detected.43,44 At the same time it was shown that the nature of the diluent does not affect the results of time resolved laser fluorescence measurements luminescence spectra of Eu3+.38,45
In order to bring deeper insight into diluent effects, we performed a systematic study of the molecular diluent influence on solvent extraction of americium and europium by N,N,N′,N′-tetraoctyl diglycolamide (TODGA) from nitric acid media. Three different groups of diluents consisting of alkanes, long chained alcohols and ketones were studied. The effect of the carbon chain length, was evaluated from 5 to 10 carbons in order to target the subtle changes in the polarity of used diluents. Moreover, standard molar Gibbs energy (ΔG0298 K), enthalpy (ΔH0298 K), and entropy (ΔS0298 K) changes for the extraction of Eu3+ and Am3+ from 4 M HNO3 into TODGA dissolved in different diluents was studied to get better understanding of thermodynamic effect of molecular diluent on these solvent extraction processes.
2. Experimental
2.1. Organic and aqueous phase preparation
Aliphatic organic diluents (ACS grade, ≥99.0%) were obtained from Sigma Aldrich and were used as supplied. TODGA (>99% by HPLC) was obtained from Technocomm Ltd (Scotland). Organic phases for experiments were prepared volumetrically by mixing TODGA with various diluents. For the primary and secondary alcohols as well as ketones the TODGA concentration in the organic phase was 20 mmol L−1. For the alkane diluents the TODGA concentration was 2 mmol L−1. Aqueous phases were prepared by gravimetrical dilution (Sartorius, 98648-018-34) of 65 volume% HNO3 (purris. p.a. grade, J.T. Baker) with deionized water (18 MΩ cm at 25 °C from Milli-Q system) to 4 mol L−1. The aqueous phases were spiked with trace amounts of radioactive 152Eu and 241Am (approximately 10−7 mol L−1 and 10−8 mol L−1, respectively) obtained from PSI stocks. The complete composition of extraction systems and extraction conditions studied in this work are shown in the Table 1.
Table 1 Composition of extraction systems and extraction conditions used
Diluent family |
Length of the carbon chain |
TODGA concentration (mmol L−1) |
Temperature (K) |
Nitric acid concentration (mol L−1) |
Alkanes |
6–10 |
1, 2, 5 |
288, 298, 308 |
4 |
Primary alcohols |
5–10 |
5, 20, 40 |
288, 298, 308 |
4 |
Secondary alcohols |
5–10 |
5, 20, 40 |
288, 298, 308 |
4 |
Secondary ketones |
5–10 |
5, 20, 40 |
288, 298, 308 |
4 |
2.2. Measurements of distribution ratios
The distribution ratios for the trace amounts of 152Eu and 241Am were determined by contacting 1 mL of the aqueous phase containing 4 mol L−1 HNO3 (as the representative HNO3 concentration in the high active raffinate solution50) and europium and americium tracers with the same volume of organic phase containing TODGA dissolved in respective diluent. Contacting was done by the shaking of the organic and aqueous phases for 120 minutes in shaking machine (IKA Vibrax VXR Basic) at 1000 rpm. This time (120 min) was determined in the initial tests to be sufficient in order to reach distribution equilibrium for both europium and americium. Shaking machine was equipped with sample holder coupled with a heated circulating water bath (Julabo, F250) to maintain constant temperature. After the contacting, the samples were allowed to settle by gravity for 120 minutes. After phase separation, 500 μL and 800 μL aliquots were taken from organic and aqueous phases, respectively. Aqueous phase (800 μL) was transferred to the second vial from which a second 500 μL aliquot was taken in order to avoid cross contamination with the organic phase. The samples were kept in the temperature-controlled setup from the phase contacting stage till the first sampling of 500 μL and 800 μL aliquots for organic and aqueous phases respectively. This decreases the possible experimental errors in the determination of the extraction constants that are highly temperature sensitive.
After the sampling, count-rates of 152Eu at 121.8 keV and 241Am at 59.6 keV in aqueous and organic phases were measured via a p-type High Purity Germanium detector (HPGe) coupled with digital spectrum analyzer. Genie 2000 software was used to analyze obtained gamma spectra. Dead time during the data acquisition was kept below 5%. The effect of density differences between the aqueous and organic phases on transmission of γ-rays from the samples was evaluated by EFFTRAN code51 and was found to be less than 0.5% thus below the random uncertainty originating from the counting statistics. The extraction of americium and europium by pure diluent in the absence of TODGA was investigated in the screening experiments which were performed in the same manner as experiments with TODGA described above.
The distribution ratios were calculated as the ratio of equilibrium concentration of solute (Eu3+ and Am3+) in the organic phase over its concentration in the aqueous phase represented by its measured radioactivity (eqn (1)).
|
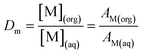 | (1) |
The separation factors were subsequently calculated as the ratio of the distribution ratios of europium and americium as shown in eqn (2)
|
 | (2) |
Experiments were performed in duplicates or triplicates and the uncertainties of all data are given as one standard deviation.
It is well known that extraction of HNO3 via some organic phases containing TODGA occurs.33,38–41,49,52 Therefore, two sets of experiments were performed – with the organic phase pre-saturated with 4 M HNO3 as well as with a non-preconditioned set of diluents. Saturation of the organic solvent (diluent and TODGA) was performed by contacting the solvent with the same volume of 4 M HNO3 by shaking machine for 60 minutes and then the phases were let to separate by gravity for 120 minutes. This procedure was performed only with polar diluents, as alkanes did not show any appreciable extraction of nitric acid thus behaving like true inert diluents.
2.3. Extraction of nitric acid into diluent
In order to obtain insight into the ability of the pure diluent to extract nitric acid from the aqueous solutions experiments were performed in the similar way as described in the work by Geist.53 Organic diluent (25 mL) was contacted with the equal volume of water and shook for 30 minutes. Phases were separated by centrifugation and 25 mL of organic phase was then transferred and contacted with the equal volume of nitric acid solutions of varied concentration (0.1–4 mol L−1) in shaking machine at 2000 rpm.
After subsequent centrifugation, 20 mL of the organic phase was transferred to the different vial and contacted with distilled MQ water in order to back-extract nitric acid from the organic phase. The acid concentration in the back-extracted solutions as well as in the initial aqueous solutions was determined by potentiometric titrations using Metrohm Titrino and 0.01 mol L−1 and 0.1 mol L−1 standard NaOH solutions. These experiments were performed in triplicates. The mass balance calculations showed that single contacting of the loaded organic phase with distilled water is sufficient for the complete back-extraction of HNO3 into aqueous phase.
2.4. Determination of the stoichiometry of the extracted species
The stoichiometry of extracted complexes with respect to average number of TODGA ligand surrounding the extracted metal was determined by the conventional slope analysis. The slope analysis was performed by the least squares linear regression of the logarithmic plots of distribution ratios against the logarithmic values of TODGA concentration in the organic phase for each diluent. As only trace amounts of Eu3+ and Am3+ were used in the tests (10−7 mol L−1 and 10−8 mol L−1, respectively), the concentration of the free TODGA at the equilibrium was assumed to be equal to its initial value. For the calculations of apparent extraction constants, activities in the aqueous phase rather than concentrations were used. Extended Specific ion Interaction Theory (ESIT) developed by Ciavatta34 was used to compute activity coefficients of free nitrate ions in aqueous phase. According to the mechanism of Eu and Am extraction via TODGA (eqn (4) below), only neutral, uncharged species exist in organic phase. It can be assumed that activity coefficients of neutral species are equal to one,54 therefore concentrations of M(NO3)n(TODGA)m(org) were used for all computations.
In order to interpret the linear regression results following assumptions were applied:
- Tracer amounts of Am3+ and Eu3+ don't contribute to the ionic strength.
- Eu3+ and Am3+ are not hydrolyzed in 4 mol L−1 HNO3.55
- Activity coefficients for neutral species are equal to one.54
There is some evidence from experimental spectroscopic data (mostly Raman and Infra-Red), Nuclear Magnetic Resonance data and molecular dynamic simulations for the existence of weak HNO03 ion pairs in relativity concentrated aqueous solutions of nitric acid.56–61 Comprehensive review of the experimental data is available62 and according to this review values of HNO03 stability (dissociation) constants at infinite dilution (K0a) vary significantly, from 10 to 40. Such a large difference in K0a can be explained by the problems associated with experimental or theoretical determination of stability constants of weak ion pairs and strongly indicates that uncertainty associated with this value is large.63,64 In this work apparent stability constant of HNO03 at 4 mol L−1 (Ka) and its 1σ standard deviation was estimated based on literature data as 25 ± 10. This value is in a good agreement with most of the literature values discussed by Levanov and co-workers.62 The degree of HNO3 dissociation at 4 mol L−1 (α) can be calculated from estimated dissociation constant (Ka = 25) using Ostwald dilution law and it can be shown that 88% of HNO3 is dissociated.
The assumed extraction mechanism is presented in eqn (3):30
|
M(aq)n+ + 3NO3(aq)− + mTODGA(org) ↔ M(NO3)3(TODGA)m(org)
| (3) |
where
m (number of TODGA molecules) in the extracted specie was determined by slope analysis.
The apparent equilibrium extraction constant (Kex) for the reaction in eqn (3) is defined as:
|
 | (4) |
Activity coefficients of free nitrate in the aqueous phase were calculated with extended SIT theory according to ref. 65:
|
 | (5) |
where
ε(H
+, NO
3−) = 0.07.
66
Thus when combined with distribution ratio (eqn (1)) the equation can be rearranged into the form of eqn (6).
|
 | (6) |
For the polar diluents, TODGA concentration of 5, 20 and 40 mmol L−1 were used, whereas for alkanes concentration of TODGA was 1, 2 and 5 mmol L−1. All the extraction experiments for stoichiometry determination were performed at 298 ± 0.2 K. For the free nitrate concentration in the aqueous phase equilibrium values obtained from the nitric acid extraction experiments were used.
The activity coefficients of TODGA in the organic diluents taken to be equal to unity in this work. However, the transport of water, nitric acid and nitrate groups into the organic phase during the contacting could possibly lead to the binding of the free TODGA ligand. Therefore, the determined stoichiometry from the slope analysis has only limited validity within the range of tested TODGA concentrations. The uncertainties of the linear regression are presented as one standard deviation.
2.5. Determination of the thermodynamic parameters
Solvent extraction of Am3+ and Eu3+ via TODGA was studied at different temperatures (288 K, 298 K and 308 K). Distribution ratios determined at these temperatures were used to compute extraction constant (Kex). For the calculation of Kex in different diluents the stoichiometry of Eu(Am):TODGA complexes for each type of diluent derived from slope analysis presented in the Table 3 were used. Natural logarithm of Kex was plotted as a function of temperature (T−1) and standard enthalpy change and the standard entropy change were derived from this plot (Van't Hoff's plot) using eqn (7). The standard change of Gibbs energy was thereafter calculated using eqn (8). |
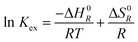 | (7) |
3. Results and discussion
3.1. Distribution ratios and separation factors
Experiments with pure diluents without TODGA showed that extraction of europium or americium into pure diluent doesn't occur.
The obtained distribution ratios for Eu3+ and Am3+ in the different pristine diluents at 298 ± 0.2 K are presented in Fig. 1A and B, respectively. The results for the diluents pre-saturated with acid are shown in Fig. 2A and B. As it is shown in Fig. 1A and B, and Fig. 2A and B in extraction experiments performed with TODGA dissolved in polar diluents, the distribution ratios of both europium and americium showed significant dependence on the diluent type as well as carbon chain length. This behavior was observed in both pre-conditioned as well as pristine solvents. Significant increase of the distribution ratios was observed in diluents with 5 carbon atoms in chain, regardless of the diluent type used. Concentration of TODGA in alkane diluents was kept 2 mmol L−1 in order to keep D-ratios below 150. In the case of alkanes, pentane was not used as diluent due to its low viscosity.
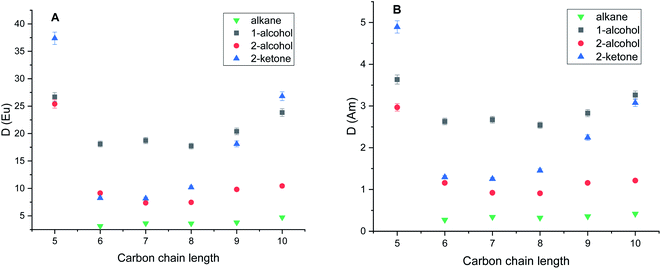 |
| Fig. 1 Dependence of distribution ratios of Eu3+ (A) and Am3+ (B) on the diluent type and its carbon chain length in pristine solvents. The concentration of TODGA was 20 mmol L−1 for polar diluents and 2 mmol L−1 for alkanes. | |
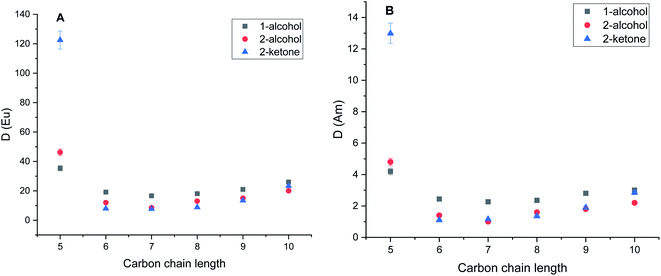 |
| Fig. 2 Dependence of distribution ratios of Eu3+ (A) and Am3+ (B) on the diluent type and its carbon chain length in solvents, pre-saturated with HNO3. | |
It is clear from Fig. 1A and B that the type of diluent as well as the length of the carbon chain have a substantial effect on the distribution ratio of europium and americium. Generally, the distribution ratio in polar diluents increases with the increase of the carbon chain length except for chain with 5 carbon atoms. Carbon chain length has a profound effect in the case of ketone diluents. It is note-worth to point out on the difference between primary and secondary alcohols with respect to distribution ratios while position of the hydroxyl group further in carbon chain leads to the significant decrease of the distribution ratio. In the case of alkane diluents, the change of the distribution ratios was insignificant with the increase of the carbon chain length. In solvents pre-saturated with nitric acid a slight increase of the distribution ratio was observed while penta-2-one showed significant increase in distribution ratios for both Am3+ and Eu3+. The increase of distribution ratios can be addressed to the higher free nitrate concentration in the aqueous phase at the extraction equilibrium. However, the trends regarding the diluent type as well as the carbon chain were the same as in pristine solvents. The separation factors between Eu3+ and Am3+ presented in the Fig. 3 show a similar behavior as the distribution ratios of Eu and Am with respect to their dependence on carbon chain length.
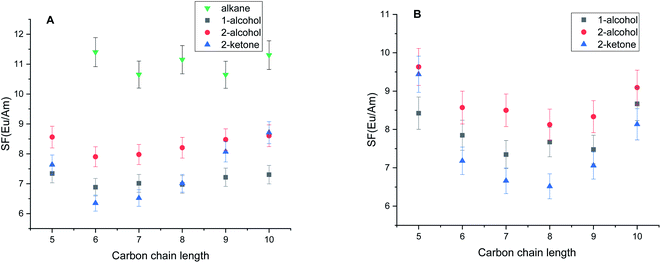 |
| Fig. 3 The dependence of separation factors between Eu3+ and Am3+ on the diluent type and its carbon chain length (A) pristine solvent (B) pre-saturated solvent. The concentration of TODGA was 20 mmol L−1 for polar diluents and 2 mmol L−1 for alkanes. | |
The observed separation factors in the secondary alcohols were higher for all carbon chain lengths when compared to primary alcohols despite aforementioned lower distribution ratios. We observed the significant increase in the separation factors in the alkane diluents when compared to the polar ones. In alkane diluents, the separation factor did not show statistically significant variations with the increase of carbon chain unlike in other diluent types. In solvents pre-saturated with nitric acid slight increase of separation factors for Eu3+ from Am3+ was observed in all three polar diluent families while maintaining the similar visual trend over the carbon chain length as in non-conditioned solvents.
3.2. Extraction of nitric acid into the diluent
The extraction of nitric acid into various diluents is shown in Fig. 4. Alkane diluent type is omitted in presented results, as this diluent type did not extract nitric acid and behaves like an inert diluent. As shown in Fig. 4, the extraction of nitric acid into organic phase always increases with its initial concentration in aqueous phase, and decreases with the carbon chain length of the diluent. The non-linear behavior (Fig. 4) could be an effect of the organic phase dielectric properties changes due to the pre-saturating of the diluent with the water before the nitric acid introduction. The water extraction by diluents was clearly visible when following the water level in graduated cylinder during contacting, however not quantified by i.e. Karl Fischer titrations. The data for the water absorption quantities in the pure diluents are known and available from the IUPAC compilations.67–74
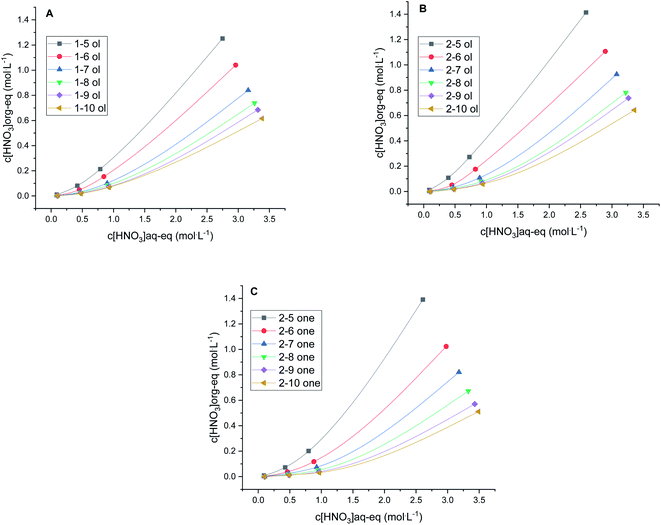 |
| Fig. 4 Equilibrium concentrations of nitric acid in organic phase against its equilibrium concentration in the aqueous phase (A) primary alcohols, (B) secondary alcohols, (C) secondary ketones. Full lines in plots are only for the eye guidance. | |
Higher concentrations of nitric acid in organic phase may result in change of intermolecular interactions and thus contribute to deviation from linearity. Therefore, it cannot be assumed that the same forces will act on the nitric acid in the case of different diluents.
While differences of acid extraction between primary and secondary alcohols are minor (if any), ketones with more than 6 carbon atoms in the alkyl chain extract significantly less nitric acid than alcohol diluents. For example the equilibrium concentration of nitric acid in the organic phase is ca. 11% lower for 2-heptanone when compared to 2-heptanol and 20% lower in case of 2-decanone versus 2-decanol. The lower concentration of nitric acid in long carbon chained ketones yielding to lower dielectric constant of the saturated diluent. This behavior might explain the increase of the distribution ratios for Eu3+ and Am3+ for the longer carbon chained ketones as shown in Fig. 1. The lower extraction of nitric acid is presumably caused by the inability of longer carbon chain ketones to act as a hydrogen bonding donors. In general, the extraction of acid followed the order according to alkyl chain length 5 > 6 > 7 > 8 > 9–10 for all diluent types. Equilibrium concentrations of nitric acid in the aqueous phase after contacting with each diluent was determined and are listed in the Table 2.
Table 2 Equilibrium concentrations of nitric acid in the aqueous phase after contacting 4 mol L−1 HNO3 solution with different diluents
Diluent |
C(eq)HNO3(aq) (mol L−1) |
Diluent |
C(eq)HNO3(aq) (mol L−1) |
Diluent |
C(eq)HNO3(aq) (mol L−1) |
1-Pentanol |
2.75 ± 0.03 |
2-Pentanol |
2.59 ± 0.03 |
2-Pentanone |
2.60 ± 0.03 |
1-Hexanol |
2.96 ± 0.03 |
2-Hexanol |
2.90 ± 0.03 |
2-Hexanone |
2.98 ± 0.03 |
1-Heptanol |
3.16 ± 0.03 |
2-Heptanol |
3.09 ± 0.03 |
2-Heptanone |
3.19 ± 0.03 |
1-Octanol |
3.25 ± 0.03 |
2-Octanol |
3.22 ± 0.03 |
2-Octanone |
3.33 ± 0.03 |
1-Nonanol |
3.31 ± 0.03 |
2-Nonanol |
3.27 ± 0.03 |
2-Nonanone |
3.42 ± 0.03 |
1-Decanol |
3.39 ± 0.03 |
2-Decanol |
3.36 ± 0.03 |
2-Decanone |
3.43 ± 0.03 |
3.3. Stoichiometry of the extracted species
The stoichiometry of the extracted species were determined by the slope analysis of the distribution ratios plotted against the TODGA concentration in the organic phase in the logarithmic scale. By this method the apparent stoichiometry of the extraction reaction was determined with respect to the TODGA molecules surrounding the extracted metal complex. The obtained results are presented in the Table 3.
Table 3 Values of slopes from log–log plots of the extraction of Eu3+ and Am3+ from 4 M HNO3 by TODGA in various organic diluents. Uncertainties are given as two standard deviations. Pearson's R was >0.99 for all performed regressions
Diluent |
Eu3+ : TODGA |
Am3+ : TODGA |
Diluent |
Eu3+ : TODGA |
Am3+ : TODGA |
Hexane |
3.40 ± 0.05 |
3.40 ± 0.05 |
2-Pentanol |
2.82 ± 0.02 |
2.46 ± 0.02 |
Heptane |
3.7 ± 0.1 |
3.7 ± 0.1 |
2-Hexanol |
2.81 ± 0.02 |
2.43 ± 0.02 |
Octane |
3.7 ± 0.1 |
3.7 ± 0.1 |
2-Heptanol |
2.80 ± 0.02 |
2.44 ± 0.03 |
Nonane |
3.5 ± 0.1 |
3.4 ± 0.1 |
2-Octanol |
2.80 ± 0.01 |
2.44 ± 0.04 |
Decane |
3.3 ± 0.1 |
3.3 ± 0.1 |
2-Nonanol |
2.83 ± 0.05 |
2.46 ± 0.02 |
|
|
|
2-Decanol |
2.82 ± 0.03 |
2.53 ± 0.03 |
Diluent |
Eu3+ : TODGA |
Am3+ : TODGA |
Diluent |
Eu3+ : TODGA |
Am3+ : TODGA |
1-Pentanol |
2.80 ± 0.01 |
2.35 ± 0.03 |
2-Pentanone |
2.84 ± 0.01 |
2.40 ± 0.05 |
1-Hexanol |
2.74 ± 0.01 |
2.28 ± 0.02 |
2-Hexanone |
2.84 ± 0.09 |
2.40 ± 0.05 |
1-Heptanol |
2.74 ± 0.005 |
2.32 ± 0.02 |
2-Heptanone |
2.90 ± 0.05 |
2.40 ± 0.05 |
1-Octanol |
2.74 ± 0.005 |
2.25 ± 0.02 |
2-Octanone |
3.00 ± 0.05 |
2.50 ± 0.05 |
1-Nonanol |
2.77 ± 0.01 |
2.31 ± 0.02 |
2-Nonanone |
3.00 ± 0.02 |
2.50 ± 0.05 |
1-Decanol |
2.86 ± 0.01 |
2.33 ± 0.02 |
2-Decanone |
3.00 ± 0.03 |
2.50 ± 0.05 |
The obtained data indicate that stoichiometry of extracted europium and americium complexes differ from each other in all the types of polar diluents. However, the apparent stoichiometry does not variate significantly with the change of the carbon chain length within the same diluent type. The slope analysis shows that Eu3+ is extracted predominantly as 1
:
3 complex whereas Am3+ as a mix of 1
:
2 and 1
:
3 complexes in the polar diluents. The determined stoichiometry in alkane diluents indicates extraction of 1
:
3 and 1
:
4 complexes within the presented concentration range of TODGA for both Eu3+ and Am3+. In general, the observed values are consistent with the previously reported stoichiometries of extracted complexes of An3+ and Ln3+ with diglycolamides.38,45,75–78 Its noteworthy that 1
:
4 coordination, although observed also in other works30,38,79 is not possible within the first solvation shell of Eu3+ and Am3+ due to geometrical hindering.80 As spectroscopic methods i.e. Time Resolved Laser Fluorescence Spectroscopy (TRLFS) target the inner coordination sphere of the extracted ion the outer sphere coordination remains unresolved. One can therefor speculate about the explanation of the observed effect due to additional complexation by TODGA molecule in the second solvation shell81 or as the consequence of TODGA aggregation into the form of micelles.82
3.4. Thermodynamic parameters
The extraction of europium as well as of americium was exothermic in all tested diluents. In alkane diluents, the decrease of distribution ratios with increasing temperature was the steepest. This behavior is in agreement with previously reported results.44,75 The derived thermodynamic parameters for the extraction of Eu3+ and Am3+ from 4 mol L−1 HNO3 by TODGA dissolved in various diluents are shown in Tables 4 and 5. The values of nitrate concentrations listed in Table 2 were used to calculate activity coefficients of free nitrate using extended SIT. In general, the observed values show similar behavior as in the previously published studies mainly with respect to exothermic behavior and negative entropy change values.75,83,84 From the derived thermodynamic parameters, the Gibbs energy change (−ΔG0) follows the order alkanes ≫ primary alcohols ≈ secondary alcohols ≈ ketones. However the change of enthalpy of extractions shows difference between primary or secondary alcohols and ketones, where the latter show smaller exothermic nature of extraction. This also leads to the different (ΔH0/ΔS0) ratios for each diluent family probably according to the disturbances in the structure of diluents during the extraction process. This explanation is feasible when taking in account more ordered structure of alcohol diluents when compared to ketones, due to the ability alcohols to form hydrogen bonds within the diluent.
Table 4 Standard molar Gibbs energy ΔG0298 K enthalpy ΔH0298 K, and entropy ΔS0298 K changes and natural logarithm of apparent extraction constants for the extraction of Eu3+ in tested systems. In polar diluents, concentration of TODGA was 20 mmol L−1, whereas in alkanes 2 mmol L−1 concentration of TODGA was used
Diluent |
ln Kex298 K |
ΔG0298 K (kJ mol−1) |
ΔH0298 K (kJ mol−1) |
ΔS0298 K [J (mol K)−1] |
1-Pentanol |
11.6 ± 0.5 |
−28.6 ± 2.0 |
−41.8 ± 2.1 |
−44.2 ± 2.2 |
1-Hexanol |
10.6 ± 0.4 |
−26.3 ± 1.9 |
−40.4 ± 2.0 |
−47.3 ± 2.4 |
1-Heptanol |
10.4 ± 0.4 |
−25.7 ± 1.8 |
−40.2 ± 2.0 |
−48.4 ± 2.4 |
1-Octanol |
10.2 ± 0.4 |
−25.3 ± 1.8 |
−46.6 ± 2.3 |
−71.5 ± 3.6 |
1-Nonanol |
10.4 ± 0.4 |
−25.7 ± 1.8 |
−45.7 ± 2.3 |
−67.2 ± 3.4 |
1-Decanol |
10.6 ± 0.4 |
−26.3 ± 1.9 |
−43.2 ± 2.2 |
−56.9 ± 2.8 |
2-Pentanol |
11.8 ± 0.5 |
−29.3 ± 2.1 |
−46.4 ± 2.3 |
−57.4 ± 2.9 |
2-Hexanol |
10.3 ± 0.4 |
−25.5 ± 1.8 |
−40.5 ± 2.0 |
−50.3 ± 2.5 |
2-Heptanol |
9.7 ± 0.4 |
−24.2 ± 1.7 |
−41.4 ± 2.1 |
−57.8 ± 2.9 |
2-Octanol |
9.6 ± 0.4 |
−23.8 ± 1.7 |
−42.3 ± 2.1 |
−62.3 ± 3.1 |
2-Nonanol |
9.9 ± 0.4 |
−24.6 ± 1.7 |
−36.4 ± 1.8 |
−39.5 ± 2.0 |
2-Decanol |
10.0 ± 0.4 |
−24.8 ± 1.8 |
−40.0 ± 2.0 |
−51.1 ± 2.6 |
2-Pentanone |
12.3 ± 0.5 |
−30.4 ± 2.1 |
−46.3 ± 2.3 |
−53.4 ± 2.7 |
2-Hexanone |
10.2 ± 0.4 |
−25.3 ± 1.8 |
−28.4 ± 1.4 |
−10.4 ± 0.5 |
2-Heptanone |
10.2 ± 0.4 |
−25.2 ± 1.8 |
−30.1 ± 1.5 |
−16.5 ± 0.8 |
2-Octanone |
10.6 ± 0.4 |
−26.2 ± 1.9 |
−23.1 ± 0.12 |
10.3 ± 0.5 |
2-Nonanone |
11.0 ± 0. |
−27.3 ± 1.9 |
−29.5 ± 1.5 |
−7.3 ± 0.4 |
2-Decanone |
11.4 ± 0.5 |
−28.2 ± 2.0 |
−32.9 ± 1.6 |
−15.6 ± 0.8 |
Hexane |
18.2 ± 0.7 |
−45.0 ± 3.2 |
−126.7 ± 6.3 |
−273.9 ± 13.7 |
Heptane |
19.5 ± 0.8 |
−48.4 ± 3.4 |
−124.4 ± 6.2 |
−255.0 ± 12.8 |
Octane |
19.2 ± 0.8 |
−47.7 ± 3.4 |
−125.1 ± 6.3 |
−260.0 ± 13.0 |
Nonane |
19.4 ± 0.8 |
−48.1 ± 3.4 |
−124.2 ± 6.2 |
−255.3 ± 12.8 |
Decane |
18.8 ± 0.8 |
−46.7 ± 3.3 |
−120.3 ± 6.0 |
−247.2 ± 12.4 |
Table 5 Standard molar Gibbs energy ΔG0298 K enthalpy ΔH0298 K, and entropy ΔS0298 K changes and natural logarithm of apparent extraction constants for the extraction of Am3+ in tested systems. In polar diluents, concentration of TODGA was 20 mmol L−1, whereas in alkanes 2 mmol L−1 concentration of TODGA was used
Diluent |
ln Kex298 K |
ΔG0298 K (kJ mol−1) |
ΔH0298 K (kJ mol−1) |
ΔS0298 K [J (mol K)−1] |
1-Pentanol |
7.8 ± 0.3 |
−19.3 ± 1.4 |
−31.6 ± 1.6 |
−41.3 ± 2.1 |
1-Hexanol |
6.9 ± 0.3 |
−17.1 ± 1.2 |
−30.5 ± 1.5 |
−45.1 ± 2.3 |
1-Heptanol |
6.8 ± 0.3 |
−16.8 ± 1.2 |
−29.4 ± 1.5 |
−42.3 ± 2.1 |
1-Octanol |
6.4 ± 0.3 |
−15.8 ± 1.1 |
−34.7 ± 1.7 |
−63.7 ± 3.2 |
1-Nonanol |
6.6 ± 0.3 |
−16.4 ± 1.2 |
−34.6 ± 1.7 |
−61.1 ± 3.1 |
1-Decanol |
6.7 ± 0.3 |
−16.6 ± 1.2 |
−34.5 ± 1.7 |
−60.0 ± 3.0 |
2-Pentanol |
8.3 ± 0.3 |
−20.5 ± 1.4 |
−35.1 ± 1.8 |
−49.2 ± 2.5 |
2-Hexanol |
6.7 ± 0.3 |
−16.7 ± 1.2 |
−27.2 ± 1.4 |
−35.3 ± 1.8 |
2-Heptanol |
6.3 ± 0.3 |
−15.5 ± 1.1 |
−28.8 ± 1.4 |
−44.6 ± 2.2 |
2-Octanol |
6.1 ± 0.2 |
−15.0 ± 1.1 |
−29.7 ± 1.5 |
−49.1 ± 2.5 |
2-Nonanol |
6.4 ± 0.3 |
−15.8 ± 1.1 |
−24.2 ± 1.2 |
−28.3 ± 1.4 |
2-Decanol |
6.6 ± 0.3 |
−16.2 ± 1.1 |
−22.3 ± 1.1 |
−20.4 ± 1.0 |
2-Pentanone |
8.6 ± 0.3 |
−21.2 ± 1.5 |
−35.8 ± 1.8 |
−48.7 ± 2.4 |
2-Hexanone |
6.5 ± 0.3 |
−16.1 ± 1.1 |
−16.7 ± 0.8 |
−1.9 ± 0.1 |
2-Heptanone |
6.3 ± 0.3 |
−15.7 ± 1.1 |
−18.8 ± 0.9 |
−10.5 ± 0.5 |
2-Octanone |
6.8 ± 0.3 |
−16.7 ± 1.2 |
−13.2 ± 0.7 |
12.0 ± 0.6 |
2-Nonanone |
7.2 ± 0.3 |
−17.7 ± 1.3 |
−18.1 ± 0.9 |
−1.2 ± 0.1 |
2-Decanone |
7.2 ± 0.3 |
−17.8 ± 1.3 |
−23.7 ± 1.2 |
−19.8 ± 1 |
Hexane |
15.5 ± 0.6 |
−38.5 ± 2.7 |
−121.1 ± 6.1 |
−277.4 ± 13.9 |
Heptane |
16.6 ± 0.7 |
−41.0 ± 2.9 |
−114.7 ± 5.7 |
−247.2 ± 12.4 |
Octane |
16.8 ± 0.7 |
−41.6 ± 2.9 |
−116.8 ± 5.8 |
−252.2 ± 12.6 |
Nonane |
16.8 ± 0.7 |
−41.7 ± 3.0 |
−118.5 ± 5.9 |
−257.5 ± 12.9 |
Decane |
15.7 ± 0.6 |
−39.0 ± 3.0 |
−112.4 ± 5.6 |
−246.4 ± 12.3 |
4. Conclusions
The performed studies indicate that diluent plays significant role in the solvent extraction of Eu3+ and Am3+ with TODGA as an extracting agent. Examinations of the effect of carbon chain length revealed that carbon chain length has significant impact on the extraction outcome in the polar diluents. Diluents with 5 carbon atoms in aliphatic chain showed higher distribution ratios as well as separation factors than longer chained homologues, leading to the concave shaped distribution ratios dependence on the carbon chain length. However, the extraction behavior in 5 carbon chain diluents could not be reasonably explained. It was shown that the diluents apart from alkanes extract a significant amount of nitric acid during the contacting with aqueous phase. This in conjunction with water absorption will lead to significant changes of the dielectric constant organic phase and the electrical forces affecting the solubility of the extracted species in the organic phase. The determined D-values and separation factors correlate with the amount of extracted HNO3 and water into the organic phase for diluents with carbon chain C6–C10. However, this correlation is not straightforward between the different diluent types as well as for C5 diluents which could mean that more than one phenomenon might take place.
Extraction process for both Eu3+ and Am3+ with TODGA is enthalpy driven in all studied diluent types. Performed studies suggest that in the alkanes extractions behave significantly more exothermic than in the other diluents. Moreover, in the case of alkanes, both distribution ratios as well as separation factors are significantly higher than in any other type of diluents.
The stoichiometry investigation indicates that at least three different metal complexes are extracted into the organic phase dependable on the used diluent. The average ligand number of TODGA molecules in the extracted complex was typically lower for Am3+ when compared with Eu3+ except for alkane diluents.
Author contributions
I. K.: conceptualization, resources, methodology, formal analysis, investigation, visualization, writing – original draft, writing – review & editing. M. F.: methodology, investigation, writing – review & editing. C. E.: formal analysis, writing – review & editing. A. V. M. methodology, formal analysis, investigation, writing – original draft, writing – review & editing.
Conflicts of interest
There are no conflicts to declare.
Acknowledgements
The authors are grateful to Dr Mikhail S. Tyumentsev and Dr Robert Eichler for valuable scientific discussion which helped to improve this work. The work was funded by Swissnuclear grant WIR-2 (LRC_20_02).
References
- Y. A. El-Nadi, Solvent Extraction and Its Applications on Ore Processing and Recovery of Metals: Classical Approach, Sep. Purif. Rev., 2017, 46(3), 195–215 CrossRef CAS.
- M. Petranikova, I. Herdzik-Koniecko, B.-M. Steenari and C. Ekberg, Hydrometallurgical processes for recovery of valuable and critical metals from spent car NiMH batteries optimized in a pilot plant scale, Hydrometallurgy, 2017, 171, 128–141 CrossRef CAS.
- J. C. M. M. C. C. G. R. Rydberg, Solvent Extraction Principles and Practice, Revised and Expanded, CRC Press, Boca Raton, 2004 Search PubMed.
- K. Binnemans, P. T. Jones, B. Blanpain, T. Van Gerven, Y. Yang, A. Walton and M. Buchert, Recycling of rare earths: a critical review, J. Cleaner Prod., 2013, 51, 1–22 CrossRef CAS.
- A. D. McNaught and AWUPAC, Compendium of Chemical Terminology: the Gold Book, ed. S. J. Chalk, Blackwell Scientific Publications, Oxford, 2nd edn, 1997 Search PubMed.
- N. B. Devi and B. Nayak, Liquid–liquid extraction and separation of copper(II) and nickel(II) using LIX®984N, J. South. Afr. Inst. Min. Metall., 2014, 114, 937–943 CAS.
- D. Dyrssen and L. D. Hay, Solvent extraction of 152,154Eu and 241Am by Di-n-butyl Phosphate (DBP) in Different Organic Solvents, Acta Chem. Scand., 1960, 14, 1100–1111 CrossRef CAS.
- T. V. Healy, Synergism in the solvent extraction of di-, tri- and tetravalent metal ions—II: Synergic effects in so-called inert diluents, J. Inorg. Nucl. Chem., 1961, 19(3), 328–339 CrossRef.
- R. K. Jyothi, H.-R. Kim, J. S. Kim, K.-W. Chung and J.-Y. Lee, Diluents Role in Extraction and Possible Separation of Light Rare Earth Elements from Chloride Solutions by using Cyanex®272 used as an Extractant, Korean J. Met. Mater., 2018, 56(10), 763–771 CrossRef CAS.
- A. S. Kertes and F. Grauer, Thermodynamics of solvent extraction processes: Effect of diluent on the enthalpy of formation of tri-n-octylammonium chloride, J. Inorg. Nucl. Chem., 1978, 40(10), 1781–1782 CrossRef CAS.
- E. Löfström-Engdahl, E. Aneheim, C. Ekberg, M. Foreman, J. Halleröd and G. Skarnemark, Extraction thermodynamics of Am(III) and Eu(III) using CyMe4-BTBP in various organic diluents, J. Chem. Thermodyn., 2014, 76, 64–69 CrossRef.
- E. Löfström-Engdahl, E. Aneheim, C. Ekberg and G. Skarnemark, A reinterpretation of C5-BTBP extraction data, performed in various alcohols, J. Radioanal. Nucl. Chem., 2013, 296(2), 733–737 CrossRef.
- K. Rezaei and H. Nedjate, Diluent effect on the distribution ratio and separation factor of Ni(II) in the liquid–liquid extraction from aqueous acidic solutions using dibutyldithiophosphoric acid, Hydrometallurgy, 2003, 68(1), 11–21 CrossRef CAS.
- G. M. Ritcey, Solvent Extraction in Hydrometallurgy: Present and Future, Tsinghua Sci. Technol., 2006, 11(2), 137–152 CAS.
- V. S. Shmidt and K. A. Rybakov, Predicting the effect of the nature of diluents on extraction equilibria, Soviet At. Energy, 1981, 50(6), 365–371 CrossRef.
- M. Taube, The influence of diluent polarity on the extraction of neptunium and uranium compounds to organic media, J. Inorg. Nucl. Chem., 1960, 15(1), 171–176 CrossRef CAS.
- J. H. Hildebrand, Solubility, American Chemical Society, New York, Monograph Series, 2nd edn, 1936 Search PubMed.
- J. H. Hildebrand, J. M. Prausnitz and R. L. Scott, Regular and related solutions: the solubility of gases, liquids, and solids, Van Nostrand Reinhold Co., 1970 Search PubMed.
- C. Hansen, Hansen Solubility Parameters, CRC Press, 2007 Search PubMed.
- T. Lécrivain, A. Kimberlin, D. E. Dodd, S. Miller, I. Hobbs, E. Campbell, F. Heller, J. Lapka, M. Huber and K. L. Nash, The Effect of Organic Diluent on the Extraction of Eu(III) by HEH[EHP], Solvent Extr. Ion Exch., 2019, 37(3–4), 284–296 CrossRef.
- M. Nilsson, S. Andersson, F. Drouet, C. Ekberg, M. Foreman, M. Hudson, J. O. Liljenzin, D. Magnusson and G. Skarnemark, Extraction Properties of 6,6′-Bis-(5,6-dipentyl-[1,2,4]triazin-3-yl)-[2,2′]bipyridinyl (C5-BTBP), Solvent Extr. Ion Exch., 2006, 24(3), 299–318 CrossRef CAS.
- A. Wilden, P. M. Kowalski, L. Klaß, B. Kraus, F. Kreft, G. Modolo, Y. Li, J. Rothe, K. Dardenne, A. Geist, A. Leoncini, J. Huskens and W. Verboom, Unprecedented Inversion of Selectivity and Extraordinary Difference in the Complexation of Trivalent f Elements by Diastereomers of a Methylated Diglycolamide, Chem.–Eur. J., 2019, 25(21), 5507–5513 CrossRef CAS PubMed.
- A. Wilden, G. Modolo, S. Lange, F. Sadowski, B. B. Beele, A. Skerencak-Frech, P. J. Panak, M. Iqbal, W. Verboom, A. Geist and D. Bosbach, Modified Diglycolamides for the An(III) + Ln(III) Co-separation: Evaluation by Solvent Extraction and Time-Resolved Laser Fluorescence Spectroscopy, Solvent Extr. Ion Exch., 2014, 32(2), 119–137 CrossRef CAS.
- P. Zsabka, K. Van Hecke, L. Adriaensen, A. Wilden, G. Modolo, M. Verwerft, K. Binnemans and T. Cardinaels, Solvent Extraction of Am(III), Cm(III), and Ln(III) Ions from Simulated Highly Active Raffinate Solutions by TODGA Diluted in Aliquat-336 Nitrate Ionic Liquid, Solvent Extr. Ion Exch., 2018, 36(6), 519–541 CrossRef CAS.
- G. Modolo, A. Wilden, A. Geist, D. Magnusson and R. Malmbeck, A review of the demonstration of innovative solvent extraction processes for the recovery of trivalent minor actinides from PUREX raffinate, Radiochim. Acta, 2012, 715 CrossRef CAS.
- V. Ostapenko, A. Vasiliev, E. Lapshina, S. Ermolaev, R. Aliev, Y. Totskiy, B. Zhuikov and S. Kalmykov, Extraction chromatographic behavior of actinium and REE on DGA, Ln and TRU resins in nitric acid solutions, J. Radioanal. Nucl. Chem., 2015, 306(3), 707–711 CrossRef CAS.
- V. Radchenko, T. Mastren, C. A. L. Meyer, A. S. Ivanov, V. S. Bryantsev, R. Copping, D. Denton, J. W. Engle, J. R. Griswold, K. Murphy, J. J. Wilson, A. Owens, L. Wyant, E. R. Birnbaum, J. Fitzsimmons, D. Medvedev, C. S. Cutler, L. F. Mausner, M. F. Nortier, K. D. John, S. Mirzadeh and M. E. Fassbender, Radiometric evaluation of diglycolamide resins for the chromatographic separation of actinium from fission product lanthanides, Talanta, 2017, 175, 318–324 CrossRef CAS PubMed.
- A. K. H. Robertson, B. L. McNeil, H. Yang, D. Gendron, R. Perron, V. Radchenko, S. Zeisler, P. Causey and P. Schaffer, 232Th-Spallation-Produced 225Ac with Reduced 227Ac Content, Inorg. Chem., 2020, 59(17), 12156–12165 CrossRef CAS PubMed.
- B. Zielinska, C. Apostolidis, F. Bruchertseifer and A. Morgenstern, An Improved Method for the Production of Ac-225/Bi-213 from Th-229 for Targeted Alpha Therapy, Solvent Extr. Ion Exch., 2007, 25(3), 339–349 CrossRef CAS.
- Y. Sasaki, Y. Sugo, S. Suzuki and S. Tachimori, The novel extractants, diglycolamides, for the extraction of lanthanides and actinides in HNO3–n-dodecane system, Solvent Extr. Ion Exch., 2001, 19(1), 91–103 CrossRef CAS.
- Z.-X. Zhu, Y. Sasaki, H. Suzuki, S. Suzuki and T. Kimura, Cumulative study on solvent extraction of elements by N,N,N′,N′-tetraoctyl-3-oxapentanediamide (TODGA) from nitric acid into n-dodecane, Anal. Chim. Acta, 2004, 527(2), 163–168 CrossRef CAS.
- S. A. Ansari, P. Pathak, P. K. Mohapatra and V. K. Manchanda, Chemistry of Diglycolamides: Promising Extractants for Actinide Partitioning, Chem. Rev., 2012, 112(3), 1751–1772 CrossRef CAS PubMed.
- K. Bell, A. Geist, F. McLachlan, G. Modolo, R. Taylor and A. Wilden, Nitric Acid Extraction into TODGA, Procedia Chem., 2012, 7, 152–159 CrossRef CAS.
- G. Modolo, H. Asp, H. Vijgen, R. Malmbeck, D. Magnusson and C. Sorel, Demonstration of a TODGA-Based Continuous Counter-Current Extraction Process for the Partitioning of Actinides from a Simulated PUREX Raffinate, Part II: Centrifugal Contactor Runs, Solvent Extr. Ion Exch., 2008, 26(1), 62–76 CrossRef CAS.
- A. Bhattacharyya, R. J. M. Egberink, P. K. Mohapatra, P. K. Verma, A. S. Kanekar, A. K. Yadav, S. N. Jha, D. Bhatacharyya, J. Huskens and W. Verboom, Remarkable Enhancement in Extraction of Trivalent f-Block Elements Using a Macrocyclic Ligand with Four Diglycolamide Arms: Synthesis, Extraction, and Spectroscopic and Density Functional Theory Studies, Inorg. Chem., 2019, 58(21), 14885–14899 CrossRef CAS PubMed.
- Y. Pan and C. L. Hussey, Electrochemical and Spectroscopic Investigation of Ln3+ (Ln = Sm, Eu, and Yb) Solvation in Bis(trifluoromethylsulfonyl)imide-Based Ionic Liquids and Coordination by N,N,N′,N′-Tetraoctyl-3-oxa-pentane Diamide (TODGA) and Chloride, Inorg. Chem., 2013, 52(6), 3241–3252 CrossRef CAS PubMed.
- A. N. Turanov, V. K. Karandashev, M. Boltoeva, C. Gaillard and V. Mazan, Synergistic extraction of uranium(VI) with TODGA and hydrophobic ionic liquid mixtures into molecular diluent, Sep. Purif. Technol., 2016, 164, 97–106 CrossRef CAS.
- P. Weßling, U. Müllich, E. Guerinoni, A. Geist and P. J. Panak, Solvent extraction of An(III) and Ln(III) using TODGA in aromatic diluents to suppress third phase formation, Hydrometallurgy, 2020, 192, 105248 CrossRef.
- E. L. Campbell, V. E. Holfeltz, G. B. Hall, K. L. Nash, G. J. Lumetta and T. G. Levitskaia, Nitric Acid and Water Extraction by T2EHDGA in n-Dodecane, Solvent Extr. Ion Exch., 2017, 35(7), 586–603 CrossRef CAS.
- D. Woodhead, F. McLachlan, R. Taylor, U. Müllich, A. Geist, A. Wilden and G. Modolo, Nitric Acid Extraction into a TODGA Solvent Modified with 1-Octanol, Solvent Extr. Ion Exch., 2019, 37(2), 173–190 CrossRef CAS.
- F. McLachlan, K. Greenough, A. Geist, B. McLuckie, G. Modolo, A. Wilden and R. Taylor, Nitric Acid Extraction into the TODGA/TBP Solvent, Solvent Extr. Ion Exch., 2016, 34(4), 334–346 CrossRef CAS.
- M. Sypula, A. Ouadi, C. Gaillard and I. Billard, Kinetics of metal extraction in ionic liquids: Eu3+/HNO3//TODGA/[C1C4im][Tf2N] as a case study, RSC Adv., 2013, 3(27), 10736–10744 RSC.
- Y. Sasaki, P. Rapold, M. Arisaka, M. Hirata, T. Kimura, C. Hill and G. Cote, An Additional Insight into the Correlation between the Distribution Ratios and the Aqueous Acidity of the TODGA System, Solvent Extr. Ion Exch., 2007, 25(2), 187–204 CrossRef CAS.
- S. A. Ansari, P. N. Pathak, M. Husain, A. K. Prasad, V. S. Parmar and V. K. Manchanda, Extraction of actinides using N,N,N′,N′-tetraoctyl diglycolamide (TODGA): a thermodynamic study, Radiochim. Acta, 2006, 307 CrossRef CAS.
- P. N. Pathak, S. A. Ansari, S. V. Godbole, A. R. Dhobale and V. K. Manchanda, Interaction of Eu3+ with N,N,N′,N′-tetraoctyl diglycolamide: A time resolved luminescence spectroscopy study, Spectrochim. Acta, Part A, 2009, 73(2), 348–352 CrossRef CAS.
- M. Boltoeva, C. Gaillard, S. Georg, V. K. Karandashev and A. N. Turanov, Speciation of uranium(VI) extracted from acidic nitrate media by TODGA into molecular and ionic solvents, Sep. Purif. Technol., 2018, 203, 11–19 CrossRef CAS.
- S. Panja, P. K. Mohapatra, S. C. Tripathi, P. M. Gandhi and P. Janardan, A highly efficient solvent system containing TODGA in room temperature ionic liquids for actinide extraction, Sep. Purif. Technol., 2012, 96, 289–295 CrossRef CAS.
- Y. Sasaki, Y. Sugo, K. Morita and K. L. Nash, The Effect of Alkyl Substituents on Actinide and Lanthanide Extraction by Diglycolamide Compounds, Solvent Extr. Ion Exch., 2015, 33(7), 625–641 CrossRef CAS.
- S. A. Ansari, P. N. Pathak, V. K. Manchanda, M. Husain, A. K. Prasad and V. S. Parmar, N,N,N′,N′-Tetraoctyl Diglycolamide (TODGA): A Promising Extractant for Actinide-Partitioning from High-Level Waste (HLW), Solvent Extr. Ion Exch., 2005, 23(4), 463–479 CrossRef CAS.
- M. Sypula, Innovative SANEX process for trivalent actinides separation from PUREX raffinate, in Schriften des Forschungszentrums J\\'fclich Reihe Energie & Umwelt/Energy & Environment\\, RWTH Aachen\\, J\\'fclich\\, 2014, p. 220 Search PubMed.
- T. Vidmar, EFFTRAN—A Monte Carlo efficiency transfer code for gamma-ray spectrometry, Nucl. Instrum. Methods Phys. Res., Sect. A, 2005, 550(3), 603–608 CrossRef CAS.
- Y. Xu, Y. Gao, Y. Zhou, C. Fan, H. Hou and M. Zhang, Extraction behavior of strontium from nitric acid medium with N,N′-dimethyl-N,N′-dioctyldiglycolamide, Solvent Extr. Ion Exch., 2017, 35(7), 507–518 CrossRef CAS.
- A. Geist, Extraction of Nitric Acid into Alcohol: Kerosene Mixtures, Solvent Extr. Ion Exch., 2010, 28(5), 596–607 CrossRef CAS.
- Chemical thermodynamics of americium. Chemical thermodynamics, ed. R. J. Silva, Amsterdam, Elsevier, 1995, vol. 2 Search PubMed.
- P. L. Brown and C. Ekberg, Hydrolysis of Metal Ions, Wiley, 2016, p. 952 Search PubMed.
- S. Hlushak, J. P. Simonin, S. De Sio, O. Bernard, A. Ruas, P. Pochon, S. Jan and P. Moisy, Speciation in aqueous solutions of nitric acid, Dalton Trans., 2013, 42(8), 2853–2860 RSC.
- N. H. C. Lewis, J. A. Fournier, W. B. Carpenter and A. Tokmakoff, Direct Observation of Ion Pairing in Aqueous Nitric Acid Using 2D Infrared Spectroscopy, J. Phys. Chem. B, 2019, 123(1), 225–238 CrossRef CAS PubMed.
- J. M. Anglada, M. T. C. Martins-Costa, J. S. Francisco and M. F. Ruiz-López, Reactivity of Undissociated Molecular Nitric Acid at the Air–Water Interface, J. Am. Chem. Soc., 2021, 143(1), 453–462 CrossRef CAS PubMed.
- T. Lewis, B. Winter, A. C. Stern, M. D. Baer, C. J. Mundy, D. J. Tobias and J. C. Hemminger, Does Nitric Acid Dissociate at the Aqueous Solution Surface?, J. Phys. Chem. C, 2011, 115(43), 21183–21190 CrossRef CAS.
- T. Langner, A. Rietig and J. Acker, Raman spectroscopic determination of the degree of dissociation of nitric acid in binary and ternary mixtures with HF and H2SiF6, J. Raman Spectrosc., 2020, 51(2), 366–372 CrossRef CAS.
- S. Wang, R. Bianco and J. T. Hynes, Dissociation of nitric acid at an aqueous surface: Large amplitude motions in the contact ion pair to solvent-separated ion pair conversion, Phys. Chem. Chem. Phys., 2010, 12(29), 8241–8249 RSC.
- A. V. Levanov, O. Y. Isaikina and V. V. Lunin, Dissociation constant of nitric acid, Russ. J. Phys. Chem. A, 2017, 91(7), 1221–1228 CrossRef CAS.
- Y. Marcus and G. Hefter, Ion Pairing, Chem. Rev., 2006, 106(11), 4585–4621 CrossRef CAS PubMed.
- A. V. Matyskin, P. L. Brown and C. Ekberg, Weak barium and radium hydrolysis using an ion exchange method and its uncertainty assessment, J. Chem. Thermodyn., 2019, 128, 362–371 CrossRef CAS.
- L. Ciavatta, The specific interaction theory in equilibrium analysis: some empirical rules for estimating interaction coefficients of metal-ion complexes, Ann. Chim., 1990, 80, 255–263 CAS.
- R. J. B. G. Silva, M. H. Rand, P. B. Robouch, H. Wanner and I. Puigdomenech, Chemical Thermodynamics of Americium, Nuclear Energy Agency, 1995 Search PubMed.
- M. Goral and B. Wisniewska-Goclowska, IUPAC-NIST Solubility Data Series. 82. Alcohols with Water—Revised and Updated: Part 3. C6 Alcohols with Water, J. Phys. Chem. Ref. Data, 2007, 36(2), 399–443 CrossRef.
- A. Maczynski, D. G. Shaw, M. Goral, B. Wisniewska-Goclowska, A. Skrzecz, Z. Maczynska, I. Owczarek, K. Blazej, M.-C. Haulait-Pirson, F. Kapuku, G. T. Hefter and A. Szafranski, IUPAC-NIST Solubility Data Series. 81. Hydrocarbons with Water and Seawater–Revised and Updated Part 1. C5 Hydrocarbons with Water, J. Phys. Chem. Ref. Data, 2005, 34(2), 441–476 CrossRef CAS.
- M. Goral and B. Wisniewska-Goclowska, IUPAC-NIST Solubility Data Series. 82: Alcohols with Water—Revised and Updated: Part 2. C5 Alcohols with Water, J. Phys. Chem. Ref. Data, 2007, 36(1), 133–190 CrossRef.
- M. Goral and B. Wisniewska-Goclowska, IUPAC-NIST Solubility Data Series. 82. Alcohols with Water—Revised and Updated: Part 4. C7 Alcohols with Water, J. Phys. Chem. Ref. Data, 2007, 36(2), 445–484 CrossRef.
- M. Goral and B. Wisniewska-Goclowska, IUPAC-NIST Solubility Data Series. 82. Alcohols with Water–Revised and Updated: Part 5. C8–C17 Alcohols with Water, J. Phys. Chem. Ref. Data, 2007, 36(3), 685–731 CrossRef.
- M. Góral and B. Wiśniewska-Gocłowska, IUPAC-NIST Solubility Data Series. 86. Ethers and Ketones with Water. Part 4. C4 and C5 Ketones with Water, J. Phys. Chem. Ref. Data, 2008, 37(3), 1517–1574 CrossRef.
- M. Góral and B. Wiśniewska-Gocłowska, IUPAC-NIST Solubility Data Series. 86. Ethers and Ketones with Water. Part 5. C6 Ketones with Water, J. Phys. Chem. Ref. Data, 2008, 37(3), 1575–1609 CrossRef.
- M. Góral and B. Wiśniewska-Gocłowska, IUPAC-NIST Solubility Data Series. 86. Ethers and Ketones with Water. Part 6. C7–C12 Ketones with Water, J. Phys. Chem. Ref. Data, 2008, 37(3), 1611–1653 CrossRef.
- M. Arisaka and T. Kimura, Thermodynamic and Spectroscopic Studies on Am(III) and Eu(III) in the Extraction System of N,N,N′,N′-Tetraoctyl-3-Oxapentane-1,5-Diamide in n-Dodecane/Nitric Acid, Solvent Extr. Ion Exch., 2011, 29(1), 72–85 CrossRef CAS.
- E. A. Mowafy and H. F. Aly, Synthesis of some N,N,N′,N′-Tetraalkyl-3-Oxa-Pentane-1,5-Diamide and their Applications in Solvent Extraction, Solvent Extr. Ion Exch., 2007, 25(2), 205–224 CrossRef CAS.
- P. N. Pathak, S. A. Ansari, S. Kumar, B. S. Tomar and V. K. Manchanda, Dynamic light scattering study on the aggregation behaviour of N,N,N′,N′-tetraoctyl diglycolamide (TODGA) and its correlation with the extraction behaviour of metal ions, J. Colloid Interface Sci., 2010, 342(1), 114–118 CrossRef CAS PubMed.
- I. Herdzik-Koniecko, C. Wagner, M. Trumm, U. Müllich, B. Schimmelpfennig, J. Narbutt, A. Geist and P. J. Panak, Do An(III) and Ln(III) ions form heteroleptic complexes with diglycolamide and hydrophilic BT(B)P ligands in solvent extraction systems? A spectroscopic and DFT study, New J. Chem., 2019, 43(16), 6314–6322 RSC.
- H. Suzuki, Y. Sasaki, Y. Sugo, A. Apichaibukol and T. Kimura, Extraction and separation of Am(III) and Sr(II) by N,N,N′,N′-tetraoctyl-3-oxapentanediamide (TODGA), Radiochim. Acta, 2004, 92(8), 463–466 CrossRef CAS.
- M. R. Antonio, D. R. McAlister and E. P. Horwitz, An europium(III) diglycolamide complex: insights into the coordination chemistry of lanthanides in solvent extraction, Dalton Trans., 2015, 44(2), 515–521 RSC.
- H. Narita, T. Yaita and S. Tachimori, Extraction behavior for trivalent lanthanides with amides and EXAFS study of their complexes, Sol. Extr. Ind. Appl., ISEC, 2000, vol. 99(1), p. 693 Search PubMed.
- T. Yaita, A. W. Herlinger, P. Thiyagarajan and M. P. Jensen, Influence of Extractant Aggregation on the Extraction of Trivalent f-Element Cations by a Tetraalkyldiglycolamide, Solvent Extr. Ion Exch., 2004, 22(4), 553–571 CrossRef CAS.
- S. A. Ansari, P. N. Pathak, M. Husain, A. K. Prasad, V. S. Parmar and V. K. Manchanda, Extraction of actinides using N,N,N′,N′-tetraoctyl diglycolamide (TODGA): a thermodynamic study, Radiochim. Acta, 2006, 94(6–7), 307–312 CrossRef CAS.
- Z. Chen, X. Yang, L. Song, X. Wang, Q. Xiao, H. Xu, Q. Feng and S. Ding, Extraction and complexation of trivalent rare earth elements with tetraalkyl diglycolamides, Inorg. Chim. Acta, 2020, 513, 119928 CrossRef CAS.
Footnote |
† Present address: Chemistry Division, Los Alamos National Laboratory, P. O. Box 1663, 87545 Los Alamos, New Mexico, United States of America. |
|
This journal is © The Royal Society of Chemistry 2021 |