DOI:
10.1039/D1RA07165C
(Paper)
RSC Adv., 2021,
11, 38648-38653
[3+2] regioselective annulation reaction of 2-arylidene-1,3-indandiones towards synthesis of spirocyclopentenes: understanding the mechanism of γ-attack vs. α-attack using DFT studies†
Received
25th September 2021
, Accepted 19th November 2021
First published on 1st December 2021
Abstract
A regioselective [3+2] cyclisation reaction between 2-arylidene-1,3-indanedione and ethyl 2,3-butadienoate catalysed by triphenylphosphine has been demonstrated to synthesize functionalised spirocyclic cyclopentenes. The reaction tolerated various electron-rich and electron-deficient aryl substituted 2-arylidene-1,3-indanediones with high to excellent chemical yields (up to 99%) and moderate to good regioselectivity (up to 5
:
1). DFT studies have also been carried out to understand the regioselective nature of this reaction. The results of Frontier molecular orbital calculations and the activation energy (Ea) favour the formation of compound 3a via γ-attack compared to that of 4a via α-attack.
Introduction
Nucleophilic phosphine-catalysed annulation of allenes has emerged as a powerful tool for the construction of diverse carbocycles and heterocycles.1 Various electrophiles such as activated alkenes,2 enones,3 ketones,4 imines,5 oxo-dienes,6 cyclic ketimines,7 chromenones,8 azomethine imines,9 salicyl N-thiophosphinyl imines,10 alkylidene malononitriles,11 exocyclic enones,12 aldehydes,13 N-acyldiazenes,14 naphthaquinones,15 p-quinine methide,16 amino esters,17 and diones18 have been used for the annulation reaction. The first [3+2] asymmetric process was reported by Zhang et al.19 Later on several chiral annulation reactions were investigated.20
1,3-Indandione is an important constituent of numerous natural products and bioactive skeletons (Fig. 1)21 and has been used as a reaction substrate extensively.22 Some of these natural products display activity due to spirocyclic moieties23 of various sizes. In recent years, a readily accessible material i.e. 2-arylidene-1,3-indandiones24 has started gaining importance in the field of phosphine catalysed annulations.25 A regioselective [4+2]26 cyclisation between 2-arylidene-1,3-indandiones and γ-substituted allenoates was carried out explicitly by Huang and co-workers towards synthesis of spiro[4.5]dec-6-ene skeletons as δ-adduct (eqn (a), Scheme 1).27 Very recently, Zheng and Er-Qing carried out a phosphine catalysed [3+2] cyclisation with γ-methyl allenoates for the synthesis of spirocyclopentene as α-adduct (eqn (b), Scheme 1).28 In continuation with our interest towards the synthesis of spiro carbocycles,29 we herein report a regioselective [3+2] cyclisation of 2-arylidene-1,3-indandiones and 2,3-butadienoate catalysed by triphenylphosphine (eqn (c), Scheme 1). To the best of our knowledge, this is the first report for the synthesis of functionalised spirocyclopentenes as γ-addition as well as α-addition adduct.
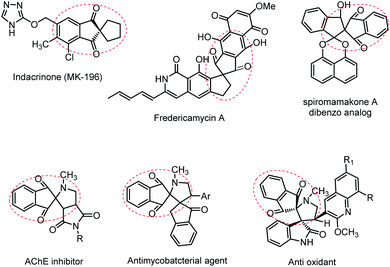 |
| Fig. 1 Natural and bioactive molecules containing Indanedione based spirocarbocycles and spiroheterocycles. | |
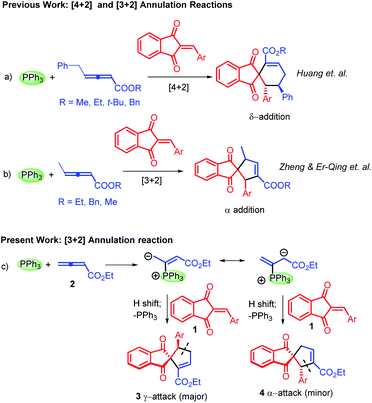 |
| Scheme 1 PPh3 driven annulation reaction of 2-arylidene-1,3-indanedione using allenoates. | |
Interestingly, spirocyclopentene 3 was obtained predominantly via γ-attack along with 4 as a minor product through α-attack of ethyl-2,3-butadienoate 2 on benzylidene-1,3-indanedione 1 using PPh3 (eqn (c), Scheme 1). The structures of both the products were further confirmed by single X-ray crystallography (Fig. 2).
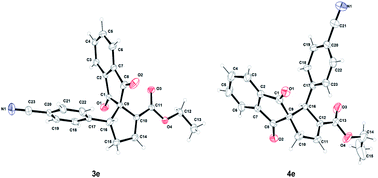 |
| Fig. 2 Crystal structures of 3e (CCDC 921839) and 4e (CCDC 921702). | |
Results and discussion
We initially carried out the reaction of 2-(benzylidene)-1,3-indanedione 1a with allenoate 2 using Ph3P (Table 1). The reaction was carried out in CH3CN at ambient temperature to give separable products 3a and 4a with a total chemical yield of 22% (Table 1, entry 1). The isolated yield of the reaction was improved to 41% when THF was used (Table 1, entry 2). A relatively electron-rich catalyst such as Bu3P showed the same reactivity with a marginal increase in yield using toluene as solvent (Table 1, entry 3). Improvement in the yield of the reaction was observed using Ph3P in toluene that resulted in good regioselectivity of 5
:
1 (Table 1, entries 4 and 5). Drastic improvement in the chemical yield of the reaction was observed when the reaction was performed under dilute conditions (Table 1, entries 6 and 7). A culminating point was observed in the yield of the reaction on a further increase in the volume of the reaction (Table 1, entry 8).
Table 1 Optimization of reaction conditionsa
With the optimised reaction conditions realised, we carried out the generalisation of the present protocol using various substituted 2-arylidene-1,3-indanediones 1b–n. It was observed that both electron-donating and electron-withdrawing groups were tolerable in the para-position of the phenyl ring in 1 (Table 2). The use of electron-withdrawing groups did not alter the reactivity of the reaction and the regioselectivity with quantitative yields was maintained (Table 2, entries 1 and 2). The chemical structures of γ- and α-substituted products were initially assigned by 1H, 13C-NMR, and HRMS analysis and were unambiguously assigned by single crystal X-ray diffraction for products 3e and 4e (Fig. 2).30
Table 2 Substrate Scope towards cascade formal [3+2] annulationa
The methyl ester group on the phenyl ring decreased the regioselectivity of the reaction presumably due to a decrease in electrophilicity of Michael acceptor 1c (Table 2, entry 3). The use of the –CN group demonstrated better regioselectivity and reactivity when compared to that of the –CF3 group (Table 2, entries 4–5). The presence of halogen-substituents in para-position of the phenyl ring was well tolerated with moderate regioselectivity and high chemical yields (Table 2, entries 6–8). The use of an acetoxy group was also compatible under the present reaction conditions (Table 2, entry 9). The use of electron-donating groups such as methyl, methoxy, and N,N-dimethyl on phenyl ring decreased the yield and regioselectivity for the formation of products 3j/4j to 3l/4l presumably due to the creation of electron density at the electrophilic carbon atom (Table 2, entries 10–12). Excellent chemical yields albeit with moderate regioselectivity when heteroaromatic substrates were used (Table 2, entries 13 and 14).
DFT studies
Computational methods
The computations were carried out using the Gaussian 09 suite of program.31 All the optimisations, transition states and single point energy calculations have been carried out at B3LYP/6-31g(d)32 level in the gas phase. The optimised conformation of reactants, intermediates and products were further confirmed that there is no imaginary frequency. Moreover, all the transition states were confirmed with a minimum of one imaginary frequency and the values are used in the discussion below.
Results and discussion. To corroborate the formation of major product spiro cyclopentene 3a among two possible regioisomers 3a and 4a, Density Functional Theory (DFT) calculations were exclusively studied. The key step involved in this reaction is the formation of highly reactive zwitterions A and B (see ESI †). Zwitterion A and B were produced by the attack of PPh3 on 2,3-butadienoate 2. 2-Benzylidene-1,3-indanedione 1a, zwitterions A and B were chosen as precursors for DFT calculations. Moreover, the HOMO–LUMO gap (see Fig. 3) for the zwitterions A and B was observed to be 3.17 and 2.74 eV, respectively, which confirms the lower band gap for the zwitterion B and its high reactivity (Fig. 3). The HOMO–LUMO gap has been calculated based on the higher oscillator strength observed for both zwitterions.
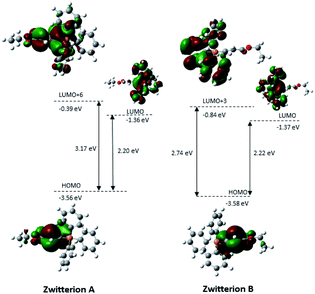 |
| Fig. 3 Frontier molecular orbital diagram of A and B obtained at B3LYP/6-31(d) level in gas phase. | |
The transition states were computed for the two possible products and the results were explained in Fig. 4. As shown in Fig. 4 (potential energy surface), initially, 1a was allowed to interact with A and has formed 1a–A complex with the binding energy of −6.35 kcal mol−1. Similarly, 1a was allowed to interact with B resulting in 1a–B complex with the binding energy of −7.04 kcal mol−1. The binding energy difference between the two complexes was observed to be minimal i.e., 0.69 kcal mol−1. The results show the competitive nature of zwitterions towards the formation of products 3a and 4a. The attack of methylene anion of B on α-carbon of 2-benzylidene-1,3-indandione 1a has produced TS1a with the vibrational frequency of −284.23 cm−1 and the barrier energy of 9.95 kcal mol−1. Whereas, the attack of methine anion of A on α-carbon of 2-benzylidene-1,3-indandione 1a has produced TS1 with the vibrational frequency of −196.10 cm−1 and the barrier energy of 6.72 kcal mol−1. The barrier energy difference between TS1 and TS1a was observed to be 2.54 kcal mol−1. This Michael addition leads to Int 1 and Int 1a with enthalpies of formation (ΔHf) −9.39 kcal mol−1 and −16.49 kcal mol−1, respectively. It was observed that Int 1a is more stable than that of Int 1 with an energy difference of 4.56 kcal mol−1. The intermediates, Int 1a and Int 1, produced TS2a and TS2 with the barrier energies of 8.81 and 7.69 kcal mol−1. The difference in barrier energies for both TS2 and TS2a was observed to be 1.12 kcal mol−1. Intramolecular Michael addition of Int 1a and Int 1 resulted in Int 2a and Int 2 respectively. The enthalpy of formation (ΔHf) of Int 2a and Int 2 was observed to be 10.21 and 10.77 kcal mol−1, respectively. Int 2a and Int 2 undergoes 1,2-proton transfer resulting in TS3a and TS3 having the barrier energies 47.11 kcal mol−1 and 73.31 kcal mol−1, respectively. It is clearly evident that TS3a is more stable than TS3 with a difference of 29.08 kcal mol−1.
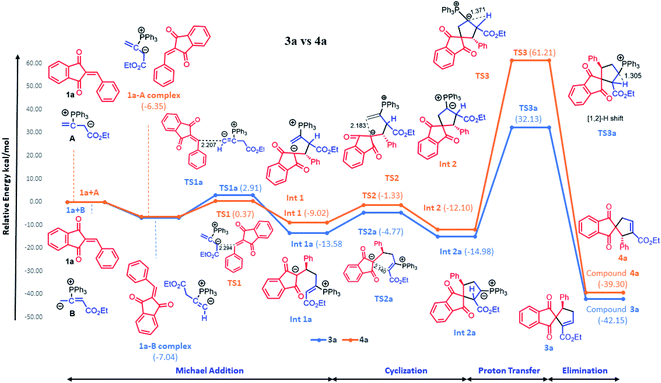 |
| Fig. 4 Energy barrier diagram for the formation of regioisomers 3a and 4a. | |
The less barrier energy observed for TS3a leads to the formation of 3a in line with the experimental observation of higher yield than that of 4a with the elimination of PPh3. For products 3a and 4a, the enthalpy energy was observed to be 74.28 and 100.51 kcal mol−1, respectively. It can be concluded that product formation 3a shows low barrier energy (Blue line) in all the steps except TS1a. Moreover, the computational results explained the cause of the major and minor products for this reaction.
No other product was detected from the 1H NMR analysis of the crude products, which demonstrated the efficiency of the current [3+2] cyclisation. With the standard protocol established for the synthesis of spirocyclopentenes, we next focused our attention on asymmetric variants by using various axially chiral trivalent phosphorus catalysts 5–7.
The use of chiral (S)-BINAP 5 gave a moderate yield of 68% with low regioselectivity (i.e., 2
:
1) and enantioselectivity for 3a/4a (i.e.15 and 10% ee, respectively). The use of chiral (R)-T-BINAP 6 resulted in poor chemical yield for 3g/4g even after 10 d of the reaction period. Finally, (R)-DM-BINAP 7 showed promising results with 74% yield for the formation of 3g/4g with high enantioselectivity of 81% for α-attack product 4g (Scheme 2).
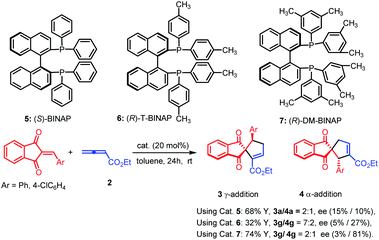 |
| Scheme 2 Asymmetric annulation catalysed by chiral catalysts. | |
A reasonable mechanism can be given for this regioselective [3+2] annulation reaction as shown in Scheme 3, catalyst Ph3P attacks the central sp carbon of ethyl-2,3-butadienoate 2 to generate Ph3P-allenoate zwitterionic intermediates A and B. The dipole A with the anion at the α-carbon position is believed to be more stable than dipole B with anion residing on γ-carbon position. This can be evident from the regioselectivity of products 3 and 4. These dipole intermediates then react with the Michael acceptor 1 in a [3+2] cyclisation fashion to form C and D. Subsequent ring closure, 1,2-proton transfer and further elimination of PPh3 gives the cyclopentenes 3 and 4 as major and minor products (Scheme 3).
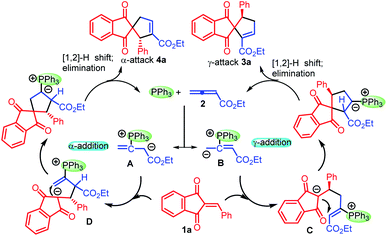 |
| Scheme 3 Possible catalytic cycle for products 3a and 4a. | |
Conclusions
In conclusion, we have demonstrated a regioselective [3+2] annulation reaction for the formation of spirocyclic cyclopentenes with high to excellent levels of chemical yield and regioselectivity. Previous work carried out using γ-substituted allenoates with 2-arylidene-1,3-indandiones in the presence of triphenylphosphine resulted in spiro[4.5]decene (i.e. δ-addition)27 and spiro[4.4]non-7-ene skeletons (i.e. α-addition).28 On the other hand, the current work also involves the use of 2-arylidene-1,3-indandiones which on reaction with simple allenoate such as ethyl-2,3-butadienoate in the presence of triphenylphosphine gives γ-addition spiro[4.4]non-6-ene adduct as the major product and α-addition spiro[4.4]non-7-ene adduct as the minor product. This triphenylphosphine mediated catalysis towards the synthesis of functionalized spirocarbocyclic derivatives results in the formation of asymmetric carbon centre as well as quaternary stereocentre. The result of DFT studies of frontier molecular orbital calculation on the regioselective reaction of 2-arylidene-1,3-indanedione and ethyl 2,3-butadienoate catalysed by triphenylphosphine shows a lower HOMO–LUMO gap for zwitterion B compared to that of A. Moreover, the activation energy has also been observed to be lower for 3a compared with that of 4a which shows the reactivity of zwitterion B which favours the formation of compound 3a as the major product.
Conflicts of interest
There are no conflicts to declare.
Acknowledgements
SA thanks DST-SERB under the young scientist scheme (SB/FT/CS-079/2014) for financial support. We thank the Ministry of Science and Technology of the Republic of China (MOST 108-2113-M-003-012) for financial support of this work. VS acknowledges Dr Shyi-Long Lee for his valuable support and provide the computational facilities to carry out this work. We thank Dr Kou and Dr R. K. Kottalanka for providing Fo/Fc data and ORTEP diagrams.
Notes and references
- For original publications, see:
(a) C. Zhang and X. Lu, J. Org.Chem., 1995, 60, 2906 CrossRef CAS;
(b) Y. Fujiwara and G. C. Fu, J. Am. Chem. Soc., 2011, 133, 12293 CrossRef CAS PubMed. For review articles, see:
(c) X. Lu, C. Zhang and Z. Xu, Acc. Chem. Res., 2001, 34, 535 CrossRef CAS PubMed;
(d) B. J. Cowen and S. C. Miller, Chem. Soc. Rev., 2009, 38, 3102 RSC;
(e) L.-W. Ye, J. Zhou and Y. Tang, Chem. Soc. Rev., 2008, 37, 1140 RSC;
(f) Y. Wei and M. Shi, Chin. J. Chem., 2020, 38, 1395 CrossRef CAS;
(g) Y. Zhu and Y. Huang, Synthesis, 2020, 52, 1181 CrossRef CAS;
(h) Q.-Y. Zhao, Z. Lian, Y. Wei and M. Shi, Chem. Commun., 2012, 48, 1724 RSC; For selected examples, see:
(i) X. Tang, C. X. A. Tan, W. –L. Chan, F. Zhang, W. Zheng and Y. Lu, ACS Catal., 2021, 11, 1361 CrossRef CAS;
(j) S. Debanath, A. S. Kumar, S. Chauhan and K. C. K. Swamy, J. Org. Chem., 2021, 86, 11583 CrossRef;
(k) T. Xu, D. Wang, W. Liu and X. Tong, Org. Lett., 2019, 21, 1944 CrossRef CAS;
(l) C. Cheng, X. Sun, Z. Wu, Q. Liu, L. Xiong and Z. Miao, Org. Biomol. Chem., 2019, 17, 3232 RSC;
(m) Z. Wang, Y. Fei, C. Tang, L. Cui, J. Shen, K. Yin, S. Lu and J. Li, Org. Lett., 2021, 23, 4094 CrossRef CAS PubMed.
-
(a) J.-H. Wu, L. Pan, J. Du, X. Wang, X. Wang, C. Jiang and T. Wang, Org. Lett., 2020, 22, 395 CrossRef CAS PubMed;
(b) E. Li, H. Jin and Y. Huang, ChemistrySelect, 2018, 3, 12007 CrossRef CAS;
(c) Q.-Z. Xi, Z. –J. Gan, E.-Q. Li and Z. Duan, Eur. J. Org. Chem., 2018, 2018, 4917 CrossRef CAS.
-
(a) J. E. Wilson and G. C. Fu, Angew. Chem., Int. Ed., 2006, 45, 1426 CrossRef CAS PubMed;
(b) A. Voituriez, A. Panossian, N. F. Bregeot, P. Retailleau and A. Marinetti, Adv. Synth. Catal., 2009, 351, 1968 CrossRef CAS;
(c) N. Pinto, P. Retailleau, A. Voituriez and A. Marinetti, Chem. Commun., 2011, 47, 1015 RSC.
-
(a) Q.-Y. Zhao, L. Huang, Y. Wei and M. Shi, Adv. Synth. Catal., 2012, 354, 1926 CrossRef CAS;
(b) T. Wang and S. Ye, Org. Lett., 2010, 12, 4168 CrossRef CAS PubMed.
-
(a) J. Tian and Z. He, Chem. Commun., 2013, 49, 2058 RSC;
(b) R. P. Wurz and G. C. Fu, J. Am. Chem. Soc., 2005, 127, 12234 CrossRef CAS PubMed;
(c) X. F. Zhu, J. Lan and O. Kwon, J. Am. Chem. Soc., 2003, 125, 4716 CrossRef CAS PubMed;
(d) Z. Yu, Z. Jin, M. Duan, R. Bai, Y. Lu and Y. Lan, J. Org. Chem., 2018, 83, 9729 CrossRef CAS;
(e) X. Kong, L. Liu, S. Luo, S. Fan, H. Qlan and H. Xiao, Synlett, 2018, 29, 1244 CrossRef CAS.
- X. Wang, T. Fang and X. Tong, Angew. Chem., Int. Ed., 2011, 50, 5361 CrossRef CAS PubMed.
- X.-Y. Chen, R.-C. Lin and S. Ye, Chem. Commun., 2012, 48, 1317 RSC.
- Y. Wang, Z.-H. Yu, H.-F. Zheng and D.-Q. Shi, Org. Biomol. Chem., 2012, 10, 7739 RSC.
- R. Na, C. Jing, Q. Xu, H. Jiang, X. Wu, J. Shi, J. Zhong, M. Wang, D. Benitez, E. Tkatchouk, W. A. Goddard III, H. Guo and O. Kwon, J. Am. Chem. Soc., 2011, 133, 13337 CrossRef CAS.
- X. Meng, Y. Huang and R. Chen, Org. Lett., 2009, 11, 137 CrossRef CAS PubMed.
- Z. Lu, S. Zheng, X. Zhang and X. Lu, Org. Lett., 2008, 10, 3267 CrossRef CAS PubMed.
- D. J. Wallace, R. L. Sidda and R. A. Reamer, J. Org. Chem., 2007, 72, 1051 CrossRef CAS PubMed.
-
(a) X.-F. Zhu, C. E. Henry, J. Wang, T. Dudding and O. Kwon, Org. Lett., 2005, 7, 1387 CrossRef CAS;
(b) R. Ma, S. Xu, X. Tang, G. Wu and Z. He, Tetrahedron, 2011, 67, 1053 CrossRef CAS;
(c) S. Xu, L. Zhou, R. Ma, H. Song and Z. He, Chem.–Eur. J., 2009, 15, 8698 CrossRef CAS.
- X. Guo, X. Chen, Y. Cheng, X. Chang, X. Li and P. Li, Org. Biomol. Chem., 2021, 19, 1727 RSC.
- L. Zhou, C. Wang, C. Yuan, H. Liu, C. Zhang and H. Guo, Org. Lett., 2018, 20, 6591 CrossRef CAS PubMed.
-
(a) Y. Zhu, D. Wang and Y. Huanf, Org. Lett., 2019, 21, 908 CrossRef CAS PubMed;
(b) V. R. Reddy, N. Maripally, R. Mutyala, J. B. Nanubolu and R. Chandra, Tetrahedron Lett., 2018, 59, 2631 CrossRef CAS;
(c) D. Wang, Z.-F. Song, W.-J. Wang and T. Xu, Org. Lett., 2019, 21, 3963 CrossRef CAS.
- M. Steurer, K. L. Jensen, D. Worgull and K. A. Jørgensen, Chem.–Eur. J., 2012, 18, 76 CrossRef CAS.
- A. Jose, K. C. S. Lakshmi, E. Suresh and V. Nair, Org. Lett., 2013, 15, 1858 CrossRef CAS PubMed.
- G. Zhu, Z. Chen, Q. Jiang, D. Xiao, P. Cao and X. Zhang, J. Am. Chem. Soc., 1997, 119, 3836 CrossRef CAS.
- For review articles, see:
(a) H. Ni, W.-L. Chan and Y. Lu, Chem. Rev., 2018, 118, 9344 CrossRef CAS PubMed;
(b) H. Guo, Y. C. Fan, Z. Sun, Y. Wu and O. Kwon, Chem. Rev., 2018, 118, 10049 CrossRef CAS PubMed; For selected examples, see:
(c) D. Wang, Y. Wei and M. Shi, Chem. Commun., 2012, 48, 2764 RSC;
(d) H. Xiao, Z. Chai, D. Cao, H. Wang, J. Chen and G. Zhao, Org. Biomol. Chem., 2012, 10, 3195 RSC;
(e) Y.-Q. Fang and E. N. Jacobsen, J. Am. Chem. Soc., 2008, 130, 5660 CrossRef CAS PubMed;
(f) Y. Du, X. Lu and Y. Yu, J. Org. Chem., 2002, 67, 8901 CrossRef CAS PubMed;
(g) W. Luo, B.-X. Shao, J. Li, X. Xiao, D. Song, F. Ling and W. Zhong, Org. Chem. Front., 2020, 7, 1016 RSC;
(h) P. Aillard, M. Gicquel, K. Yavari, P. Retailleau, A. Voituriez and A. Marinetti, Eur. J. Org. Chem., 2018, 2018, 5853 CrossRef CAS.
-
(a) O. W. Woltersdorf Jr, S. J. deSolms, G. E. Stokker and E. J. Cragoe Jr, J. Med. Chem., 1984, 27, 840 CrossRef CAS PubMed;
(b) S. Kotha and A. Fatma, Asian J. Org. Chem., 2021, 10, 129 CrossRef CAS;
(c) C. Bharkavi, S. V. Kumar, M. A. Ali, H. Osman, S. Muthusubramanian and S. Perumal, Bioorg. Med. Chem. Lett., 2017, 27, 3071 CrossRef CAS PubMed;
(d) A. C. Wei, M. A. Ali, Y. K. Yoon, R. Ismail, T. S. Choon, R. S. Kumar, N. Arumugam, A. I. Almansour and H. Osman, Bioorg. Med. Chem. Lett., 2012, 22, 4930 CrossRef CAS PubMed;
(e) S. Mathusalini, T. Arasakumar, K. Lakshmi, C.-H. Lin, P. S. Mohan, M. G. Ramnath and R. Thirugnanasampandan, New J. Chem., 2016, 40, 5164 RSC.
-
(a) M. Li, W. L. Yang, L. R. Wen and F. Q. Li, Eur. J. Org. Chem., 2008, 2008, 2751 CrossRef;
(b) S. J. Tu, B. Jiang, J. Y. Zhang, R. H. Jia, Y. Zhang and C. S. Yao, Org. Biomol. Chem., 2006, 4, 3980 RSC;
(c) D. Pizzirani, M. Roberti and M. Recanatini, Tetrahedron Lett., 2007, 48, 7120 CrossRef CAS;
(d) D. B. Ramachary, C. Venkaiah and P. M. Krishna, Chem. Commun., 2012, 48, 2252 RSC.
-
(a) A. Ding, M. Meazza, H. Guo, J. W. Yang and R. Rios, Chem. Soc. Rev., 2018, 47, 5946 RSC;
(b) E. Chupakhin, O. Babich, A. Prosekov, L. Asyakina and M. Krasavin, Molecules, 2019, 24, 4165 CrossRef CAS PubMed.
-
(a) D. B. Ramachary and P. M. Krishna, Asian J. Org. Chem., 2016, 5, 729 CrossRef CAS;
(b) U. Das, Y.-L. Tsai and W. Lin, Org. Biomol. Chem., 2013, 11, 44 RSC;
(c) J. Qi, J. Zheng and S. Cui, Org. Chem. Front., 2018, 5, 222 RSC;
(d) G. Zhan, M. L. Shi, Q. He, W. J. Lin, Q. Ouyang, W. Du and Y. C. Chen, Angew. Chem., Int. Ed., 2016, 55, 2147 CrossRef CAS PubMed;
(e) J. Duan, J. Cheng and P. Li, Org. Chem. Front., 2015, 2, 1048 RSC;
(f) N. L. Ahlburg, P. G. Jones and D. B. Werz, Org. Lett., 2020, 22, 6404 CrossRef CAS PubMed;
(g) Y.-R. Chen, M. R. Ganapuram, K.-H. Hsieh, K.-H. Chen, P. Karanam, S. S. Vagh, Y.-C. Liou and W. Lin, Chem. Commun., 2018, 54, 12702 RSC.
-
(a) R. Chen, X. Fan, J. Gong and Z. He, Asian J. Org. Chem., 2014, 3, 877 CrossRef CAS;
(b) F. Hu, Y. Wei and M. Shi, Tetrahedron, 2012, 68, 7911 CrossRef CAS;
(c) C.-Z. Liu, Y. Han, W.-J. Qi and C.-G. Yan, Heterocycl. Commun., 2016, 22, 301 CAS.
- W. Shi, B. Mao, J. Xu, Q. Wang, W. Wang, Y. Wu, X. Li and H. Guo, Org. Lett., 2020, 22, 2675 CrossRef CAS PubMed.
- E. Li, Y. Huang, L. Liang and P. Xie, Org. Lett., 2013, 15, 3138 CrossRef CAS PubMed.
- M. Rong, S. Gege, X. Qiuzhen, Y. Liu, L. Er-Qing and D. Zheng, Chin. J. Org. Chem., 2019, 39, 2196 CrossRef.
-
(a) S. Roy, M. Amireddy and K. Chen, Tetrahedron, 2013, 69, 8751 CrossRef CAS;
(b) H.-H. Kuan, C.-H. Chien and K. Chen, Org. Lett., 2013, 15, 2880 CrossRef CAS PubMed;
(c) S. Anwar, S. M. Li and K. Chen, Org. Lett., 2014, 16, 2993 CrossRef CAS PubMed;
(d) M. Amireddy and K. Chen, Tetrahedron, 2015, 71, 8003 CrossRef CAS;
(e) V. B. Gudise, P. C. Settipalli, E. K. Reddy and S. Anwar, Eur. J. Org. Chem., 2019, 2019, 2234 CrossRef CAS;
(f) Y. P. Reddy, V. B. Gudise, P. C. Settipalli and S. Anwar, ChemistrySelect, 2021, 6, 4456 CrossRef CAS.
- Detailed X-ray crystallographic data are available from CCDC, 12 Union Road, Cambridge CB2, 1EZ, UK for product 3e (CCDC-921839) and 4e (CCDC-921702)†.
- M. J. Frisch, G. W. Trucks, H. B. Schlegel, G. E. Scuseria, M. A. Robb, J. R. Cheeseman, G. Scalmani, V. Barone, B. Mennucci, G. A. Petersson, H. Nakatsuji, M. Caricato, X. Li, H. P. Hratchian, A. F. Izmaylov, J. Bloino, G. Zheng, J. L. Sonnenberg, M. Hada, M. Ehara, K. Toyota, R. Fukuda, J. Hasegawa, M. Ishida, T. Nakajima, Y. Honda, O. Kitao, H. Nakai, T. Vreven, J. A. Montgomery, J. E. Peralta Jr, F. Ogliaro, M. Bearpark, J. J. Heyd, E. Brothers, K. N. Kudin, V. N. Staroverov, R. Kobayashi, J. Normand, K. Raghavachari, A. Rendell, J. C. Burant, S. S. Iyengar, M. Tomasi, N. Cossi, J. M. Rega, M. Millam, J. E. Klene, J. B. Knox, V. Cross, C. Bakken, J. Adamo, J. Jaramillo, R. Gomperts, R. E. Stratmann, O. Yazyev, A. J. Austin, R. Cammi, C. Pomelli, J. W. Ochterski, R. L. Martin, K. Morokuma, V. G. Zakrzewski, G. A. Voth, P. Salvador, J. J. Dannenberg, S. Dapprich, A. D. Daniels, O. Farkas, J. B. Foresman, J. V. Ortiz, J. Cioslowski and D. J. Fox, Revision A. 02, Gaussian Inc., Wallingford CT, 2009 Search PubMed.
-
(a) A. D. Becke, Phys. Rev. A, 1993, 98, 5648 CAS;
(b) C. Lee, W. Yang and R. G. Parr, Phys. Rev. B: Condens. Matter Mater. Phys., 1988, 37, 785 CrossRef CAS PubMed.
Footnote |
† Electronic supplementary information (ESI) available: Experimental procedure, spectral data of products 3/4 and X-ray crystallographic data for 3e and 4e. CCDC 921839, 921702 and 2110327–2110330. For ESI and crystallographic data in CIF or other electronic format see DOI: 10.1039/d1ra07165c |
|
This journal is © The Royal Society of Chemistry 2021 |
Click here to see how this site uses Cookies. View our privacy policy here.