DOI:
10.1039/D1RA06923C
(Paper)
RSC Adv., 2021,
11, 37436-37442
Synthesis of butadiene/isoprene–styrene di-block copolymer with high cis-1,4 unit content based on a neodymium phosphate ester†
Received
15th September 2021
, Accepted 14th November 2021
First published on 19th November 2021
Abstract
A novel two-step synthesis strategy based on a liquid neodymium phosphate ester (Nd(P507)3) catalyst was used to synthesize diene-styrene di-block copolymers with high cis-1,4 unit content, such as butadiene–styrene (PB-b-PS), isoprene–styrene (PI-b-PS), and so on. The strategy not only makes full use of the high cis-1,4 stereo-selectivity of the rare earth catalyst to conjugated dienes, but also adjusts the electron cloud density of the catalytic active center by introducing triphenyl phosphine (PPh3). Thus, the catalytic activity of the neodymium-based catalyst center toward styrene has been largely improved, and a series of PB/PI-b-PSs with high cis-1,4 unit content (98.3 and 98.1% respectively), narrow molecular weight distribution and controllable block ratio were successfully synthesized. Herein, molecular weight (Mn), molecular weight distribution (Mw/Mn) and microstructure of the block polymers were characterized using SEC-MALLS, FT-IR, 1H NMR, 13C NMR and DSC. The DSC curve of a PB-b-PS with 98.3% cis-1,4 unit and 18.6% polystyrene content shows a Tg of −103.1 °C, which means it has relatively excellent mechanical properties at lower temperature. The results show that these PB/PI-b-PS materials have good application prospects in harsh low temperature environments.
1. Introduction
Butadiene/isoprene–styrene block copolymers (PB/PI-b-PS) are one of the most important thermoplastic elastomer varieties, and have excellent mechanical and thermal properties, due to their unique macromolecular structure with both hard and soft segments.1–6 It is reported in the literature that by increasing the cis-1,4 unit content in PB or PI segments, the homopolymer or copolymer's low temperature resistance and mechanical properties such as the tensile strength and elongation ratios significantly improved.7,8 At present, the industrial synthesis of PB/PI-b-PS mainly uses alkyllithium-based anionic polymerization methods, resulting in a precisely controlled molecular weight and a narrow molecular weight distribution. However, the product's stereo-structure still could not be sufficiently controlled by these alkyllithium-based catalytic system and the cis-1,4 structure content of the butadiene and isoprene segments in the copolymer were lower than 50% and 90%9,10 respectively. Furthermore, many kinds of synthesis strategies, such as ring-opening polymerization of ectopic (ROMP)11 and reversible addition–fragmentation chain transfer polymerization (RAFT),12 are also used to prepared the block copolymer PB/PI-b-PS. However, the stereo-structure of PB in the result polymers still could not be effectively controlled. As a result, it obviously limits the application of such polymers in certain specific fields such as low temperature environment.
A large number of literature reports indicate that the coordination polymerization method can effectively control the stereo-structure of conjugated diene, especially the rare earth catalytic system, has extremely high catalytic activity and stereo-selectivity to butadiene and isoprene.13–15 Unfortunately, due to the electron-withdrawing effect of the benzene ring in styrene, the electron cloud density of the C
C in styrene is significantly different from that of butadiene and isoprene. As a result, the coordination catalytic system that can effectively catalyze the polymerization of butadiene or isoprene monomer is not necessarily suitable for the polymerization of styrene.16–18 Therefore, it is very difficult to synthesize butadiene–styrene or isoprene–styrene block copolymer with high cis-1,4 structure content via a single catalytic system. Recently, numerous studies have focused on the synthesis of butadiene/isoprene–styrene di-block and tri-block copolymers using coordination polymerization systems. For instance, Shen et al.18,19 reported that the catalytic system NdCl3*HMPA/Al(i-Bu)3 has high activity for styrene, and the copolymer with isoprene possessing high 1,4-unit content (>90%) was synthesized. Ban et al.20 have reported that styrene–butadiene tri-block copolymers (SBS) with 70% cis-1,4 unit content could be synthesized successfully using metallocene-titanium/methylaluminoxane (MAO), but this metallocene catalytic system has very low catalytic activity toward butadiene monomer. Cui et al.5,16 synthesized PS-b-cis-PB (cis-1,4 >97%) and PS-b-cis-PI (cis-1,4 >80%) respectively via a linked-half-sandwich lutetium-bis(allyl)/[Ph3C][B(C6F5)4] catalyst system, but its industrial application will be greatly restricted by the expensive raw material of main catalyst and co-catalyst.
In this article, a series of PB/PI-b-PSs di-block copolymer with high cis-1,4 unit content were synthesized by a novel synthetic strategy based on a phosphate ester neodymium (Nd(P507)3). Firstly, the polymerization of butadiene (Bd) or isoprene (Ip) initiated by a ternary homogeneous catalytic system consisting of Nd(P507)3 (Nd), Al(i-Bu)3 (Al) and CHCl3 (Cl). After that, triphenyl phosphine (PPh3) was used to adjust the electron density of the catalytic active center, and enhance the coordination ability between active center and styrene. Lastly, styrene monomer was added to obtain the PB/PI-b-PS with high cis-1,4 unit content via this novel Nd-based catalytic system (Nd(P507)3/Al(i-Bu)3/CHCl3/PPh3). Taking PB-b-PS as an example, the synthesis process of block copolymer with high cis-1,4 unit content are illustrated in Scheme 1.
 |
| Scheme 1 Synthesis route of PB-b-PS via catalyst system Nd(P507)3/Al(i-Bu)3/CHCl3/PPh3. | |
2. Experimental
2.1 Materials
Isoprene (Ip, 99%) and styrene (St, 99%) were purchased from Macklin China, and diluted to 2 M in cyclohexane after vacuum distillation with CaH2. 1,3-Butadiene (Bd, 1.9 M in hexane) was obtained from Energy China and used as received. Neodymium 2-ethylhexyl hydrogen-2-ethylhexylphosphonate solution (Nd(P507)3, 0.04 M in n-hexane) was provided by Foshan Shunde Change Material Co., Ltd. Diisobutylaluminum hydride (Al(i-Bu)3, 1 M in n-hexane), triphenyl phosphine (PPh3, 97%) and 2,6-di-tert-butyl-p-cresol (Antiager 264, 97%) were purchased from Macklin China and used as received. Cyclohexane was distilled with CaH2 and stored on 4 Å molecular sieves for one week before used. Chloroform (99%), n-hexane (98%), ethanol (99.5%) and butanone (99%) were used without further purification.
2.2 Synthesis procedure for PB-b-PS and PI-b-PS
All synthesis operations were performed in a dry argon atmosphere. A detailed polymerization procedure (as shown in Table 1) is described as a typical example. Specifically, Nd(P507)3 solution (Nd, 0.01 mmol, 0.04 M in cyclohexane), butadiene (Bd, 0.2 mmol, 2 M in n-hexane), Al(i-Bu)3 (Al, 0.2 mmol, 1 M in n-hexane) were injected into a Schlenk tube with a rubber septum sequentially by a syringe. The above mixture was aged at 50 °C with continuous stirring. After 15 min, CHCl3 (Cl, 0.03 mmol) was injected and aged for another 15 min, and a yellow transparent catalyst solution was obtained.
Table 1 Synthesis of PB-b-PS via Nd(P507)3/Al(i-Bu)3/CHCl3/PPh3a
Entry |
Monomer |
Conv.b (%) |
St cont.c (%) |
Mnd*104 (g mol−1) |
Mw/Mnd |
cis-1,4e (%) |
trans-1,4e (%) |
1,2-e (%) |
1, 2 correspond to the first, second polymerization steps. First step, [Nd] = 0.01 mmol, catalyst ratio: [Nd]/[Bd]/[Al]/[Cl] = 1 : 20 : 20 : 3, [Bd]/[Nd] = 1000, second step, [PPh3]/[Nd] = 1, [St]/[Nd] = 250. Conversion of monomer in each polymerization steps. Measured by 1H NMR. Measured by SEC-MALLS. Measured by 1H NMR and 13C NMR. |
1 |
Bd |
98.2 |
— |
5.6 |
1.21 |
98.3 |
0.7 |
1.0 |
2 |
St |
81.5 |
18.6 |
7.8 |
1.39 |
98.3 |
0.7 |
1.0 |
Then, the butadiene solution (10 mmol, 1.9 M in hexane, [Bd]/[Nd] = 1000) were injected into the Schlenk tube with the preformed catalyst solution using a syringe. The polymerization was carried out at 50 °C for 3 h. Then, triphenyl phosphine (PPh3, 0.01 mmol, [PPh3]/[Nd] = 1) was added, and 15 min later, styrene solution (St, 2.5 mmol, 2 M in cyclohexane, [St]/[Nd] = 250) was added. This polymerization was carried out at 50 °C for 5 h under stirring, and then quenched by ethanol containing antiager 264 (1 wt%) as a stabilizer. The result product was precipitated in ethanol and washed with ethanol repeatedly, then extracted with butanone and n-hexane three times each. Finally, the product was dried under vacuum at 40 °C until a constant weight of a white solid was obtained. The experimental procedure for the synthesis of PI-b-PS is the same as that described for PB-b-PS.
2.3 Characterization
1H NMR and 13C NMR were recorded with Bruker 400 MHz using CDCl3 as the solvent. The ratio of 1,4- and 1,2-unit content of PB, and ratio of cis-1,4 and 3,4-unit content of PI were determined by 1H NMR, and the ratio of cis-1,4 and trans-1,4 unit content of PB was determined by 13C NMR.21–24 In addition, the actual ratio of the PS and PB/PI segments in the copolymer was also estimated by 1H NMR, according to a previously published method.22–25 FT-IR spectra were measured using a Bruker Nicolet iS10 spectrophotometer. The number-average molecular weights (Mn) and dispersity (Mw/Mn) of the polymers were measured by a DAWN EOS size exclusion chromatography/multi-angle light scatter instrument (SEC-MALLS, Wyatt Technology), the polymer samples were dissolved in HPLC grade THF with the concentration of 10.0 mg ml−1, flow rate of eluent was 0.5 ml min−1.
3. Results and discussion
3.1 Synthesis of cis-PB-b-PS via Nd(P507)3/Al(i-Bu)3/CHCl3/PPh3
3.1.1 Synthesis and structure characterization of cis-PB-b-PS. The ternary neodymium-based catalyst system Nd(P507)3/Al(i-Bu)3/CHCl3 exhibits extremely high catalytic activity and cis-1,4 stereo-selectivity (cis-1,4 > 98%) for butadiene,27,28 as shown in Table 1. However, the catalyst system with a PB segment had almost no catalytic activity towards styrene (as shown in entry 1, Table 2), which makes it difficult to obtain a block copolymer with a controlled content of styrene segment. After an equimolar amount of PPh3 ([PPh3]/[Nd] = 1
:
1) was added to adjust the electron density around of Nd atom in the catalyst before the polymerization of the styrene, the catalytic activity of this Nd-based catalytic system (Nd(P507)3/Al(i-Bu)3/CHCl3/PPh3 for styrene has significantly improved, the conversion rate of styrene monomer increases greatly correspondingly. Finally, a series of PB-b-PS di-block copolymer with high cis-1,4 unit content (=98.3%) was prepared successfully. In addition, the PB-b-PS obtained has a narrow molecular weight distribution (Mw/Mn). Because the liquid neodymium phosphate catalyst used in this article has good solubility in the aliphatic solvent, and the monomer could be polymerized in a completely homogeneous state.29,30 Consequently, the homopolymer PB and di-block PB-b-PS with narrower molecular weight distribution could be successfully achieved.
Table 2 The effect of [PPh3]/[Nd] in the synthesis of PB-b-PSsa
Entry |
[PPh3]/[Nd] |
Conv.b (%) |
St cont.c (%) |
Mnd*104 (g mol−1) |
Mw/Mnd |
cis-1,4e (%) |
First step, [Nd] = 0.01 mmol, [Nd]/[Bd]/[Al]/[Cl] = 1 : 20 : 20 : 3, [Bd]/[Nd] = 1000. Second step, [St] = 5 mmol. Conversion of monomer Bd in the second step. Determined by the 1H NMR spectrum. Measured by SEC-MALLS. Determined by the 1H NMR and 13C NMR spectrum. |
1 |
0 |
3.9 |
1.8 |
5.6 |
1.25 |
98.3 |
2 |
0.5 |
46.7 |
10.5 |
6.5 |
1.41 |
98.2 |
3 |
1 |
81.5 |
18.6 |
7.8 |
1.39 |
98.3 |
4 |
1.5 |
59.6 |
13.9 |
6.9 |
1.45 |
98.3 |
5 |
2.0 |
15.3 |
3.7 |
5.8 |
1.28 |
98.2 |
As shown in Fig. 1, the chemical shifts at 5.40 ppm and 5.05 ppm correspond to the 1,4- and 1,2-structures of the PB segment, whereas the chemical shifts between 6.25 and 7.25 ppm correspond to phenyl group in the PS segment.23,25 The appearance of the phenyl peak of the products obtained in the second steps indicates that the di-block copolymer PB-b-PS was successfully synthesized. In addition, according to the method introduced in the literature,23,28 the integral calculation of the 1H NMR peaks shows that the content of styrene in the polymer is 18.6%.
 |
| Fig. 1 1H NMR spectra of the polymers in each polymerization steps of PB-b-PS. | |
The SEC curves of PB and PB-b-PS obtained from each synthetic steps are shown in Fig. 2. When the styrene monomer was added in the second polymerization step, the PB-b-PS curve was shifted to a higher molecular weight region and maintained monomodal shape. It was indicated that there was no homopolymer PB in the PB-b-PS copolymer samples, and a PB-b-PS di-block polymer was successfully prepared.
 |
| Fig. 2 SEC curves of polymers in each polymerization steps of PB-b-PS. | |
As shown in Fig. 3 and 4, the FT-IR and 13C NMR spectra of the PB-b-PS (entry3, in Table 2) prepared using the Nd(P507)3/Al(i-Bu)3/CHCl3/PPh3 catalytic system are presented respectively. The FT-IR spectrum exhibited two obvious absorption peaks at 695 cm−1 and 1505 cm−1, which correspond to the out-of-plane deformation vibrations and skeleton stretching vibrations of phenyl group in the PS segment of PB-b-PS. The peak at 750 cm−1, 967 cm−1 and 911 cm−1 represent of cis-1,4, trans-1,4 and 1,2-unit in the PB segment and the data revealed that the di-block copolymer has very high cis-1,4 PB unit content (cis-1,4 >98%).23,26 In the Fig. 4, the chemical shifts at 27.5 ppm, 32.6 ppm, 34.2 ppm and 145.2 ppm represent the cis-1,4, trans-1,4 and 1,2-PB segment and PS segment, respectively. Which also indicated that the PB-b-PS polymer obtained containing a relatively higher cis-1,4 unit content (>98%).
 |
| Fig. 3 FT-IR spectra of PB-b-PS via Nd(P507)3/Al(i-Bu)3/CHCl3/PPh3 catalytic system. | |
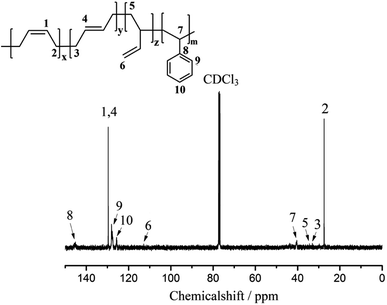 |
| Fig. 4 13C NMR spectra of PB-b-PS via Nd(P507)3/Al(i-Bu)3/CHCl3/PPh3 catalytic system. | |
The DSC curve of sample PB-b-PS (entry 2, in Table 1) was shown in Fig. 5. The PB-b-PS with high cis-1,4 unit content (98.3%) showed a Tg of −103.1 °C, which is very close to the Tg of Nd-based cis-butadiene rubber,15 and was notably lower than the Tg of industrial SBS prepared by the initiation of the butyllithium (approx. −80 °C). The data indicate that PB-b-PS prepared via Nd(P507)3/Al(i-Bu)3/CHCl3/PPh3 catalytic system could maintain a better flexibility at an extremely low temperature, which means a relatively excellent mechanical properties at the lower temperature.15,27
 |
| Fig. 5 DSC curve of PB-b-PS via Nd(P507)3/Al(i-Bu)3/CHCl3/PPh3 catalytic system. | |
The proposed mechanism of block polymerization of butadiene and styrene is shown in Scheme 2, according to literature reports.31–36 Firstly, butadiene was homopolymerized using a ternary neodymium-based catalytic system (Nd(P507)3/Al(i-Bu)3/CHCl3/PPh) via a coordination mechanism. The η3-π-allyl Nd-based catalytic active center (as shown in Scheme 2) was formed with good stability and solubility in aliphatic solvents, which has relatively high catalytic activity and cis-1,4 stereo-selectivity for butadiene or isoprene.14,25,36 However, this neodymium-based active center has almost no catalytic activity for styrene, after initiating the polymerization of butadiene. In order to realize the block copolymerization of styrene and butadiene, a certain amount of triphenyl phosphine ([PPh3]/[Nd] = 1
:
1) was added into the catalytic system, to adjust the electron density around the Nd atom.27,32 Because the π-electron of the electron donor PPh3 could flow to the styrene monomer through the metal atom, the coordination ability between styrene and catalytic active center is greatly increased.15,36 Then the catalytic activity of the catalytic system towards styrene showed a significant improvement, and the conversion of styrene reached over than 80%, as shown in Table 2. Based on this mechanism of adjusting the electron cloud density of the around the rare earth atom in the catalytic active center, the difficult of the large different catalytic activity of styrene and butadiene via a single coordination catalyst system was overcome, and a series of di-block copolymer PB-b-PS with a high cis-1,4 unit content was synthesized successfully.
 |
| Scheme 2 Synthesis mechanism of PB-b-PS via Nd(P507)3/Al(i-Bu)3/CHCl3/PPh3 catalytic system. | |
3.1.2 Effect of the ratio of [PPh3]/[Nd]. It can be seen from Table 2, if PPh3 is not added, the catalytic system after initiating the homopolymerization of butadiene, has almost no catalytic activity for styrene. As the amount of PPh3 increases, the conversion rate of styrene in the second polymerization stage increases gradually. When the ratio of [PPh3]/[Nd] = 1, the catalytic activity of the catalytic system towards styrene was the highest, which allowed a conversion rate of styrene to reach values higher than 80%. However, when the amount of PPh3 continues to increase, the catalytic activity of the system for styrene appears to decrease. This may be because an excessive amount of electron donors coordinated with Nd atoms, which caused the positive charge of the metal atoms to decrease too much, thereby losing the coordination ability to styrene.
3.1.3 Effect of the monomer feed ratio of [St]/[Bd]. Table 3 presented the macromolecular characteristics of a series of PB-b-PSs obtained using Nd(P507)3/Al(i-Bu)3/CHCl3/PPh3 with different styrene content and molecular weight, by changing the molar feed ratio of butadiene verse styrene monomer. Obviously, by changing the feeding amount and feed ratio of the two monomers, the molecular weight and styrene content of the block copolymer can be effectively controlled, and its cis-1,4 structure content is always maintained at more than 98%.
Table 3 Effect of [Bd]/[Nd] and [St]/[Nd] ratios in the synthesis of PB-b-PSsa
Entry |
[Bd]/[Nd] |
[St]/[Nd] |
Conv.b (%) |
St cont.c (%) |
Mnd*104 (g mol−1) |
Mw/Mnd |
cis-1,4e (%) |
First step, [Nd] = 0.01 mmol, [Nd]/[Bd]/[Al]/[Cl] = 1 : 20 : 20 : 3. Second step, [PPh3]/[Nd] = 1. Conversion of monomer styrene in the second step. Determined by the 1H NMR spectrum. Measured by SEC-MALLS. Determined by the 1H NMR and 13C NMR spectrum. |
1 |
500 |
250 |
83.3 |
31.3 |
4.6 |
1.34 |
98.3 |
2 |
1000 |
250 |
81.5 |
18.6 |
7.8 |
1.39 |
98.3 |
3 |
1500 |
250 |
78.5 |
12.8 |
10.3 |
1.40 |
98.2 |
4 |
2000 |
250 |
72.9 |
10.6 |
12.9 |
1.38 |
98.3 |
5 |
500 |
125 |
84.1 |
19.1 |
4.1 |
1.32 |
98.2 |
6 |
2000 |
500 |
80.2 |
18.0 |
16.9 |
1.49 |
98.4 |
3.2 Synthesis of cis-PI-b-PS via Nd(P507)3/Al(i-Bu)3/CHCl3/PPh3
3.2.1 Synthesis and structure characterization of cis-PI-b-PS. The Nd(P507)3/Al(i-Bu)3/CHCl3/PPh3 was applied in the copolymerization of the isoprene and styrene. Under the same synthetic strategy aforementioned as the cis-1,4-PB-b-PS, the di-block copolymer PI-b-PS with a high cis-1,4 content (cis-1,4 = 98.1%) was also obtained. The specific data were shown in Table 4.
Table 4 Synthesis of PI-b-PS via Nd(P507)3/Al(i-Bu)3/CHCl3/PPh3 catalytic systema
Entry |
Monomer |
Conv.b (%) |
St cont.c (%) |
Mnd*104 (g mol−1) |
Mw/Mnd |
cis-1,4e (%) |
3,4-e (%) |
1, 2 correspond to the first, second polymerization steps. First step, [Nd] = 0.01 mmol, catalyst ratio: [Nd]/[Ip]/[Al]/[Cl] = 1 : 20 : 20 : 3, [Ip]/[Nd] = 1000, second step, [PPh3]/[Nd] = 1, [St]/[Nd] = 250. Conversion of monomer in each polymerization steps. Measured by 1H NMR. Measured by SEC-MALLS. Measured by 1H NMR. |
1 |
Ip |
97.5 |
— |
7.0 |
1.21 |
98.1 |
1.9 |
2 |
St |
79.8 |
18.3 |
9.3 |
1.35 |
98.1 |
1.9 |
The 1H NMR and FT-IR spectra of the PI-b-PS prepared via two steps with Nd(P507)3/Al(i-Bu)3/CHCl3/PPh3 catalytic systems are presented in Fig. 6 and 7, respectively. In Fig. 6, the chemical shifts at 5.20 ppm and 4.75 ppm correspond to the cis-1,4 and 3,4-structures of polyisoprene, the chemical shifts between 6.25 and 7.25 ppm represent the phenyl group in the PS segment. These data confirmed that PI-b-PS had been successfully synthesized via the Nd(P507)3/Al(i-Bu)3/CHCl3/PPh3 catalytic systems. In addition, as per previous reports,23,26 the integration of these chemical shifts indicate that the polyisoprene contains a higher amount of cis-1,4 units. As shown in Fig. 7, the FT-IR spectrum exhibited two distinct absorption bands at 698 cm−1 corresponding to the out-of-plane deformation vibrations of phenyl group in the PS segment of PB-b-PS the PI-b-PS, and the results from FT-IR spectra show a strong absorption peak at 1370 cm−1, but the absorption peaks at 888 cm−1 (3,4-units) are very weak, demonstrate that the PI-b-PS was predominantly contained cis-1,4 units.23,27
 |
| Fig. 6 1H NMR spectra of PI-b-PS via Nd(P507)3/Al(i-Bu)3/CHCl3/PPh3 catalytic system. | |
 |
| Fig. 7 FT-IR spectra of PI-b-PS via Nd(P507)3/Al(i-Bu)3/CHCl3/PPh3 catalytic system. | |
3.2.2 Effect of the monomer feed ratio of [St]/[Ip]. As shown in Table 5, a series of PI-b-PSs with different styrene content and molecular weight, were also obtained base on the Nd(P507)3/Al(i-Bu)3/CHCl3/PPh3, by changing the changing the monomer feed amount and ratio. The molecular weight and styrene content of the block copolymer can also be effectively controlled, and cis-1,4 unit content of the obtained PI-b-PS are always maintained at more than 98%.
Table 5 Effect of [Ip]/[Nd] and [St]/[Nd] ratios in the synthesis of PI-b-PSa
Entry |
[IP]/[Nd] |
[St]/[Nd] |
Conv.b (%) |
St cont.c (%) |
Mnd*104 (g mol−1) |
Mw/Mnd |
cis-1,4e (%) |
First step, [Nd] = 0.01 mmol, [Nd]/[Ip]/[Al]/[Cl] = 1 : 20 : 20 : 3. Second step, [PPh3]/[Nd] = 1. Conversion of monomer styrene in the second step. Determined by the 1H NMR spectrum. Measured by SEC-MALLS. Determined by the 1H NMR spectrum. |
1 |
500 |
250 |
80.6 |
29.3 |
5.6 |
1.38 |
98.1 |
2 |
1000 |
250 |
79.8 |
18.3 |
9.3 |
1.35 |
98.1 |
3 |
1500 |
250 |
76.6 |
11.2 |
12.8 |
1.46 |
98.2 |
4 |
2000 |
500 |
74.5 |
16.9 |
17.9 |
1.63 |
98.2 |
5 |
3000 |
750 |
60.2 |
14.5 |
25.6 |
2.28 |
98.2 |
4. Conclusion
In this study, a novel two-step synthesis strategy based on a liquid phosphate ester neodymium (Nd(P507)3) catalyst was used to synthesize conjugated diene with high cis-1,4 unit content, such as butadiene–styrene (PB-b-PS), isoprene–styrene (PI-b-PS) di-block copolymer, and so on. Firstly, the polymerization of butadiene (Bd) or isoprene (Ip) was initiated by a ternary catalytic system consisting of liquid Nd(P507)3 (Nd), Al(i-Bu)3 (Al) and CHCl3 (Cl). Then, styrene (St) monomer was added after the electron density of the active center adjusting by triphenyl phosphine (PPh3). Finally, a series of PB/PI-b-PSs with high cis-1,4 unit content (98.3% and 98.1% respectively), narrow molecular weight distribution and controllable block ratio were successfully synthesized. The DSC curve of a PB-b-PS with 98.3% cis-1,4 unit and 18.6% polystyrene content shows a Tg of −103.1 °C, which means it has a relatively excellent mechanical properties at the lower temperature. The result shows these PB/PI-b-PSs materials have good application prospects in harsh low temperature environment.
Conflicts of interest
There are no conflicts to declare.
Acknowledgements
The authors gratefully acknowledge the financial support from National Natural Science Foundation of China (No. 52003148), Key Scientific Research Project of Education Department of Shaanxi Province (No. 21JS005), Scientific and technological research projects of Jiangxi Education Department (No. GJJ201838), the Open Project of Shaanxi Key Laboratory of Macromolecular Science and Technology, China (No. LMST2020KF-03), and the start-up funds of Shaanxi University of Technology, China (No. SLGRCQD2004). The authors declare no competing financial interest.
References
- F. Lin, M. Y. Wang, Y. P. Pan, T. Tang, D. M. Cui and B. Liu, Macromolecules, 2017, 50, 849–856 CrossRef CAS.
- I. Lee and F. S. Bates, Macromolecules, 2013, 46, 4529–4539, DOI:10.1021/ma400479b.
- J. M. Widin, A. K. Schmitt, A. L. Schmitt, K. Im and M. K. Mahanthappa, J. Am. Chem. Soc., 2012, 134, 3834–3844 CrossRef CAS PubMed.
- I. V. Vasilenko, H. Y. Yeong, M. Delgado, S. Ouardad, F. Peruch, B. Voit, F. Ganachaud and S. V. Kostjuket, Angew. Chem. Int. Ed., 2015, 54, 12728–12732 CrossRef CAS PubMed.
- Z. B. Jian, S. J. Tang and D. M. Cui, Macromolecules, 2011, 44, 7675–7681 CrossRef CAS.
- M. Nishiura, F. Guo and Z. M. Hou, Acc. Chem. Res., 2015, 48, 2209–2220 CrossRef CAS PubMed; L. X. Zhang, T. Suzuki, Y. Luo, M. Nishiura and Z. M. Hou, Angew. Chem. Int. Ed., 2007, 119, 1941–1945 CrossRef.
- H. Leicht, I. Göttker-Schnetmann and S. Mecking, Macromolecules, 2017, 50, 8464–8468 CrossRef CAS.
- M. Lanzi, L. Paganin, F. P. Di-Nicola and C. Trombini, J. Polym. Res., 2015, 22, 208 CrossRef.
- W. Gao and D. M. Cui, J. Am. Chem. Soc., 2008, 130, 4984–4991 CrossRef CAS PubMed.
- R. Z. Wei, Y. W. Luo, Z. Wang, F. Z. Wang and S. H. Xu, Ind. Eng. Chem. Res., 2012, 51, 15530–15535 CrossRef CAS.
- C. W. Bielawski, T. Morita and R. H. Grubbs, Macromolecules, 2000, 33, 678–680 CrossRef CAS.
- Q. Q. Dai, X. Q. Zhang, Y. M. Hu, J. He, C. Shi, Y. Q. Li and C. X. Bai, Macromolecules, 2017, 50, 7887–7894 CrossRef CAS.
- C. L. Fan, C. X. Bai, H. G. Cai, Q. Q. Dai, X. Q. Zhang and F. S. Wang, J. Polym. Sci., Part A: Polym. Chem., 2010, 48, 4768–4774 CrossRef CAS.
- L. Friebe, O. Nuyken and W. Obrecht, Neodymium-Based Ziegler/Natta Catalysts and their Application in Diene Polymerization. Springer: Berlin, 2006, pp. 101–168 Search PubMed.
- Z. B. Jian, S. J. Tang and D. M. Cui, Chem.–Eur. J., 2010, 16, 14007–14015 CrossRef CAS PubMed.
- S. Kaita, Z. M. Hou and Y. Wakatsuki, Macromolecules, 2001, 34, 1539–1541 CrossRef CAS.
- M. Jiménez-Tenorio, M. C. Puerta, I. Salcedo, P. Valerga, S. I. Costa, L. C. Silva and P. T. Gomeset, Organometallics, 2004, 23, 3139–3146 CrossRef.
- Q. S. Zhang, X. F. Ni, Y. F. Zhang and Z. Q. Shen, Macromol. Rapid Commun., 2001, 22, 1493–1496 CrossRef CAS.
- X. O. Xu, X. F. Ni and Z. Q. Shen, Chin. J. Chem., 2004, 22, 764–767 CrossRef CAS.
- H. T. Ban, T. Kase, M. Kawabe, A. Miyazawa and T. Shiono, Macromolecules, 2006, 39, 171–176 CrossRef CAS.
- R. N. Kularatne, A. Yang, H. Q. Nguyen, G. T. McCandless and M. C. Stefan, Macromol. Rapid Commun., 2017, 38, 1700427 CrossRef PubMed.
- F. Guo, R. Meng, Y. Li and Z. M. Hou, Polymer, 2015, 76, 159–167 CrossRef CAS.
- P. Li, K. Y. Zhang and M. Nishiura, Angew. Chem. Int. Ed., 2011, 50, 12012–12015 CrossRef PubMed.
- H. Zhu, Y. X. Wu, J. W. Zhao, Q. L. Guo and Q. G. Huang, J. Appl. Polym. Sci., 2007, 106, 103–109 CrossRef CAS.
- C. Y. Ren, G. L. Li, W. M. Dong, L. S. Jiang, X. Q. Zhang and F. S. Wang, Polymer, 2007, 48, 2470–2474 CrossRef CAS.
- X. Min and X. D. Fan, Macromol. Chem. Phys., 2018, 219, 1800479 CrossRef.
- F. Wang, C. Y. Zhang, Y. M. Hu, X. Y. Jia, C. X. Bai and X. Q. Zhang, Polymer, 2012, 53, 6027–6032 CrossRef CAS.
- K. Lv and D. M. Cui, Organometallics, 2010, 29, 2987–2993 CrossRef CAS.
- I. E. Nifant'ev, A. N. Tavtorkin, S. A. Korchagina, I. F. Gavrilenko, N. N. Glebova, N. N. Kostitsyna, V. A. Yakovlev, G. N. Bondarenko and M. P. Filatova, Appl. Catal., A, 2014, 478, 219–227 CrossRef.
- L. Friebe, O. Nuyken and W. Obrecht, J. Macromol. Sci., Part A: Pure Appl.Chem., 2005, 42, 839–851 CrossRef.
- R. Taube, H. Windisch and S. Maiwald, Macromol. Symp., 1995, 89, 393–409 CrossRef CAS.
- G. Ricci, A. Sommazzi, F. Masi, M. Ricci, A. Boglia and G. Leone, Coord. Chem. Rev., 2010, 254, 661–676 CrossRef CAS.
- F. M. B. Coutinho, T. C. J. Rocha, I. L. Mello, D. S. S. Nunes and M. A. S. Costa, J. Appl. Polym. Sci., 2005, 98, 2539–2543 CrossRef CAS.
- J. R. Ascenso, A. R. Dias, P. T. Gomes, C. C. RomaO, I. Tkatchenko, A. Revillon and Q. T. Phamet, Macromolecules, 1996, 29, 4172–4179 CrossRef CAS.
- K. Ohno, A. Nagasawa and T. Fujihara, Dalton Trans., 2015, 44, 368–376 RSC.
- Z. Jian, D. Cui, Z. Hou and X. Li, Chem. Commun., 2010, 46, 3022 RSC.
Footnotes |
† Electronic supplementary information (ESI) available. See DOI: 10.1039/d1ra06923c |
‡ Co-first author. |
|
This journal is © The Royal Society of Chemistry 2021 |
Click here to see how this site uses Cookies. View our privacy policy here.