DOI:
10.1039/D1RA05736G
(Paper)
RSC Adv., 2021,
11, 29661-29667
Chlorinated metabolites with antibacterial activities from a deep-sea-derived Spiromastix fungus†
Received
27th July 2021
, Accepted 20th August 2021
First published on 3rd September 2021
Abstract
Chromatographic separation of the solid cultures of a deep-sea-derived Spiromastix fungus (MCCC 3A00308) resulted in the isolation of eight compounds. Their structures were identified on the basis of the spectroscopic data. Compounds 1–8 are classified as depsidone-type (1–4), isocoumarin-type (5 and 6), and benzothiazole-type (7 and 8), of which 1–7 are new compounds and 1–3 along with 5 and 6 are chlorinated. Compound 3 is characterized by trichlorination and shows potent activities against Gram-positive pathogenic bacteria including Staphylococcus aureus ATCC 25923, Bacillus thuringiensis ATCC 10792, and Bacillus subtilis CMCC 63501, with minimum inhibitory concentration (MIC) values ranging from 0.5 to 1.0 μg mL−1. This study extends the chemical diversity of chlorinated natural products from marine-derived fungi and provides a promising lead for the development of antibacterial agents.
1. Introduction
Marine-occurring halogenated compounds are widely distributed in macroorganisms (algae,1–3 sponges,4–7 corals,8,9 and tunicates10,11) and microorganisms (fungi and bacteria)12–19 that inhabit extreme marine environments. Halometabolites play a crucial role in pharmaceutical and agriculture applications. It is estimated that approximately 25% of clinically used medicines are halogenated, demonstrating a significant contribution of halogen atoms to bioactivity. Well-known halogenated medicines include the antibiotics chloramphenicol,20 vancomycin,21 and rebeccamycin,22 which are clinically used for their antibacterial and antitumor properties. Compared to those from marine macroorganisms, bioactive halometabolites from marine microorganisms are relatively unexplored. Nevertheless, a number of brominated and chlorinated resorcylides, i.e., resorcylic acid lactones, with structural novelty have been isolated from marine-derived fungus.23 In addition, marine-derived bacteria are a promising source for the production of halometabolites, as exemplified by the napyradiomycins obtained from a marine-derived actinomycete strain that show induction of apoptosis in the colon tumor cell line HCT-116,24 and the chlorinated bisindole alkaloids (indimicins) obtained from a deep-sea-derived Streptomyces sp. that show antitumor activities in cell levels.25 Halogenated compounds in nature are catalyzed by the relevant halogenases, including haloperoxidases, nonheme Fe(II)-α-ketoglutarate-dependent halogenases, S-adenosyl-L-methionine-dependent halogenases and flavin-dependent halogenases to incorporate with fluorine, chlorine, bromine, and iodine donors.26,27 In our previous study, a group of chlorodepsidones, namely, spiromastixones B–P, were obtained from a deep-sea Spiromastix sp. fungus; these compounds display selective activity against a panel of Gram-positive bacteria, including potent inhibition against methicillin-resistant and vancomycin-resistant pathogenic bacteria.14,28 In addition to known chlorodepsidones, the LC-MS total ion chromatograph of the EtOAc extract of the fermented Spiromastix sp. strain provided the ion peaks for a number of unknown chlorinated compounds. To discover new chlorinated metabolites from the same fungal strain, the minor components from the EtOAc fraction of the solid cultures were subjected to chromatographic separation, resulting in the isolation of four new depsidones (spiromastixones P–S, 1–4), three new isocoumarins (spiromastimelleins A (5) and B (6)), and spiromastibenzothiazole A (7)), together with the known benzothiazole 8,29 of which 1–3 and 5 and 6 were chlorinated (Fig. 1). Herein, the structural elucidation and antibacterial activities of all compounds are described.
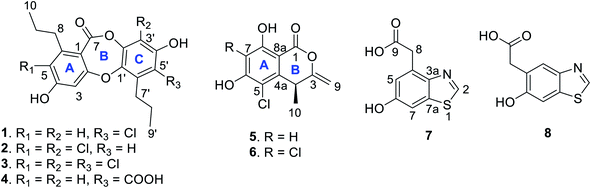 |
| Fig. 1 Chemical structures of 1–8. | |
2. Experimental section
2.1 General procedure
UV spectra were recorded using a Cary 300 spectrometer. IR data were recorded using a Thermo Nicolet Nexus 470 FT-IR spectrometer. A Bruker Avance-400 FT NMR spectrometer was used to measure NMR spectroscopic data, and DMSO-d6 was used as the solvent with the reference peaks at δH 2.50 and δC 39.9 ppm. A Xevo G2 Q-TOF mass spectrometer was used to record the HRESIMS spectra. Semipreparative HPLC was performed using an Alltech instrument coupled with a YMC-packed reversed-phase C18 column (5 μm, 10 mm × 250 mm). Column chromatography (CC) was carried out using silica gel (100–200 and 200–300 mesh), octadecyl silica (ODS, 50 μm, YMC, Japan), and Sephadex LH-20 (18–110 μm, Uppsala, Sweden). TLC analysis was performed using precoated silica gel plates (Merck, Kieselgel 60 F254). All solvents used for chromatography were of analytical grade.
2.2 Fungal material
The fungus Spiromastix sp. MCCC 3A00308 was isolated from South Atlantic Ocean sediment at a depth of 2869 m. The protocols for taxonomy and fermentation were described in the previous study.14
2.3 Isolation and purification
The fermented broth was extracted with EtOAc, and the EtOAc extract was fractionated into ten fractions (FA–FJ) using vacuum liquid chromatography on silica gel, with petroleum ether (PE)–acetone (50
:
1 to 1
:
1) as the eluent. Previously, fractions FF and FH have been chemically examined.14,28 In this study, fraction FI was selected for further examination. FI (186.2 mg) was fractionated on a Sephadex LH-20 column eluted with MeOH to yield 4 (5.6 mg) and 1 (4.5 mg), along with subfractions FI5–FI8. FI5 (32.1 mg) was separated via semipreparative HPLC with MeCN–H2O (13
:
7) as the mobile phase to obtain 2 (2.3 mg) and 3 (3.8 mg). FI6 (56.5 mg) was purified via semipreparative HPLC using MeOH–H2O (7
:
3) as the mobile phase to yield 5 (8.5 mg) and 6 (7.3 mg). FI7 (26.6 mg) was separated via semipreparative HPLC with MeCN–H2O (3
:
17) to yield 7 (4.4 mg) and 8 (3.8 mg).
Spiromastixone P (1): white powder; UV (MeOH) λmax (log
ε) 225 (4.26), 262 (3.86) nm; IR (KBr) νmax 3400, 2934, 1653, 1613, 1454, 1385, 1251 cm−1; 1H and 13C NMR data, see Table 1; HRESIMS m/z 361.0843 [M − H]− (calcd for C19H18ClO5, 361.0843).
Table 1 1H and 13C NMR data of 1–4 in DMSO-d6
Position |
1 |
2 |
3 |
4 |
δC, type |
δH (J in Hz) |
δC, type |
δH (J in Hz) |
δC, type |
δH (J in Hz) |
δC, type |
δH (J in Hz) |
1 |
111.4, C |
|
119.5, C |
|
119.9, C |
|
111.5, C |
|
2 |
163.2, C |
|
160.9, C |
|
160.7, C |
|
162.5, C |
|
3 |
105.1, CH |
6.62, br s |
105.9, CH |
6.85, s |
105.9, CH |
6.88, s |
105.1, CH |
6.59, d (2.2) |
4 |
162.7, C |
|
158.5, C |
|
158.7, C |
|
163.2, C |
|
5 |
115.6, CH |
6.63, br s |
112.7, C |
|
112.6, C |
|
115.5, CH |
6.62, d (2.2) |
6 |
149.7, C |
|
145.5, C |
|
145.8, C |
|
149.6, C |
|
7 |
162.6, C |
|
161.9, C |
|
161.3, C |
|
162.8, C |
|
8 |
35.5, CH2 |
2.67, t (7.5) |
33.0, CH2 |
2.83, t (7.4) |
33.1, CH2 |
2.83, t (7.5) |
35.5, CH2 |
2.68, t (7.5) |
9 |
24.7, CH2 |
1.49, m |
22.7, CH2 |
1.54, m |
22.7, CH2 |
1.54, m |
24.7, CH2 |
1.49, m |
10 |
14.3, CH3 |
0.85, t (7.2) |
14.1, CH3 |
0.86, t (7.4) |
14.1, CH3 |
0.86, t (7.3) |
14.3, CH3 |
0.85, t (7.3) |
1′ |
141.6, C |
|
142.2, C |
|
142.6, C |
|
141.2, C |
|
2′ |
143.1, C |
|
145.5, C |
|
140.4, C |
|
145.3, C |
|
3′ |
105.7, CH |
6.75, s |
109.0, C |
|
111.8, C |
|
105.9, CH |
6.61, s |
4′ |
151.4, C |
|
151.7, C |
|
148.3, C |
|
153.6, C |
|
5′ |
116.9, C |
|
112.9, CH |
6.69, s |
119.2, C |
|
119.7, C |
|
6′ |
134.1, C |
|
133.5, C |
|
132.1, C |
|
134.0, C |
|
7′ |
29.9, CH2 |
2.85, t (7.8) |
31.2, CH2 |
2.67, t (7.7) |
29.9, CH2 |
2.85, t (7.8) |
29.8, CH2 |
2.78, t (7.8) |
8′ |
22.7, CH2 |
1.54, m |
23.6, CH2 |
1.57, m |
22.5, CH2 |
1.56, m |
24.3, CH2 |
1.55, m |
9′ |
14.6, CH3 |
1.04, t (7.3) |
14.3, CH3 |
0.98, t (7.3) |
14.5, CH3 |
1.05, t (7.4) |
14.8, CH3 |
0.99, t (7.3) |
10′ |
|
|
|
|
|
|
169.0, C |
|
4-OH |
|
10.78, s |
|
11.65, br s |
|
11.75, br s |
|
10.72, br s |
4′-OH |
|
10.53, s |
|
10.50, s |
|
10.45, br s |
|
11.93, br s |
Spiromastixone Q (2): white powder; UV (MeOH) λmax (log
ε) 223 (4.32), 266 (3.63) nm; IR (KBr) νmax 3391, 1726, 1595, 1431, 1241 cm−1; 1H and 13C NMR data, see Table 1; HRESIMS m/z 395.0449 [M − H]− (calcd for C19H17Cl2O5, 395.0453).
Spiromastixone R (3): white powder; UV (MeOH) λmax (log
ε) 223 (4.25), 266 (3.71) nm; IR (KBr) νmax 3381, 1729, 1594, 1428, 1240 cm−1; 1H and 13C NMR data, see Table 1; HRESIMS m/z 429.0053 [M − H]− (calcd for C19H16Cl3O5, 429.0063).
Spiromastixone S (4): white powder; IR (KBr) νmax 3259, 1718, 1607, 1451, 1251, 1221 cm−1; 1H and 13C NMR data, see Table 1; HRESIMS m/z 371.1126 [M − H]− (calcd for C20H19O7, 371.1131).
Spiromastimellein A (5): colorless oil; [α]25D + 223 (c 1.07, CH2Cl2); UV (MeOH) λmax 220 (3.15), 269 (2.65), 313 (1.74) nm; ECD (MeOH) λmax (Δε): 244 (+33.09), 264 (+36.11), 315 (−4.47) nm; IR (KBr) νmax 3188, 1692, 1658, 1617, 1469, 1216 cm−1; 1H and 13C NMR data, see Table 2; HRESIMS m/z 239.0118 [M − H]− (calcd for C11H8ClO4, 239.0111).
Table 2 1H and 13C NMR data of 5–8 in DMSO-d6
No. |
5 |
6 |
7 |
8 |
δC |
δH (J in Hz) |
δC |
δH (J in Hz) |
δC |
δH (J in Hz) |
δC |
δH (J in Hz) |
Assignment in the column can be interchanged. |
1 |
164.8, C |
|
165.3, C |
|
|
|
|
|
2 |
|
|
|
|
152.2a, CH |
9.06, s |
152.4a, CH |
9.07, s |
3 |
156.1, C |
|
155.7, C |
|
|
|
|
|
3a |
|
|
|
|
146.5, C |
|
147.0, C |
|
4 |
35.0, CH |
4.08, q (7.0) |
34.8, CH |
4.11, q (7.0) |
130.9, C |
|
125.2, CH |
7.84, s |
4a |
142.8, C |
|
140.3, C |
|
|
|
|
|
5 |
109.6, C |
|
110.9, C |
|
117.3, CH |
6.92, d (2.3) |
123.3, C |
|
6 |
161.3a, C |
|
157.5, C |
|
155.9, C |
|
154.6, C |
|
7 |
102.6, CH |
6.49, s |
108.3, C |
|
105.8, CH |
7.32, d (2.3) |
106.3, CH |
7.46, s |
7a |
|
|
|
|
7.32, d (2.3) |
|
133.6, C |
|
8 |
161.9a, C |
|
157.8, C |
|
37.7, CH2 |
3.98, s |
36.2, CH2 |
3.62, s |
8a |
99.2, C |
|
99.4, C |
|
|
|
|
|
9 |
97.2, CH2 |
4.86, br s |
97.8, CH2 |
4.90, d (1.8) |
172.7, C |
|
173.0, C |
|
4.80, br s |
4.85, d (1.8) |
10 |
21.5, CH3 |
1.34, d (7.0) |
21.5, CH3 |
1.36, d (7.0) |
|
|
|
|
6-OH |
|
11.74, br s |
|
|
9.78, s |
|
|
10.0, s |
8-OH |
|
10.74, s |
|
11.34, s |
|
|
|
|
COOH |
|
|
|
|
12.32, br s |
|
|
12.17, br s |
Spiromastimellein B (6): colorless oil; [α]25D + 165 (c 0.65, CH2Cl2); UV (MeOH) λmax 218 (3.17), 269 (2.45), 313 (1.82) nm; ECD (MeOH) λmax (Δε): 245 (+24.15), 264 (+26.34), 318 (−2.48) nm; IR (KBr) νmax 3419, 2962, 1767, 1710, 1690, 1663, 1461, 1243 cm−1; 1H and 13C NMR data, see Table 2; HRESIMS m/z 272.9718 [M − H]− (calcd for C11H7Cl2O4, 272.9721).
Spiromastibenzothiazole A (7): colorless oil; UV (MeOH) λmax 220 (2.25), 240 (4.15), 273 (3.55) nm; 1H and 13C NMR data, see Table 2; HRESIMS m/z 208.0070 [M − H]− (calcd for C9H6NO3S, 208.0068).
2.4 Antibacterial test
Antibacterial effects against Gram-positive bacteria (Staphylococcus aureus ATCC 25923, Bacillus thuringiensis ATCC 10792, and Bacillus subtilis CMCC 63501) and a Gram-negative bacterium (Escherichia coli ATCC 25922) were detected using a protocol in the literature.28 Chloroamphenicol was used as a positive control.
3. Results and discussion
Spiromastixone P (1) was obtained as a white powder. Its molecular formula was established as C19H19ClO5 on the basis of the HRESIMS data (m/z 361.0843, [M − H]−), indicating ten degrees of unsaturation. The IR absorptions at 3400, 1653, and 1613 cm−1 suggested the presence of hydroxy, carbonyl, and phenyl functionalities. The 1H NMR data showed three aromatic protons, two methyl group triplets, four methylene groups, and two phenol protons (Table 1). Diagnostic 13C NMR and HSQC data revealed a total of 19 carbon resonances, from which 12 aromatic resonances for two phenyl groups, a carbonyl carbon at δC 162.6, four methylene and two methyl carbons were identified. These NMR data were characteristic of a depsidone, and closely resembled those of spiromastixone I,14 a co-isolated analog from the same fungal strain. The 2D NMR data revealed that the partial structure of ring A was identical to that of the known analog, but the absence of an aromatic proton and the presence of an additional quaternary carbon in aromatic ring C were recognized. The HMBC correlations from H-3′ to C-1′ (δC 141.6), C-2′, C-4′(δC 151.4), and C-5′ (δC 116.9), OH-4′ (δH 10.53, s) to C-3′ (δC 105.7), C-4′, and C-5′, and from H2-7′ to C-1′, C-5′, and C-6′ (δC 134.1), clarified that C-5′ was chlorinated. An ether bond connecting rings A and C across C-2 to C-1′ was evident from the NOE correlation between H-3 and H2-7′ (Fig. 2). Thus, 1 was identified as a 5′-chlorinated spiromastixone I.
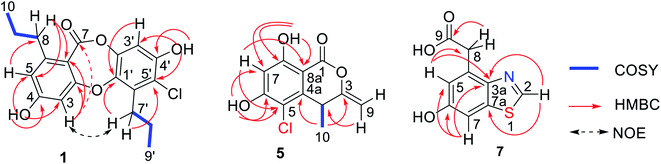 |
| Fig. 2 Key COSY, HMBC and NOE correlations of 1, 5 and 7. | |
Spiromastixone Q (2) had a molecular formula of C19H18Cl2O5 as determined by the HRESIMS data (m/z 395.0449 [M − H]−). A comparison of the NMR data (Table 1) revealed that 2 was an analog of spiromastixone K with the same partial structure of ring A. The difference was found in ring C, where a quaternary carbon was observed at C-3′ (δC 109.0) instead of the methine group of the known analogue. The HMBC correlations from 4′-OH (δH 10.50, s) to C-3′, C-4′ (δC 151.7), and C-5′ (δC 112.9), and from H-5′ (δH 6.69, s) to C-3′, C-1′ (δC 142.2), and C-7′ (δC 31.2) suggested a chlorine substitution at C-3′. Thus, 2 was identified as a 3′-chlorinated spiromastixone K.14
The molecular formula of spiromastixone R (3) was determined as C19H17Cl3O5 from the HRESIMS (m/z 429.0053, [M − H]−) and 13C NMR data. The similar NMR data of 2 and 3, except for the quaternary carbon at C-5′ (δC 119.2) for 3, in association with the molecular composition identified 3 to be a 5′-chlorinated analog of 2. The NOE interaction between H-3 (δH 6.88) and H2-7′ confirmed the location of the ether bond.
The HRESIMS data (m/z 371.1126 [M − H]−) indicated the molecular formula of spiromastixone S (4) to be C20H20O7. Its NMR data resembled those of spiromastixone I, but a carboxylic group (δC 169.0) and the loss of H-5′ were recognized. The HMBC correlations from H-3′ (δH 6.61) to C-1′ (δC 141.2) and C-5′ (δC 119.7) and from H2-7′ (δH 2.78) to C-1′, C-5′, and C-6′ (δC 134.0), along with the NOE interaction between H-3 (δH 6.59) and H2-7′, allowed the assignment of 4 as an analog of spiromastixone I with a carboxylic group at C-5′.
Spiromastimellein A (5) has a molecular formula of C11H9ClO4 as determined from the HRESIMS and NMR data, requiring seven degrees of unsaturation and containing a chlorine atom. The IR absorptions at 3188 and 1658 cm−1 suggested the presence of hydroxy and carbonyl functionalities. The 1H NMR spectrum exhibited resonances including a methyl doublet, an exocyclic methylene group, an sp3 methine, an aromatic singlet, and two D2O-exchangeable protons (Table 2). The 13C NMR spectrum showed 11 carbon resonances, including six aromatic carbons corresponding to a phenyl unit, a carbonyl carbon, two olefinic carbons, an sp3 methine, and a methyl carbon (Table 2). The aromatic proton H-7 (δH 6.49, s) showed HMBC correlations with C-5 (δC 117.3), C-6 (δC 161.3), C-8 (δC 161.9), and C-8a (δC 99.2), which, in conjunction with the NOE relationships of H-7 with the phenol protons OH-6 (δH 11.74, br) and OH-8 (δH 10.74, s), indicated an aromatic ring with OH groups at C-6 and C-8. The long-range W correlation of H-7 with C-1 (δC 164.8) suggested the presence of a carbonyl group at C-8a. The presence of a butene unit was established from the COSY relationship between H-4 (δH 4.08, q) and H3-10 (δH 1.34, d) together with the HMBC correlations between H2-9 (δH 4.86, 4.80)/C-4 (δC 35.0) and H3-10/C-3 (δC 156.1). The linkage of the butane unit to C-4a (δC 142.8) was deduced from the HMBC correlations between H3-10 and C-4a and from H-4 to C-4a, C-5 and C-8a. According to the molecular unsaturation, the one remaining unsaturation was contributed by the formation of a δ-lactone across C-1 and C-3, while a chlorine atom was substituted at C-5. Thus, 5 was determined to be a chlorinated isocoumarin with an exomethylene group at C-3.30 Compound 5 and clearanol C30–32 both possess a δ-lactone ring substituted with a methylene group at the C-3 position and a methyl group at the C-4 position. Since both compounds have similar optical rotations and ECD spectra ([α]25D + 223 and positive Cotton Effects (CEs) at 244 and 264 nm for 5; [α]25D + 215.0 (MeOH) and positive CEs at 237 and 267 nm for clearanol C,30 Fig. 3 and S8-2†), they are both assigned the absolute configuration of S at C-4.
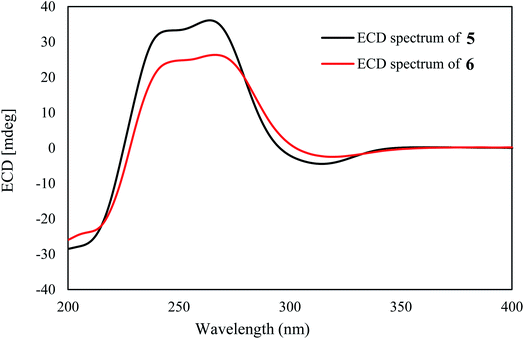 |
| Fig. 3 Experimental ECD spectra of 5 and 6 in MeOH. | |
The molecular formula of spiromastimellein B (6) was determined to be C11H8Cl2O4 based on the HRESIMS and NMR data, containing two chlorine atoms. The NMR data of 6 (Table 2) were closely related to those of 5, except that the aromatic carbon C-7 was substituted. The HMBC correlations of OH-8 (δH 11.34) with the nonprotonated aromatic carbons C-7 (δC 108.3), C-8 (δC 157.8), and C-8a (δC 99.4) supported the conclusion that 6 was a 7-chlorinated analog of 5. The same sign of optical rotation and the similar ECD data (Fig. 3) suggested that both 5 and 6 had the same configuration.
Spiromastimellein C (7) has a molecular formula of C9H7NO3S as determined from the HRESIMS data (m/z 208.0070 [M − H]−), containing seven degrees of unsaturation. The 1H NMR spectrum exhibited meta-coupled aromatic H-5 (δH 6.92, d, J = 2.3 Hz) and H-7 protons (δH 7.32, d, J = 2.3 Hz), an olefinic H-2 proton (δH 9.06, s), methylene protons (δH 3.98, s), and D2O-exchangeable OH-6 (δH 9.78, s) and COOH protons (δH 12.32, br) (Table 2). A tetra-substituted phenyl ring was established from the HMBC correlations from H-5 and H-7 to C-3a (δC 146.5) and from OH-6 to C-5 (δC 117.3), C-6 (δC 155.9), and C-7 (δC 105.8), indicating the presence of a phenolic group at C-6. The presence of an acetic acid unit at C-7 was evident from the HMBC correlations from H2-8 to carboxylic carbon C-9 (δC 172.7) as well as to C-5 and C-3a. The remaining unit containing a nitrogen atom and a sulfur atom was suggested to be a thiazole moiety fused to the phenyl ring, which was supported by the HMBC correlations from H-2 to C-4a and C-8a.
In addition, a known compound identical to M4582 (8)30 was isolated, which was identified as an analog of 7 with an alternative substitution of the acetic acid unit at C-5 on the basis of the NMR data.
Compounds 1–8 were tested for antibacterial activity against the Gram-positive bacterium strains Staphylococcus aureus ATCC 25923, Bacillus thuringiensis ATCC 10792, and Bacillus subtilis CMCC 63501, as well as against Gram-negative bacterium Escherichia coli ATCC 25922. Compounds 1–6 showed significant inhibitory activities against three Gram-positive bacteria with minimum inhibitory concentration (MIC) values ranging from 0.5–32 μg mL−1. However, all compounds exhibited weak activity against E. coli, implying selective inhibition toward Gram-positive bacteria (Table 3). Comparison of the activities revealed that the capabilities of depsidones to produce antibacterial effects was related to the number of chlorine atoms in the backbone. Among them, 3, which was trichlorinated, was the most active. Dichlorinated analogue 2 showed more activity than 1; the latter incorporated one chlorine atom. Compound 4 without chlorine substitution showed weaker activity than 1–3. Similarly, dichlorinated isocoumarin 6 showed more activity than the monochlorinated analogue 5.
Table 3 Antibacterial effects of 1–8
Compound |
MIC (μg mL−1) |
Staphylococcus aureus ATCC 25923 |
Bacillus thuringiensis ATCC 10792 |
Bacillus subtilis CMCC 63501 |
Escherichia coli ATCC 25922 |
CP: chloroamphenicol used as a positive control. |
1 |
8 |
4 |
8 |
>128 |
2 |
4 |
2 |
2 |
>128 |
3 |
1 |
1 |
0.5 |
>128 |
4 |
32 |
16 |
16 |
>128 |
5 |
32 |
32 |
16 |
>128 |
6 |
16 |
4 |
4 |
>128 |
7 |
>128 |
>128 |
>128 |
>128 |
8 |
>128 |
>128 |
>128 |
>128 |
CPa |
1 |
1 |
1 |
4 |
4. Conclusion
In summary, six new chlorinated metabolites were obtained from a deep-sea-derived Spiromastix sp. fungus. These findings enriched the chemical diversity of marine-fungus-derived chlorinated metabolites, and implied that marine-derived fungi are a potential source to discover halometabolites. The antibacterial activities of 3 were comparable to that of the positive control chloroamphenicol, suggesting that 3 was a promising lead for the development of an antibacterial agent. The different scaffolds of 3 and chloroamphenicol implied that 3 may have a distinct mode of action, but this requires further investigation.
Author contributions
SN and DL isolated all compounds; ZS and JH did the bioassay; AF and WL elucidated the structures and edited the article.
Conflicts of interest
There are no conflicts to declare.
Acknowledgements
This work was funded by DY135-B2-05, and the National Natural Science Foundation of China (81991525, 21861142006, 81872793, 81630089, 81803424).
References
- G. W. Gribble, Naturally occurring organohalogen compounds-a comprehensive survey, Prog. Chem. Org. Nat. Prod., 2010, 68, 1–423 Search PubMed.
- G. W. Gribble, Naturally occurring organohalogen compounds-a comprehensive update, Prog. Chem. Org. Nat. Prod., 1996, 91, 9–348 Search PubMed.
- X. Yu, W. He, D. Liu, M. Feng, Y. Fang, B. Wang, L. Feng, Y. Guo and S. Mao, A seco-laurane sesquiterpene and related laurane derivatives from the red alga Laurencia okamurai Yamada, Phytochemistry, 2014, 103, 162–170 CrossRef CAS PubMed.
- L. Tian, Y. Feng, Y. Shimizu, T. A. Pfeifer, C. Wellington, J. N. A. Hooper and R. J. Quinn, ApoE secretion modulating bromotyrosine derivative from the Australian marine sponge Callyspongia sp., Bioorg. Med. Chem. Lett., 2014, 24, 3537–3540 CrossRef CAS PubMed.
- T. Kusama, N. Tanaka, K. Sakai, T. Gonoi, J. Fromont, Y. Kashiwada and J. Kobayashi, Agelamadins A and B, dimeric bromopyrrole alkaloids from a marine sponge Agelas sp., Org. Lett., 2014, 16, 3916–3918 CrossRef CAS PubMed.
- L. Tian, Y. Feng, Y. Shimizu, T. Pfeifer, C. Wellington, J. N. A. Hooper and R. J. Quinn, Aplysinellamides A–C, bromotyrosine-derived metabolites from an Australian Aplysinella sp. marine sponge, J. Nat. Prod., 2014, 77, 1210–1214 CrossRef CAS PubMed.
- Y. Lee, W. Wang, H. Kim, A. G. Giri, D. H. Won, D. Hahn, K. R. Baek, J. Lee, I. Yang, H. Choi, J. Nam and H. Kang, Phorbaketals L–N, cytotoxic sesterterpenoids isolated from the marine sponge of the genus Phorbas, Bioorg. Med. Chem. Lett., 2014, 24, 4095–4098 CrossRef CAS PubMed.
- Y. Su, C. Cheng, W. Chen, Y. Chang, Y. Chen, T. Hwang, Z. Wen, W. Wang, L. Fang, J. Chen, Y. Wu, J. Sheu and P. Sung, Briarenolide J, the first 12-chlorobriarane diterpenoid from an octocoral Briareum sp. (Briareidae), Tetrahedron Lett., 2014, 55, 6065–6067 CrossRef CAS.
- H. Lei, J.-F. Sun, Z. Han, X.-F. Zhou, B. Yang and Y. Liu, Fragilisinins A–L, new briarane-type diterpenoids from gorgonian Junceella fragilis, RSC Adv., 2014, 4, 5261–5271 RSC.
- D. Smith, M. M. K. Kumar, H. Ramana and D. V. Rao, Rubrolide R: A new furanone metabolite from the ascidian Synoicum of the Indian Ocean, Nat. Prod. Res., 2014, 28, 12–17 CrossRef PubMed.
- M. Tadesse, J. Svenson, K. Sepčić, L. Trembleau, M. Engqvist, J. H. Andersen, M. Jaspars, K. Stensvåg and T. Haug, Isolation and synthesis of pulmonarins A and B, acetylcholinesterase inhibitors from the colonial ascidian Synoicum pulmonaria, J. Nat. Prod., 2014, 77, 364–369 CrossRef CAS PubMed.
- W. Zhang, C. Shao, M. Chen, Q. Liu and C. Wang, Brominated resorcylic acid lactones from the marine-derived fungus Cochliobolus lunatus induced by histone deacetylase inhibitors, Tetrahedron Lett., 2014, 55, 4888–4891 CrossRef CAS.
- T. El-Elimat, H. A. Raja, C. S. Day, W. Chen, S. M. Swanson and N. H. Oberlies, Greensporones: Resorcylic acid lactones from an aquatic Halenospora sp., J. Nat. Prod., 2014, 77, 2088–2098 CrossRef CAS PubMed.
- S. Niu, D. Liu, X. Hu, P. Proksch, Z. Shao and W. Lin, Spiromastixones A–O, antibacterial chlorodepsidones from a deep-sea-derived Spiromastix sp. fungus, J. Nat. Prod., 2014, 77, 1021–1030 CrossRef CAS PubMed.
- L. Farnaes, N. G. Coufal, C. A. Kauffman, A. L. Rheingold, A. G. DiPasquale, P. R. Jensen and W. Fenical, Napyradiomycin derivatives, produced by a marine-derived actinomycete, illustrate cytotoxicity by induction of apoptosis, J. Nat. Prod., 2014, 77, 15–21 CrossRef CAS PubMed.
- W. Zhang, L. Ma, S. Li, Z. Liu, Y. Chen, H. Zhang, G. Zhang, Q. Zhang, X. Tian, C. Yuan, S. Zhang, W. Zhang and C. Zhang, Indimicins A–E, bisindole alkaloids from the deep-sea-derived Streptomyces sp. SCSIO 03032, J. Nat. Prod., 2014, 77, 1887–1892 CrossRef CAS PubMed.
- M. Vegman and S. Carmeli, Three aeruginosins and a microviridin from a bloom assembly of Microcystis spp. collected from a fishpond near Kibbutz Lehavot HaBashan, Israel, Tetrahedron, 2014, 70, 6817–6824 CrossRef CAS.
- S. Luo, H. Kang, A. Krunic, G. E. Chlipala, G. Cai, W. Chen, S. G. Franzblau, S. M. Swanson and J. Orjala, Carbamidocyclophanes F and G with anti-Mycobacterium tuberculosis activity from the cultured freshwater cyanobacterium Nostoc sp., Tetrahedron Lett., 2014, 55, 686–689 CrossRef CAS PubMed.
- M. E. F. Hegazy, A. Y. Moustfa, A. E. H. H. Mohamed, M. A. Alhammady, S. E. I. Elbehairi, S. Ohta and P. W. Pare, New cytotoxic halogenated sesquiterpenes from the Egyptian sea hare, Aplysia oculifera, Tetrahedron Lett., 2014, 55, 1711–1714 CrossRef CAS.
- H. M. Feder Jr, Chloramphenicol: what we have learned in the last decade, South. Med. J., 1986, 79, 1129–1134 CrossRef CAS.
- R. Álvarez, L. E. López Cortés, J. Molina, J. M. Cisneros and J. Pachón, Optimizing the clinical use of vancomycin, Antimicrob. Agents Chemother., 2016, 60, 2601–2609 CrossRef PubMed.
- J. A. Bush, B. H. Long, J. J. Catino, W. T. Bradner and K. Tomita, Production and biological activity of rebeccamycin, a novel antitumor agent, J. Antibiot., 1987, 40, 668–678 CrossRef CAS PubMed.
- W. Zhang, C. Shao, M. Chen, Q. Liu and C. Wang, Brominated resorcylic acid lactones from the marine-derived fungus Cochliobolus lunatus induced by histone deacetylase inhibitors, Tetrahedron Lett., 2014, 55, 4888–4891 CrossRef CAS.
- L. Farnaes, N. G. Coufal, C. A. Kauffman, A. L. Rheingold, A. G. DiPasquale, P. R. Jensen and W. Fenical, Napyradiomycin derivatives, produced by a marine-derived actinomycete, illustrate cytotoxicity by induction of apoptosis, J. Nat. Prod., 2014, 77, 15–21 CrossRef CAS PubMed.
- K. A. McArthur, S. S. Mitchell, G. Tsueng, A. Rheingold, D. J. White, J. Grodberg, K. S. Lam and B. C. M. Potts, Lynamicins A–E, chlorinated bisindole pyrrole antibiotics from a novel marine actinomycete, J. Nat. Prod., 2008, 71, 1732–1737 CrossRef CAS PubMed.
- J. Zeng and J. Zhan, Chlorinated natural products and related halogenases, Isr. J. Chem., 2019, 59, 387–402 CrossRef CAS.
- A. S. Eustáquio, F. Pojer, J. P. Noel and B. S. Moore, Discovery and characterization of a marine bacterial SAM-dependent chlorinase, Nat. Chem. Biol., 2008, 4, 69–74 CrossRef PubMed.
- S. Niu, D. Liu, P. Proksch, Z. Shao and W. Lin, New polyphenols from a deep sea Spiromastix sp. fungus, and their antibacterial activities, Mar. Drugs, 2015, 13, 2526–2540 CrossRef CAS PubMed.
- M. D. Parenti, S. Pacchioni, A. M. Ferrari and G. Rastelli, Three-dimensional quantitative structure–activity relationship analysis of a set of Plasmodium falciparum dihydrofolate reductase inhibitors using a pharmacophore generation approach, J. Med. Chem., 2004, 47, 4258–4267 CrossRef CAS PubMed.
- W. C. Tayone, S. Kanamaru, M. Honma, K. Tanaka, T. Nehira and M. Hashimoto, Absolute stereochemistry of novel isochromanone derivatives from Leptosphaeria sp. KTC 727, Biosci., Biotechnol., Biochem., 2011, 75, 2390–2393 CrossRef CAS PubMed.
- T. El-Elimat, H. A. Raja, M. Figueroa, J. O. Falkinham and N. H. Oberlies, Isochromenones, isobenzofuranone, and tetrahydronaphthalenes produced by Paraphoma radicina, a fungus isolated from a freshwater habitat, Phytochemistry, 2014, 104, 114–120 CrossRef CAS PubMed.
- J. Tang, W. Wang, A. Li, B. Yan, R. Chen, X. Li, X. Du, H. Sun and J. Pu, Polyketides from the endophytic fungus Phomopsis sp. sh917 by using the one strain/many compounds strategy, Tetrahedron, 2017, 73, 3577–3584 CrossRef CAS.
Footnote |
† Electronic supplementary information (ESI) available. See DOI: 10.1039/d1ra05736g |
|
This journal is © The Royal Society of Chemistry 2021 |
Click here to see how this site uses Cookies. View our privacy policy here.