DOI:
10.1039/D1RA05494E
(Paper)
RSC Adv., 2021,
11, 36230-36236
MOF-Zn-NHC as an efficient N-heterocyclic carbene catalyst for aerobic oxidation of aldehydes to their corresponding carboxylic acids via a cooperative geminal anomeric based oxidation†
Received
19th July 2021
, Accepted 17th October 2021
First published on 10th November 2021
Abstract
As an efficient heterogenous N-heterocyclic carbene (NHC) catalyst, MOF-Zn-NHC was used in the aerobic oxidation of aryl aldehydes to their corresponding carbocyclic acids via an anomeric based oxidation. Features such as mild reaction conditions and no need for a co-catalyst or oxidative reagent can be considered as the major advantages of the presented method in this study.
Introduction
Nowadays, N-heterocyclic carbenes (NHCs), with unique features such as strong σ-donor ability and electronic tenability, are considered as new complexes by the chemical community.1–4 N-Heterocyclic carbene (NHC)-complexes have exclusive properties, which can be utilized to conduct exceptional chemical reactions.5–8 The NHC-complexes have been used as catalysts in the synthesis of pharmaceutical and chemical compounds; they can also be used as stable ligands in the synthesis of various metal complexes with diverse biological properties.9–12 In situ generation of carbene, in the course of the reaction, has allowed researchers to use NHC-complexes in a wide range of chemical processes. Also, metal–organic frameworks (MOFs) with N-heterocyclic carbene pillars, as a new class of nanoporous materials, have been used as multi-purpose materials.13,14
In the last few years, there has been an increasing penchant for researching the oxidation of organic compounds. In this regard, choosing a convenient oxidation method is considered to be the main objective in this research subject.8,12,15–39 Previously, many of the presented oxidation strategies were focused on the conversion of aryl aldehyde to their corresponding carbocyclic acids (when O2 or oxidative reagent was used) (Scheme 1).9,40–44 Therefore, these approaches suffer from significant drawbacks, mainly: (i) using many catalysts and co-catalysts, (ii) longer reaction times, (iii) using toxic solvent, catalyst and oxidative reagent and (iv) using oxidizing agents. Therefore, mild oxidation methodologies need to be developed for more efficient operation strategy.
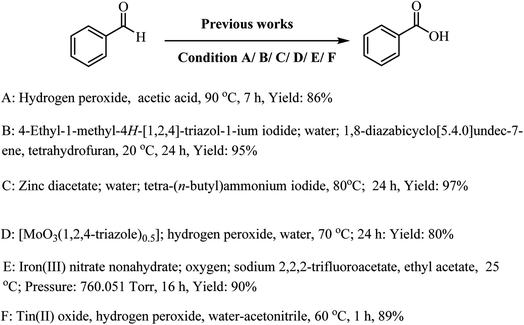 |
| Scheme 1 Previously reported methods of oxidation of aldehyde to their corresponding carboxylic acid. | |
In the course of organic reactions, the anomeric effect can support the conversion of susceptible molecules and/or intermediates to the desired products.45 For example, we recently proposed adding hydroxide (OH−) to the carbonyl group of aldehydes in the Cannizzaro reaction, which did not any have α-hydrogen (Scheme 2), sharing the lone pairs electrons from both geminal oxygen atoms at the tetrahedral carbon into the anti-bonding orbital of C–H bond
and weakening it. The resulting labile hydride acts as a powerful nucleophile that attaches to the second molecule of aldehyde. Finally, the aforementioned reaction produced an equal amount of acid and alcohol.46 We have recently introduced the term “anomeric based oxidation” or ABO for these types of reactions.47–49 In the discussion section of the presented work, the anomeric based oxidation is considered for proposing a rational mechanism. To the best of our knowledge, carbon is the core element and one of the major components of organic chemistry. However, oxygen plays the major role of stereoelectronic control in organic chemistry, since C–O bond has both a strong donor (lone pair) and a strong acceptor
. This unique property of oxygen provides a slew of opportunities to influence the organic functional transformations. Also, we recently wrote a comprehensive review article about the stereoelectronic power of oxygen in controlling the chemical reactivity.50
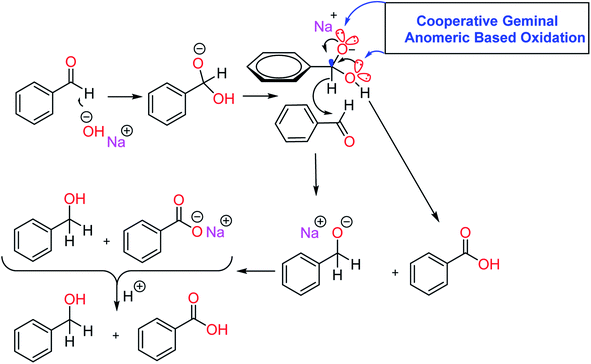 |
| Scheme 2 Anomeric based oxidation leads to oxidation–reduction in the mechanism of Cannizzaro reaction.46 | |
As for the next step in our investigation into the development of the chemoselective oxidation of alcohols,51–53 heterocyclic carbene complexes54 and catalytic application of metal organic frameworks,55–59 we aim to use MOF-Zn-NHC as an efficient heterogenous N-heterocyclic carbene (NHC) catalysts for aerobic oxidation of aryl aldehydes into their corresponding carbocyclic acids under mild and green conditions (Schemes 3 and 4). The hydrolysis of aryl nitriles was occurred under the same conditions.
 |
| Scheme 3 The conversion of aldehydes to their corresponding carboxylic acids in the presence of MOF-Zn-NHC. | |
 |
| Scheme 4 The conversion of isophthalaldehyde to the isophthalic acid (2p) in the presence of MOF-Zn-NHC. | |
The described catalyst converts both aldehyde groups of isophthalaldehyde to their carboxylic acid groups (Scheme 4).
Experimental
General procedure for the preparation of MOF-Zn-NHC
MOF-Zn-NHC was synthesized according to the previously reported experimental procedure.60 A mixture of 1,3-bis(4-carboxyphenyl)-1H-imidazol-3-ium chloride (1 mmol, 0.344 g) and zinc nitrate hexahydrate (4 mmol, 1.19 g) was stirred in 20 mL of dimethyl formamide (DMF) for 10 minutes. The resulting solution was heated in a Teflon-lined autoclave at 140 °C for 24 h. After the reaction mixture was cooled down to room temperature, a cream solid was collected and washed with DMF. Finally, the desired product was dried at 150 °C in a vacuum oven (Scheme S1†).
General procedure for the conversion of aldehydes to the corresponding carboxylic acids
A mixture of aldehyde (2 mmol), triethylamine (10 mol%, 0.01 g) and MOF-Zn-NHC (15 mg) were stirred in a 25 mL round-bottomed flask under refluxing water. Moreover, thin-layer chromatography (TLC) technique was used to assess reaction progress. After the reaction was completed, the catalyst was removed from the reaction using a centrifugation at 1000 rpm. Then, by using hydrochloric acid (0.5 N), the pH of the reaction mixture was reduced to 4. In the next step, ethyl acetate (5 mL) was added the reaction mixture and then, the product was extracted using a separator funnel. Finally, the solvent of organic layer was evaporated and its residue was purified using the flash chromatography technique (n-hexane-ethyl acetate: 10/1) (Scheme 3).
Typical procedure for the conversion of isophthalaldehyde to the isophthalic acid (2p)
According to the abovementioned oxidation method, a mixture of isophthalaldehyde (1 mmol), triethylamine (10 mol%, 0.01 g) and MOF-Zn-NHC (15 mg) were stirred in a 25 mL round-bottomed flask, under refluxing water. Moreover, thin-layer chromatography (TLC) technique was used to assess the reaction progress. After the reaction was completed, the catalyst was removed from the reaction using a centrifugation at 1000 rpm. Finally, the solvent of organic layer was evaporated and its residue was purified using the flash chromatography technique (n-hexane-ethyl acetate 10/1) (Scheme 4 and Table 2, product 2p).
4-Methylbenzoic acid (2a). White solid; Mp: 180–183 °C; IR (KBr): ν (cm−1) = 3071, 2975, 2830, 2652, 2545, 1678, 1611. 1H NMR (600 MHz, DMSO-d6) δppm: 12.81 (s, 1H), 7.84 (d, J = 8.0 Hz, 2H), 7.30 (d, J = 8.0 Hz, 2H), 2.37 (s, 3H). 13C NMR (100 MHz, CDCl3) δppm: 171.0, 163.5, 131.9, 121.1, 113.3, 55.0.
4-Methoxybenzoic acid (2b). White solid; Mp: 185 °C; IR (KBr): ν (cm−1) = 2984, 236, 1686, 1603, 1262. 1H NMR (400 MHz, CDCl3) δppm: 8.10 (d, J = 9.0 Hz, 2H), 6.98 (d, J = 9.0 Hz, 2H), 3.91 (s, 3H). 13C NMR (100 MHz, CDCl3) δppm: 171.0, 163.5, 131.9, 121.1, 113.3, 55.0.
4-Nitrobenzoic acid (2c). White solid; Mp: 185 °C; IR (KBr): ν (cm−1) = 3064, 2894, 2859, 2745, 2439, 1681, 1602. (400 MHz, CDCl3) δppm: 8.24 (d, J = 8.9 Hz, 1H), 8.08 (d, J = 8.9 Hz, 1H). 13C NMR (100 MHz, CDCl3) δppm: 170.6, 151.7, 131.5, 120.7, 120.6.
3-Methylbenzoic acid (2d). White solid; Mp: 260–261 °C; IR (KBr): ν (cm−1) = 3087, 3006, 2823, 2663, 1691, 1611, 1280. 1H NMR (400 MHz, DMSO-d6) δppm: 12.91 (s, 1H), 7.77 (s, 1H), 7.75 (d, J = 7.6 Hz, 1H), 7.43 (d, J = 7.6 Hz, 1H), 7.38 (t, J = 7.6 Hz, 1H), 2.36 (s, 3H).
4-Chlorobenzoic acid (2e). White solid; Mp: 238–242 °C; IR (KBr): ν (cm−1) = 2984, 2839, 2674, 2562, 1682, 1592. 1H NMR (400 MHz, CDCl3) δppm: 8.07 (d, J = 8.5 Hz, 2H), 7.48 (d, J = 8.5 Hz, 2H). 13C NMR (100 MHz, CDCl3) δppm: 169.1, 149.0, 136.2, 131.1, 128.4.
4-Hydroxybenzoic acid (2h). White solid; Mp: 214–216 °C; IR (KBr): ν (cm−1) = 2893, 2497, 1655, 1604, 1574, 1271. 1H NMR (400 MHz, CDCl3) δppm: 13.20 (s, 1H), 10.22 (s, 1H), 8.08 (d, J = 9.0 Hz, 2H), 6.96 (d, J = 9.0 Hz, 2H). 13C NMR (100 MHz, CDCl3) δppm: 170.6, 163.1, 131.5, 120.7, 112.9.
Cinnamic acid (2i). White solid; Mp: 130 °C; IR (KBr): ν (cm−1) = 3070, 3027, 2833, 2592, 1682, 1329. 1H NMR (400 MHz, DMSO-d6) δppm: 12.46 (s, 1H), 7.70 (d, J = 3.6 Hz, 1H), 7.68 (d, J = 2.2 Hz, 1H), 7.61 (d, J = 16.0 Hz, 1H), 7.43–7.40 (m, 3H), 6.55 (d, J = 16.0 Hz, 1H).
2-Chlorobenzoic acid (2j). White solid; Mp: 143–144 °C; IR (KBr): ν (cm−1) = 2995, 2821, 2651, 1691, 1591, 1256. 1H NMR (400 MHz, DMSO-d6) δppm: 13.42 (s, 1H), 7.79 (d, J = 7.2 Hz, 1H), 7.57–7.51 (m, 2H), 7.46–7.40 (m, 1H).
4-Fluorobenzoic acid (2k). White solid; Mp: 185–186 °C; IR (KBr): ν (cm−1) = 2991, 2836, 2678, 2556, 1682, 1529. 1H NMR (400 MHz, DMSO-d6) δppm: 13.08 (s, 1H), 8.02 (d, J = 5.6 Hz, 1H), 7.99 (d, J = 5.6 Hz, 1H), 7.34–7.27 (m, 2H).
Isophthalic acid (2p). White solid; Mp: >300 °C; IR (KBr): ν (cm−1) = 3103, 3069, 2814, 1682, 1283. 1H NMR (400 MHz, DMSO-d6) δppm: 13.29 (s, 2H), 8.49 (t, J = 1.7 Hz, 1H), 8.17 (dd, J = 7.8, 1.8 Hz, 2H), 7.64 (t, J = 7.8 Hz, 1H).
Result and discussion
Since the chemoselective and aerobic oxidation of functional groups is a basic organic reaction, we decided to study the aerobic oxidation of aldehydes. Based on our previous experience and our knowledge of the catalytic applications of N-heterocyclic carbene complexes54 and metal–organic frameworks55 and for the purpose of aerobic oxidation of aryl aldehydes to their corresponding carbocyclic acids, we decided to use MOF-Zn-NHC; this catalyst has both of them in a single structure (Scheme 3). Although the aerobic oxidation of aryl aldehydes using N-heterocyclic carbenes under different reaction conditions,12,18–20,54 has been reported, no recorded report was found about using metal–organic frameworks with N-heterocyclic carbene pillars for this purpose. At the first step, the desired catalyst MOF-Zn-NHC was synthesized and characterized according to a previously reported procedure.60
The FT-IR spectrum of 1,3-bis(4-carboxyphenyl)-1H-imidazol-3-ium chloride as ligand and MOF-Zn-NHC are illustrated in Fig. 1. The absorption bands at 480 cm−1 are linked to the stretching vibrational modes of Zn–O groups in N-heterocyclic carbene. The absorption band at 1708 cm−1 is related to the C
O stretching in ligand. Also, a broad peak is evident at about 2600–3500 cm−1, which is related to OH of CO2H groups in ligand. Finally, the differences between ligand and MOF-Zn-NHC in the FT-IR spectrum confirms the synthesis of N-heterocyclic carbene (NHC). The FT-IR spectrum data seems to be in close agreement with data reported in the literature.60
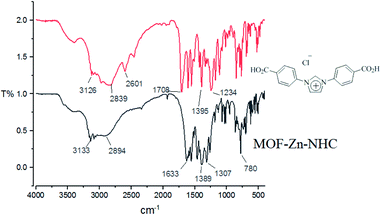 |
| Fig. 1 The FT-IR spectrum of MOF-Zn-NHC and 1,3-bis(4-carboxyphenyl)-1H-imidazol-3-ium chloride as ligand. | |
The XRD diagram for the MOF-Zn-NHC as N-heterocyclic carbene (NHC) in the domain of 2θ = 10–50° is shown in Fig. S1.† The XRD pattern of MOF-Zn-NHC as N-heterocyclic carbene (NHC) and simulated MOF-Zn-NHC are compared in Fig. S1.†60
After the synthesis and characterization of MOF-Zn-NHC, in order to show the catalytic efficiency of MOF-Zn-NHC in the aerobic oxidation of various aldehyde to their carboxylic acids, it was used in aforementioned reactions. In this work, the reaction of 4-methoxybenzaldehyde (2 mmol, 0.272 g) in the presence of MOF-Zn-NHC (15 mg) was selected as a model reaction and tested at different temperatures, bases and in various solvents such as CH2Cl2, DMF, EtOH, CH3CN (5 mL), compared to solvent-free conditions (Table 1). The results of Table 1 indicates that reflux and 15 mg of NHC were the best conditions, in which the optimal efficiency was achieved (Table 1, entry 6). Also, increasing the catalytic amount had no effect on the efficiency (Table 1, entry 7). Moreover, the reaction recorded lower efficiency under lower temperature conditions and lesser amount of the catalyst. This conversion did not occur in catalyst-free conditions (Table 1, entry 9). Also, no improvement was detected in the yield of conversion reaction when using different bases (co-catalyst) (Table 1, entries 11–14). Therefore, the best reaction condition was determined to be 15 mg MOF-Zn-NHC in refluxing H2O for the aerobic oxidation of the various aldehyde to their corresponding carboxylic acids (Table 1, entry 5). The higher efficiency observed in the water solvent is due to the formation of more hydrogen bonds compared to other solvents. This phenomenon causes the raw materials to be closer to each other, which in turn leads to more interaction between the materials and therefore, increases the efficiency.61
Table 1 The effect of different amounts of catalysts, temperature and solvent (5 mL) in the oxidation of aldehydes

|
Entry |
Solvent |
Temp. (°C) |
Base (mol%) |
Catalyst (mg) |
Time (h) |
Yielda (%) |
Isolated yield. |
1 |
H2O |
r.t. |
Et3N (10) |
5 |
12 |
Trace |
2 |
H2O |
50 |
Et3N (10) |
5 |
7 |
45 |
3 |
H2O |
80 |
Et3N (10) |
5 |
5 |
50 |
4 |
H2O |
Reflux |
Et3N (10) |
5 |
3 |
60 |
5 |
H2O |
Reflux |
Et3N (10) |
10 |
2 |
75 |
6 |
H2O |
Reflux |
Et3N (10) |
15 |
2 |
87 |
7 |
H2O |
Reflux |
Et3N (10) |
20 |
6 |
87 |
8 |
H2O |
Reflux |
Et3N (15) |
10 |
2 |
87 |
9 |
H2O |
Reflux |
Et3N (10) |
— |
12 |
— |
10 |
H2O |
Reflux |
— |
10 |
12 |
Trace |
11 |
H2O |
Reflux |
NaOH (10) |
10 |
6 |
45 |
12 |
H2O |
Reflux |
KOH (10) |
10 |
5 |
55 |
13 |
H2O |
Reflux |
Pyridine (10) |
10 |
10 |
45 |
14 |
H2O |
Reflux |
K2CO3 (10) |
10 |
6 |
40 |
15 |
CH2Cl2 |
Reflux |
Et3N (10) |
10 |
10 |
Trace |
16 |
DMF |
90 |
Et3N (10) |
10 |
12 |
— |
17 |
EtOH |
Reflux |
Et3N (10) |
10 |
12 |
— |
18 |
CH3CN |
Reflux |
Et3N (10) |
10 |
12 |
— |
19 |
— |
100 |
Et3N (10) |
10 |
6 |
— |
After optimizing the reaction condition, various aryl aldehydes containing the electron-releasing substituents, the electron-withdrawing substituents, the heterocycle and the halogens on the aromatic ring were reacted with MOF-Zn-NHC to give the desired carboxylic acid compounds. All the products were prepared in high yields and in very short reaction times (Table 2).
Table 2 The catalytic aerobic oxidation of aryl aldehyde to their corresponding carboxylic acids using MOF-Zn-NHC
Isolated yield. |
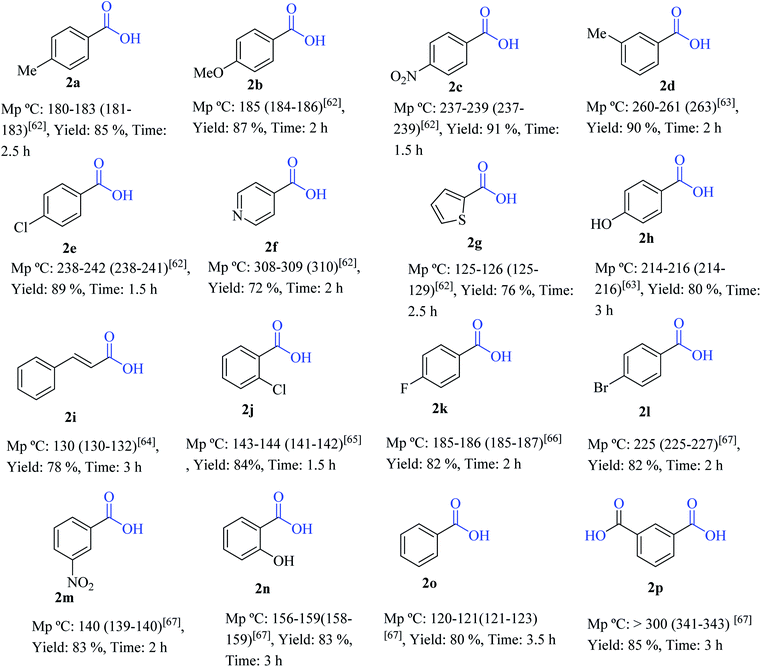 |
The proposed mechanism for the aerobic oxidation of an aryl aldehyde to its corresponding carboxylic acid is shown in Scheme 5. N-Heterocyclic carbene (I) was generated in situ by removing hydrogen with triethylamine (base) from imidazole salt, which is a pillar of MOFs. The generated N-heterocyclic carbene I reacts with the aldehyde to afford intermediate II. Then, intermediate II would give intermediate III via a tautomerization process. Intermediate III reacts with a molecule of oxygen to afford intermediates IV and V respectively. In the latter step, intermediate V is fragmented to an N-heterocyclic carbene I and an anion of carboxylate via a cooperative geminal anomeric based oxidation mechanism. As mentioned in the introduction section of the article, sharing the lone pairs electrons from both geminal oxygen atoms of tetrahedral carbon at the intermediate V into the anti-bonding orbital of C–C bond
lead to the C–C bond cleavage. We think that a cooperative geminal anomeric effect is supporting the C–C bond cleavage at the intermediate V. Finally, this reaction produced an equal amount of carboxylate and N-heterocyclic carbene I.46
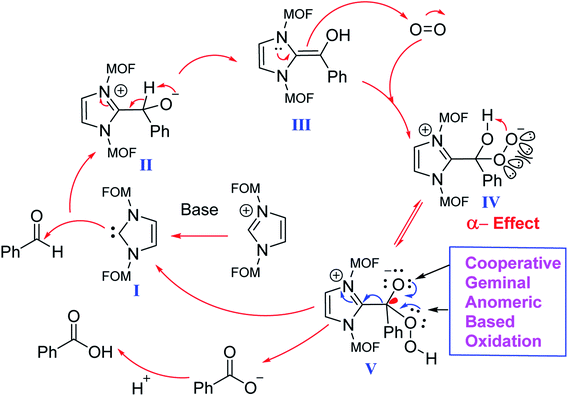 |
| Scheme 5 The proposed mechanism for the aerobic oxidation of an aryl aldehyde to its corresponding carboxylic acid using MOF-Zn-NHC. | |
According to the illustrated results in the Fig. 2, MOF-Zn-NHC can be separated by centrifugation and reused without significantly reducing its catalytic activity. For this purpose, the recyclability of the catalyst was tested on the reaction of 4-methoxy benzaldehyde (2 mmol, 0.268 g) as a model reaction under the aforementioned optimized reaction conditions. Therefore, MOF-Zn-NHC can be reused up to five runs without any noticeable changes in the catalytic activity.
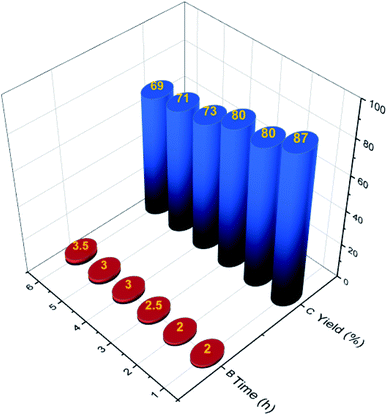 |
| Fig. 2 The recyclability of MOF-Zn-NHC for the synthesis of carboxylic acid compounds. | |
Conclusion
In this work, MOF-Zn-NHC was used as an efficient heterogeneous catalyst for the aerobic oxidation of aryl aldehydes to their corresponding carboxylic acids under mild conditions. Similar to the Cannizzaro reaction, a cooperative geminal anomeric effect was proposed for the latter step of the aerobic oxidation mechanism. Mild reaction conditions, good to excellent yields, and no need for a co-catalyst or oxidizing reagent can be considered as the major advantages of the described methodology.
Conflicts of interest
The authors declare no competing financial and non-financial interests.
Acknowledgements
We would like to thank Bu-Ali Sina University and Iran National Science Foundation (INSF) (Grant Number: 98001912) for their financial support.
References
- S. T. Liddle, I. S. Edworthy and P. L. Arnold, Chem. Soc. Rev., 2007, 36, 1732–1744 RSC.
- P. L. Arnold and I. J. Casely, Chem. Rev., 2009, 109, 3599–3611 CrossRef CAS PubMed.
- H. Song, Y. Kim, J. Park, K. Kim and E. Lee, Synlett, 2016, 27, 477–485 CAS.
- M. A. Land and J. A. Clyburne, Synlett, 2016, 27, 2416–2424 CrossRef CAS.
- A. Grossmann and D. Enders, Angew. Chem., Int. Ed., 2012, 51, 314–325 CrossRef CAS PubMed.
- J. Mahatthananchai and J. W. Bode, Acc. Chem. Res., 2014, 47, 696–707 CrossRef CAS PubMed.
- D. P. Curran, A. Solovyev, M. Makhlouf Brahmi, L. Fensterbank, M. Malacria and E. Lacôte, Angew. Chem., Int. Ed., 2011, 50, 10294–10317 CrossRef CAS PubMed.
- X. Jiang, J. Liu and S. Ma, Org. Process Res. Dev., 2019, 23, 825–835 CrossRef CAS.
- E. G. Delany, C.-L. Fagan, S. Gundala, K. Zeitler and S. J. Connon, Chem. Commun., 2013, 49, 6513–6515 RSC.
- M. He, G. J. Uc and J. W. Bode, J. Am. Chem. Soc., 2006, 128, 15088–15089 CrossRef CAS PubMed.
- J. L. Moore and T. Rovis, Top. Curr. Chem., 2010, 118–144 CrossRef PubMed.
- A. K. Khatana, V. Singh, M. K. Gupta and B. Tiwari, Synthesis, 2018, 50, 4290–4294 CrossRef CAS.
- C. I. Ezugwu, N. A. Kabir, M. Yusubov and F. Verpoort, Coord. Chem. Rev., 2016, 307, 188–210 CrossRef CAS.
- R. S. Crees, M. L. Cole, L. R. Hanton and C. J. Sumby, Inorg. Chem., 2010, 49, 1712–1719 CrossRef CAS PubMed.
- E. E. Finney, K. A. Ogawa and A. J. Boydston, J. Am. Chem. Soc., 2012, 134, 12374–12377 CrossRef CAS PubMed.
- P.-F. Dai, J.-P. Qu and Y.-B. Kang, Org. Lett., 2019, 21, 1393–1396 CrossRef CAS PubMed.
- L. Vanoye, M. Abdelaal, K. Grundhauser, B. Guicheret, P. Fongarland, C. De Bellefon and A. Favre-Réguillon, Org. Lett., 2019, 21, 10134–10138 CrossRef CAS PubMed.
- M. Ji, S. Lim and H.-Y. Jang, RSC Adv., 2014, 4, 28225–28228 RSC.
- S.-M. Kim, Y.-S. Kim and J.-W. Yang, Bull. Korean Chem. Soc., 2011, 32, 2529–2530 CrossRef.
- S. De Sarkar, S. Grimme and A. Studer, J. Am. Chem. Soc., 2010, 132, 1190–1191 CrossRef CAS PubMed.
- Y. Min, W. Chaolong, Z. Li, Z. Li and Y. Xiaoquan, Lett. Org. Chem., 2021, 18, 167–175 CrossRef.
- I. E. Amrani and A. Atlamsani, Mediterr. J. Chem., 2019, 8, 380–389 CrossRef.
- L. Qi, T. Wang, Y. Wei and H. Tian, Eur. J. Org. Chem., 2018, 2018, 6557–6565 CrossRef CAS.
- X. Yan, Y.-H. Lai and R. N. Zare, Chem. Sci., 2018, 9, 5207–5211 RSC.
- X. Jiang, Y. Zhai, J. Chen, Y. Han, Z. Yang and S. Ma, Chin. J. Chem., 2018, 36, 15–19 CrossRef CAS.
- L. Vanoye, M. Pablos, N. Smith, C. de Bellefon and A. Favre-Réguillon, RSC Adv., 2014, 4, 57159–57163 RSC.
- L. Vanoye, A. Aloui, M. Pablos, R. Philippe, A. Percheron, A. Favre-Réguillon and C. de Bellefon, Org. Lett., 2013, 15, 5978–5981 CrossRef CAS PubMed.
- N. Iqbal, S. Choi, Y. You and E. J. Cho, Tetrahedron Lett., 2013, 54, 6222–6225 CrossRef CAS.
- L. Vanoye, A. Favre-Réguillon, A. Aloui, R. Philippe and C. de Bellefon, RSC Adv., 2013, 3, 18931–18937 RSC.
- C. Marsden, E. Taarning, D. Hansen, L. Johansen, S. K. Klitgaard, K. Egeblad and C. H. Christensen, Green Chem., 2008, 10, 168–170 RSC.
- P. Fristrup, L. B. Johansen and C. H. Christensen, Chem. Commun., 2008, 24, 2750–2752 RSC.
- H.-B. Ji, D.-G. He, J. Song and Y. Qian, Chin. Chem. Lett., 2004, 15, 1241–1244 CAS.
- R. Giannandrea, P. Mastrorilli, C. Nobile and G. Suranna, J. Mol. Catal., 1994, 94, 27–36 CrossRef CAS.
- Z. Jia, M. Liu and C. J. Li, Silver Catalysis in Organic Synthesis, 2019, pp. 645–660 Search PubMed.
- J. Roček, The Carbonyl Group, 1966, 1, 461–505 Search PubMed.
- A. Schmid, F. Hollmann and B. Bühler, Enzyme Catalysis in Organic Synthesis: A Comprehensive Handbook, 2002, pp. 1194–1202 Search PubMed.
- K.-J. Liu, Z. Wang, L.-H. Lu, J.-Y. Chen, F. Zeng, Y.-W. Lin, Z. Cao, X. Yu and W.-M. He, Green Chem., 2021, 23, 496–500 RSC.
- K.-J. Liu, J.-H. Deng, T.-Y. Zeng, X.-J. Chen, Y. Huang, Z. Cao, Y.-W. Lin and W.-M. He, Chin. Chem. Lett., 2020, 31, 1868–1872 CrossRef CAS.
- W.-B. He, L.-Q. Gao, X.-J. Chen, Z.-L. Wu, Y. Huang, Z. Cao, X.-H. Xu and W.-M. He, Chin. Chem. Lett., 2020, 31, 1895–1898 CrossRef CAS.
- T. R. Amarante, P. Neves, A. A. Valente, F. A. A. Paz, M. Pillinger and I. S. Gonçalves, J. Catal., 2016, 340, 354–367 CrossRef CAS.
- M. Dehbashi, M. Aliahmad, M. R. M. Shafiee and M. Ghashang, Phosphorus, Sulfur Silicon Relat. Elem., 2013, 188, 864–872 CrossRef CAS.
- S. Tanaka, Y. Kon, Y. Uesaka, R. Morioka, M. Tamura and K. Sato, Chem. Lett., 2016, 45, 188–190 CrossRef CAS.
- X. Yang, S. Tang, T. Lu, C. Chen, L. Zhou, Y. Su and J. Xu, Synth. Commun., 2013, 43, 979–985 CrossRef CAS.
- P. Marcé, J. Lynch, A. J. Blacker and J. M. Williams, Chem. Commun., 2016, 52, 1013–1016 RSC.
- K. Gilmore and R. Mohamed, Wiley Interdiscip. Rev.: Comput. Mol. Sci., 2016, 6, 487–514 CAS.
- M. Kiafar, M. A. Zolfigol, M. Yarie and A. A. Taherpour, RSC Adv., 2016, 6, 102280–102291 RSC.
- M. A. Zolfigol, M. Safaiee, F. Afsharnadery, N. Bahrami-Nejad, S. Baghery, S. Salehzadeh and F. Maleki, RSC Adv., 2015, 5, 100546–100559 RSC.
- M. Yarie, Iran. J. Catal., 2017, 7, 85–88 CAS.
- M. Yarie, Iran. J. Catal., 2020, 10, 79–83 CAS.
- I. V. Alabugin, L. Kuhn, M. G. Medvedev, N. V. Krivoshchapov, V. A. Vil’, I. A. Yaremenko, P. Mehaffy, M. Yarie, A. O. Terent’ev and M. A. Zolfigol, Chem. Soc. Rev., 2021, 50, 10253–10345 RSC.
- M. A. Zolfigol, F. Shirini and A. G. Choghamarani, Synthesis, 2006, 2006, 2043–2046 CrossRef.
- M. A. Zolfigol, F. Shirini, G. Chehardoli and E. Kolvari, J. Mol. Catal., 2007, 265, 272–275 CrossRef CAS.
- M. A. Zolfigol, M. Hajjami and A. Ghorbani-Choghamarani, J. Iran. Chem. Soc., 2012, 9, 13–18 CrossRef CAS.
- Z. Ahmadvand, M. Bayat and M. A. Zolfigol, J. Comput. Chem., 2020, 41, 2296–2309 CrossRef CAS PubMed.
- S. Babaee, M. Zarei, H. Sepehrmansourie, M. A. Zolfigol and S. Rostamnia, ACS Omega, 2020, 5, 6240–6249 CrossRef CAS PubMed.
- H. Sepehrmansourie, M. Zarei, M. A. Zolfigol, S. Babaee and S. Rostamnia, Sci. Rep., 2021, 11, 1–15 CrossRef PubMed.
- S. Babaee, M. Zarei, M. A. Zolfigol, S. Khazalpour, M. Hasani, U. Rinner, R. Schirhagl, N. Norouzi and S. Rostamnia, RSC Adv., 2021, 11, 2141–2157 RSC.
- H. Sepehrmansouri, M. Zarei, M. A. Zolfigol, A. R. Moosavi-Zare, S. Rostamnia and S. Moradi, Mol. Catal., 2020, 481, 110303 CrossRef CAS.
- A. M. Naseri, M. Zarei, S. Alizadeh, S. Babaee, M. A. Zolfigol, D. Nematollahi, J. Arjomandi and H. Shi, Sci. Rep., 2021, 11, 16817–16847 CrossRef CAS PubMed.
- S. Sen, N. N. Nair, T. Yamada, H. Kitagawa and P. K. Bharadwaj, J. Am. Chem. Soc., 2012, 134, 19432–19437 CrossRef CAS PubMed.
- S. P. Liu, M. Zhao, G. E. Sun, W. Gao and Q. Jiang, Phys. Chem. Chem. Phys., 2018, 20, 8341–8348 RSC.
- D.-F. Yu, P. Xing and B. Jiang, Tetrahedron, 2015, 25, 4269–4273 CrossRef.
- M. Kazemnejadi, S. A. Alavi, Z. Rezazadeh, M. A. Nasseri, A. Allahresani and M. Esmaeilpour, J. Mol. Struct., 2019, 1186, 230–249 CrossRef CAS.
- X.-J. Huang, F. Dong, L. Chen and Y.-Q. Li, Monatsh. Chem., 2008, 139, 1447–1451 CrossRef CAS.
- A. Varenikov, E. Shapiro and M. Gandelman, Chem. Rev., 2020, 121, 412–484 CrossRef PubMed.
- J. Zelenka, E. Svobodová, J. Tarábek, I. Hoskovcová, V. Boguschová, S. Bailly, M. Sikorski, J. Roithová and R. Cibulka, Org. Lett., 2019, 21, 114–119 CrossRef CAS PubMed.
- H. P. Kalmode, K. S. Vadagaonkar, S. L. Shinde and A. C. Chaskar, J. Org. Chem., 2017, 82, 3781–3786 CrossRef CAS PubMed.
Footnote |
† Electronic supplementary information (ESI) available. See DOI: 10.1039/d1ra05494e |
|
This journal is © The Royal Society of Chemistry 2021 |