DOI:
10.1039/D1RA04901A
(Paper)
RSC Adv., 2021,
11, 25695-25699
Formation of synthetically relevant CF3-substituted phenonium ions in superacid media†
Received
24th June 2021
, Accepted 14th July 2021
First published on 26th July 2021
Abstract
Predestined to be transient theoretical species, phenonium ions can now be considered as cationic intermediates of choice in organic synthesis. Here, we demonstrate that under non-nucleophilic and superacidic conditions, CF3-substituted phenonium ions can be generated to furnish original CF3-substituted dihydrostilbenes of interest.
Since the pioneering studies of Cram1 (Fig. 1a), considerable interest has been focused on phenonium ions. The first observations of the anthrylethyl bridged cation and p-anisonium ion respectively by Eberson, Winstein2 and Olah3 (Fig. 1b) triggered numerous studies to understand the stability/reactivity of these original cations.4 These studies greatly participated in shifting the phenonium ion from a fundamental phenomenon to a synthetically useful cationic intermediate.5 The participation of an aryl neighbouring group to form a phenonium ion after leaving group activation can surprisingly favour processes that should not occur without such a stabilization. This is especially evident when electron-withdrawing groups adorn the phenonium cation (Fig. 1c).6 In this context, fluorinated phenonium ions have also recently been elegantly exploited (Fig. 1d).7 Among destabilized carbocations, CF3-substituted carbocations8 are rarely considered as affordable intermediates for synthetic perspectives, despite an evident potential for modern applications to the design of fluorinated products.9 To the best of our knowledge, only one example of a CF3-substituted phenonium ion has been postulated.7e Herein, we describe the first evidence of this species and its selective exploitation to design trifluoromethylated dihydrostilbene derivatives (Fig. 1e).
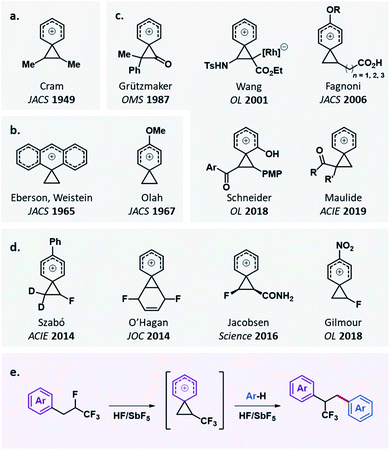 |
| Fig. 1 (a) First postulated phenonium ion; (b) first observed phenonium ions; (c) postulated destabilized phenonium ions; (d) postulated fluorinated phenonium ions; (e) this work. | |
In due course of our recent ongoing efforts to generate and exploit destabilized cations and polycations10,11 in superacid HF/SbF5,12 we thus envisioned to generate trifluoromethylated phenonium ions from CF3-substituted alcohols. To favour a substantial stabilization of the resonance-demanding phenonium ion by the lone pair of an amino group, 3-(4-(dimethylamino)phenyl)-1,1,1-trifluoropropan-2-ol 1 was chosen as a model substrate (Scheme 1a). Treatment of 1 with HF/SbF5 furnished a clean ionic species whose NMR signals did not match with the ones of a phenonium ion. Nevertheless, the clean spectra allowed to identify dication A as the sole species generated in solution. In the 1H NMR spectrum, the methyl groups of the amine function appear as a doublet at 1.84 ppm confirming the formation of the ammonium ion. The protonation of the OH group is also confirmed by the presence of a doublet at 9.72 ppm
13 and by the presence of a quadruplet in the 13C NMR spectrum at 119.2 ppm. This dicationic species was surprisingly stable up to 20 °C. Considering the difficulty to activate the C–OH bond geminal to the trifluoromethyl group, we turned our attention to the fluorinated analogue 2. This was especially motivated by previous results from Prakash and Olah: attempting to generate the p-anisonium ion from β-p-anisylethanol in superacid, only a dication coming from the protonation of alcohol and ether functions was observed.3,14 Interestingly, the targeted phenonium ion could be generated from the corresponding p-anisyl-2-chloroethane suggesting that the carbon–halogen bond could be activated in these conditions. Capitalizing on our experience on fluoroalkylamino reagents (FAR) and their exploitation in synthesis,15 substrate 2 could be generated in two steps from perfluoropropene and ready to be tested under similar superacid conditions (see ESI†). Compound 2 reacted in superacid at −40 °C leading to a mixture of ionic species (Scheme 1b). The 1H NMR spectrum resulting from this solution was difficult to interpret. However, in the 19F NMR spectrum, the signals at −83.8 ppm and −204.5 ppm confirmed the presence of ion B in solution. As for the alcohol derivative 1, the conditions were modified to tentatively favour the C–F bond activation by performing the reaction at higher temperature, but the reaction solution exhibited a complex mixture of different fluorinated species that were difficult to analyse. At −20 °C, after 10 min reaction, the reaction medium was hydrolysed and purified. The dihydrostilbene derivative 3 could be isolated from the reaction mixture in 14% yield. Although generated in a modest yield, the formation of this pseudodimeric compound could result from the reaction between the deactivated aromatic ring of the protonated ion B and the CF3-substituted phenonium superelectrophilic ion C.10 To confirm the involvement of ion C in the process, a deuterium labelling experiment was conducted in the presence of cyclohexane-d12 (Scheme 1c). With this nucleophilic source, any deuteration transfer reaction in superacid must occur through an ionic mechanism.11a,16 Under these conditions, in addition to compound 3, the deuterated compounds 4 and 5 could be isolated after one hour reaction of substrate 2.17 The formation of the reduced/deuterated products which must respectively originate from the insertion of a cation into a C–H/C–D bond of the alkane confirms the involvement of a CF3-substituted phenonium ion C. The regioselectivity of the reaction, identical for the formation of 3 and 4 is also in accordance with an ionic mechanism. Interestingly, the formation of compound 5 as a minor product confirms the highly destabilized character of the elusive α-CF3 carbocation.
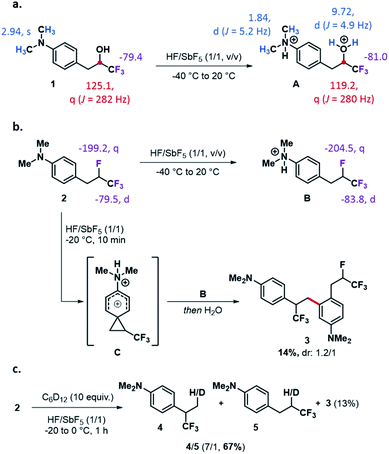 |
| Scheme 1 (a) Exclusive formation of dication A after reaction of 3-(4-(dimethylamino)phenyl)-1,1,1-trifluoropropan-2-ol 1 in HF/SbF5 at low temperature and characteristic NMR signals (δ in ppm); (b) reactivity of N,N-dimethyl-4-(2,3,3,3-tetrafluoropropyl)aniline 2 in HF/SbF5 and characteristic NMR signals (δ in ppm); (c) deuterium labelling experiment supporting the involvement of dication C. | |
Considering that the trifluoromethylated 1,2-diarylethane motif could be considered as an interesting pharmacophore for further applications,18 we explored the ability to regioselectively trap the phenonium ion C with representative arenes. As a first instance, and encouraged by the formation of product 3 from intermolecular addition of the aniline, acetanilide 6a was chosen as a model nucleophilic partner and 2 as a source of phenonium ion to screen the reaction conditions (Table 1). Gratifyingly, a first attempt in HF/SbF5 allowed to generate the targeted fluorinated product 7a, albeit in a modest 15% yield (Table 1, entry 1). Extending the reaction time and increasing the temperature throughout the reaction course was found ideal to favour a clean reaction (Table 1, entries 2–4) and product 7a could eventually be obtained in 97% yield. The necessity to use HF/SbF5 superacid conditions to activate the C–F bond19 was demonstrated by the recovery of the starting material after exploring the reaction in trifluoromethanesulfonic superacid solutions, and thus whatever conditions used (Table 1, entries 5-6). Trying to activate the C–F bond20 through a hydrogen bonding strategy in a hexafluoroisopropanol solution21 was unsuccessful (Table 1, entry 7). Lewis acid activation of the C–F bond22 was also explored with SbF5, BF3·OEt2 or B(C6F5)3 but remained inefficient (Table 1, entries 8–10). This seems to confirm that highly acidic conditions and the absence of any nucleophilic counter-anions in solution23 are needed to activate the C–F bond that is geminal to the CF3 group to form the targeted phenonium ion.
Table 1 Optimization of the reaction conditions between phenonium ion precursor 2 and acetanilide 6a

|
Entry |
Acida (v/v) |
Conditions |
Yieldb [%] |
Used as solvent, unless stated otherwise. 7a was isolated in each case as a 1/1 mixture of para/meta isomers. Recovery of the starting material. Complex mixture. In CH2Cl2. In MeNO2. |
1 |
HF/SbF5 (1/1) |
−20 °C, 0.5 h |
15 |
2 |
HF/SbF5 (1/1) |
−20 °C, 4 h |
65 |
3 |
HF/SbF5 (1/1) |
−20 to 0 °C, 0.5 h |
71 |
4 |
HF/SbF5 (1/1) |
−20 to 0 °C, 1 h |
97 |
5 |
TfOH |
20 °C, 1 h |
0c |
6 |
TfOH/SbF5 (1/1) |
−20 to 0 °C, 1 h |
0d |
7 |
HFIP/CH2Cl2 (1/1) |
20 °C, 16 h |
0c |
8 |
SbF5 |
0 to 20 °C, 1 h |
0c |
9 |
BF3·OEt2 (10 equiv.)e |
20 °C, 1 h |
0c |
10 |
B(C6F5)3 (5 mol%)f |
20 °C, 16 h |
0c |
With these optimized conditions in hand, to determine whether the external aromatic nucleophilic trapping of the CF3-substituted phenonium ion can be extended to more elaborate substrates, compound 2 was submitted to superacid in the presence of different aromatic partners (Fig. 2).
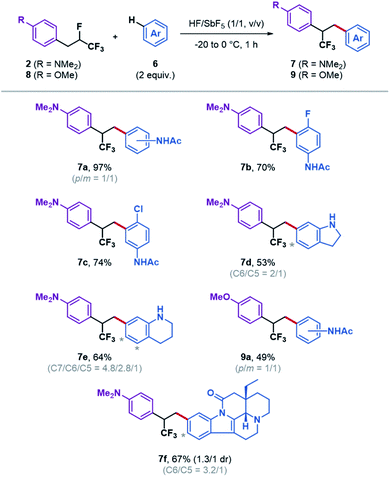 |
| Fig. 2 Formation of CF3-containing diarylethanes 7 and 9 in superacid. | |
The reaction could be applied to the synthesis of halogen-substituted products 7b and 7c in good yields after reaction with 4-fluoro and 4-chloroacetanilide. Not limited to aromatic amides, the reaction was also found to be efficient with indoline and tetrahydroquinoline affording the trifluoromethylated dihydrostilbenes 7d and 7e.24 The regioselectivity of the Friedel–Crafts type reaction is dictated by the orientating effect of the alkyl chain, the amine being protonated under its ammonium form under these conditions. The reaction was found not limited to the trapping of the phenonium ion C, as its oxygenated analogue that must be generated from substrate 8 could also be trapped by acetanilide to generate product 9a. At this stage it is also important to note that the reaction is highly regioselective, as the phenonium ion is selectively opened at the external position, in accordance with an ionic mechanism. No steric or electronic effect from the nucleophilic partner seems to affect this selectivity. To test this method on a more complex target, such as those that might be encountered in pharmaceutical research, vinburnine was tested as nucleophilic partner. Delightfully, the corresponding trifluoromethylated diarylethane analogue 7f could be generated in 67% yield as a mixture of regio- and diastereomers.
To conclude, the ability to generate CF3-containing phenonium ions in superacid HF/SbF5 was demonstrated. These cations can react with poor nucleophilic partners such as deactivated nitrogen-containing arenes, suggesting a superelectrophilic character for this family of cations. Following recent and excellent contributions demonstrating the synthetic utility of phenonium ions, this work opens perspectives to exploit their CF3-substituted counterpart for synthetic purposes.
Conflicts of interest
There are no conflicts to declare.
Acknowledgements
We gratefully acknowledge the Frontier Research in Chemistry Foundation (FRC), the Université de Strasbourg (grant FLE-FRC-0002-0035, SuperFlOx), the Université de Poitiers, and the CNRS for financial support. The authors also acknowledge financial support from the European Union (ERDF) and the Région Nouvelle Aquitaine (SUPERDIV project-HABISAN program). The authors thank the French fluorine network (GIS-FLUOR).
Notes and references
-
(a) D. J. Cram, J. Am. Chem. Soc., 1949, 71, 3863 CrossRef CAS
;
(b) D. J. Cram and R. Davies, J. Am. Chem. Soc., 1949, 71, 3871 CrossRef CAS
. - L. Eberson and S. Winstein, J. Am. Chem. Soc., 1965, 87, 3506 CrossRef CAS
. - G. A. Olah, M. B. Comisarow, E. Namanworth and B. G. Ramsey, J. Am. Chem. Soc., 1967, 89, 5259 CrossRef CAS
. - For selected analytical and theoretical studies on phenonium ions, please see:
(a) G. A. Olah, N. J. Head, G. Rasul and G. K. S. Prakash, J. Am. Chem. Soc., 1995, 117, 875 CrossRef CAS
;
(b) G. A. Olah and R. Dudley Porter, J. Am. Chem. Soc., 1970, 92, 7627 CrossRef CAS
;
(c) J. A. Manner, J. A. Cook Jr and B. G. Ramsey, J. Org. Chem., 1974, 39, 1199 CrossRef CAS
;
(d) G. A. Olah, S. Hamanaka, J. A. Wilkinson and J. A. Olah, Proc. Natl. Acad. Sci. U. S. A., 1992, 89, 915 CrossRef CAS PubMed
;
(e) E. del Rio, M. I. Menéndez, R. López and T. L. Sordo, J. Phys. Chem. A, 2000, 104, 5568 CrossRef CAS
;
(f) E. del Rio, M. I. Menéndez, R. López and T. L. Sordo, J. Am. Chem. Soc., 2001, 123, 5064 CrossRef CAS PubMed
;
(g) B. Guizzardi, M. Mella, M. Fagnoni and A. Albini, J. Org. Chem., 2003, 68, 1067 CrossRef CAS PubMed
;
(h) I. Manet, S. Monti, G. Grabner, S. Protti, D. Dondi, V. Dichiarante, M. Fagnoni and A. Albini, Chem.–Eur. J., 2008, 14, 1029 CrossRef CAS PubMed
;
(i) Y. Tsuji and J. P. Richard, J. Phys. Org. Chem., 2015, 29, 557 CrossRef PubMed
. - For selected recent examples mentioning phenonium ions intermediates in key synthetic reactions, please see:
(a) S. Xu, H. M. Holst, S. B. McGuire and N. J. Race, J. Am. Chem. Soc., 2020, 142, 8090 CrossRef CAS PubMed
;
(b) X. Lei, Y. Li, Y. Lai, S. Hu, C. Qi, G. Wang and Y. Tang, Angew. Chem., Int. Ed., 2021, 60, 4221 CrossRef CAS PubMed
;
(c) L. Min, W. Yang, Y. Weng, W. Zheng, X. Wang and Y. Hu, Org. Lett., 2019, 21, 2574 CrossRef CAS PubMed
;
(d) Y. H. Jang and S. W. Youn, Org. Lett., 2014, 16, 3720 CrossRef CAS PubMed
;
(e) O. E. Okoromoba, Z. Li, N. Robertson, M. S. Mashuta, U. R. Couto, C. F. Tormena, B. Xu and G. B. Hammond, Chem. Commun., 2016, 52, 13353 RSC
. For a recent review, please see:
(f) H. M. Holst, S. B. McGuire and N. J. Race, Synlett, 2021, 32, 1 CrossRef CAS
. - For selected examples mentioning phenonium ions substituted with electron withdrawing groups, please see:
(a) A.-M. Dommröse and H.-F. Grützmacher, J. Mass Spectrom., 1987, 22, 437 Search PubMed
;
(b) N. Jiang, Z. Qu and J. Wang, Org. Lett., 2001, 3, 2989 CrossRef CAS PubMed
;
(c) S. Protti, M. Fagnoni and A. Albini, J. Am. Chem. Soc., 2006, 128, 10670 CrossRef CAS PubMed
;
(d) A. Suneja and C. Schneider, Org. Lett., 2018, 20, 7576 CrossRef CAS PubMed
;
(e) J. Li, A. Bauer, G. Di Mauro and N. Maulide, Angew. Chem., Int. Ed., 2019, 58, 9816 CrossRef CAS PubMed
. - For selected examples mentioning fluorinated
phenonium ions, please see:
(a) N. O. Ilchenko, B. O. A. Tasch and K. J. Szabó, Angew. Chem., Int. Ed., 2014, 53, 12897 CrossRef CAS PubMed
;
(b) A. J. Durie, T. Fujiwara, N. Al-Maharik, A. M. Z. Slawin and D. O'Hagan, J. Org. Chem., 2014, 79, 8228 CrossRef CAS PubMed
;
(c) S. M. Banik, J. W. Medley and E. N. Jacobsen, Science, 2016, 353, 51 CrossRef CAS PubMed
;
(d) B. Zhou, M. K. Haj, E. N. Jacobsen, K. N. Houk and X.-S. Xue, J. Am. Chem. Soc., 2018, 140, 15206 CrossRef CAS PubMed
;
(e) F. Scheidt, J. Neufeld, M. Schäfer, C. Thiehoff and R. Gilmour, Org. Lett., 2018, 20, 8073 CrossRef CAS PubMed
. - A. J. Fernandes, A. Panossian, B. Michelet, A. Martin-Mingot, F. R. Leroux and S. Thibaudeau, Beilstein J. Org. Chem., 2021, 17, 343 CrossRef CAS PubMed
. -
(a) P. Kirsch, Modern Fluoroorganic Chemistry: Synthesis, Reactivity, Applications, 2nd edn, 2013, Wiley-VCH CrossRef
;
(b) I. Ojima, Fluorine in Medicinal Chemistry and Chemical Biology, 2009, Wiley-Blackwell, Chichester CrossRef
;
(c) H. Groult, F. Leroux and A. Tressaud, Modern Synthesis Processes and Reactivity of Fluorinated Compounds, 2017, Elsevier Science, London Search PubMed
;
(d) G. Haufe and F. Leroux, Fluorine in Life Sciences: Pharmaceuticals, Medicinal Diagnostics, and Agrochemicals, 2019, Academic press, London Search PubMed
;
(e) R. Littich and P. J. H. Scott, Angew. Chem., Int. Ed., 2012, 51, 1106 CrossRef CAS PubMed
;
(f) S. Preshlock, M. Tredwell and V. Gouverneur, Chem. Rev., 2016, 116, 719 CrossRef CAS PubMed
;
(g) R. Berger, G. Resnati, P. Metrangolo, E. Weber and J. Hulliger, Chem. Soc. Rev., 2011, 40, 3496 RSC
;
(h) D. O'Hagan, Chem. Soc. Rev., 2008, 37, 308 RSC
;
(i) J.-P. Bégué and D. Bonnet-Delpon, Bioorganic and Medicinal Chemistry of Fluorine, 2008, Wiley, New Jersey CrossRef
. - G. A. Olah and D. Klumpp, Superelectrohiles and Their Chemistry, 2008, John Wiley and Sons, New York Search PubMed
. -
(a) A. Martin, A. Arda, J. Désiré, A. Martin-Mingot, N. Probst, P. Sinaÿ, J. Jiménez-Barbero, S. Thibaudeau and Y. Blériot, Nat. Chem., 2016, 8, 186 CrossRef CAS PubMed
;
(b) L. Lebedel, A. Ardá, A. Martin, J. Désiré, A. Mingot, M. Aufiero, N. A. Font, R. Gilmour, J. Jiménez-Barbero, Y. Blériot and S. Thibaudeau, Angew. Chem., Int. Ed., 2019, 58, 13758 CrossRef CAS PubMed
;
(c) N. Bhuma, L. Lebedel, H. Yamashita, Y. Shimizu, Z. Abada, A. Ardá, J. Désiré, B. Michelet, A. Martin-Mingot, A. Abou-Hassan, M. Takumi, J. Marrot, J. Jiménez-Barbero, A. Nagaki, Y. Blériot and S. Thibaudeau, Angew. Chem., Int. Ed., 2021, 60, 2036 CrossRef CAS PubMed
;
(d) G. Compain, A. Martin-Mingot, G. Frapper, C. Bachmann, M.-P. Jouannetaud and S. Thibaudeau, Chem. Commun., 2012, 48, 5877 RSC
;
(e) M. Artault, N. Mokhtari, T. Cantin, A. Martin-Mingot and S. Thibaudeau, Chem. Commun., 2020, 56, 5905 RSC
. - Superacids, ed. G. A. Olah, G. K. S. Prakash, A. Molnar and J. Sommer, 2nd edn, 2009, Wiley Intersciences, New-York Search PubMed
. - G. A. Olah and E. Namanworth, J. Am. Chem. Soc., 1966, 88, 5327 CrossRef CAS
. -
(a) G. A. Olah and R. D. Porter, J. Am. Chem. Soc., 1971, 93, 6877 CrossRef CAS
;
(b) G. A. Olah, N. J. Head, G. Rasul and G. K. S. Prakash, J. Am. Chem. Soc., 1995, 117, 875 CrossRef CAS
. -
(a) S. Pazenok, F. Giornal, G. Landelle, N. Lui, J.-P. Vors and F. R. Leroux, Eur. J. Org. Chem., 2013, 4249 CrossRef CAS
;
(b) F. Giornal, G. Landelle, N. Lui, J.-P. Vors, S. Pazenok and F. R. Leroux, Org. Process Res. Dev., 2014, 18, 1002 CrossRef CAS
;
(c) E. Schmitt, G. Landelle, J.-P. Vors, N. Lui, S. Pazenok and F. R. Leroux, Eur. J. Org. Chem., 2015, 6052 CrossRef CAS
;
(d) E. Schmitt, B. Rugeri, A. Panossian, J.-P. Vors, S. Pazenok and F. R. Leroux, Org. Lett., 2015, 17, 4510 CrossRef CAS PubMed
;
(e) F. Aribi, E. Schmitt, A. Panossian, J.-P. Vors, S. Pazenok and F. R. Leroux, Org. Chem. Front., 2016, 3, 1392 RSC
;
(f) E. Schmitt, A. Panossian, J.-P. Vors, C. Funke, N. Lui, S. Pazenok and F. R. Leroux, Chem.–Eur. J., 2016, 22, 11239 CrossRef CAS PubMed
;
(g) B. Commare, E. Schmitt, F. Aribi, A. Panossian, J.-P. Vors, S. Pazenok and F. R. Leroux, Molecules, 2017, 22, 977–981 CrossRef PubMed
;
(h) E. Schmitt, S. Bouvet, B. Pegot, A. Panossian, J.-P. Vors, S. Pazenok, E. Magnier and F. R. Leroux, Org. Lett., 2017, 19, 4960 CrossRef CAS PubMed
;
(i) F. Aribi, A. Panossian, J.-P. Vors, S. Pazenok and F. R. Leroux, Eur. J. Org. Chem., 2018, 3792 CrossRef CAS
;
(j) E. Schmitt, B. Commare, A. Panossian, J.-P. Vors, S. Pazenok and F. R. Leroux, Chem.–Eur. J., 2018, 24, 1311 CrossRef CAS PubMed
. - J. Sommer and J. Bukala, Acc. Chem. Res., 1993, 26, 370 CrossRef CAS
. - NMR analysis showed a 35% deuterium incorporation for compound 4; deuterium incorporation for compound 5 could not have been determined due to signal overlapping.
-
(a) For an example of 1,2-fluorinated diarylethane, please see: M. O'Duill, E. Dubost, L. Pfeifer and V. Gouverneur, Org. Lett., 2015, 17, 3466 CrossRef PubMed
; For recent reports on bioactive natural dihydrostilbenes, please, see:
(b) L. He, Q. Su, L. Bai, M. Li, J. Liu, X. Liu, C. Zhang, Z. Jiang, J. He, J. Shi, S. Huang and L. Guo, Eur. J. Med. Chem., 2020, 204, 112530 CrossRef CAS PubMed
;
(c) L. W. K. Moodie, R. Trepos, G. Cervin, K. A. Bråthen, B. Lindgård, R. Reiersen, P. Cahill, H. Pavia, C. Hellio and J. Svenson, J. Nat. Prod., 2017, 80, 2001 CrossRef CAS PubMed
;
(d) Y. Zhu, W.-H. Pan, C. F. Ku, H.-J. Zhang and S. W. Tsang, Eur. J. Med. Chem., 2018, 143, 1254 CrossRef CAS PubMed
. - For a seminal contribution on C–F bond activation in superacid, please see: G. A. Olah, Y. K. Mo and Y. Halpern, J. Org. Chem., 1972, 37, 1169 CrossRef CAS
. - For reviews on C–F bond activation, please see:
(a) H. Amii and K. Uneyama, Chem. Rev., 2009, 109, 2119 CrossRef CAS PubMed
;
(b) T. Ahrens, J. Kohlmann, M. Ahrens and T. Braun, Chem. Rev., 2015, 115, 931 CrossRef CAS PubMed
. - P. A. Champagne, Y. Benhassine, J. Desroches and J.-P. Paquin, Angew. Chem., Int. Ed., 2014, 53, 13835 CrossRef CAS PubMed
. - T. Stahl, H. F. T. Klare and M. Oestreich, ACS Catal., 2013, 3, 1578 CrossRef CAS
. - I. Krossing and I. Raabe, Angew. Chem., Int. Ed., 2004, 43, 2066 CrossRef CAS PubMed
. - For recent studies on bioactive properties of non-trifluoromethylated analogues of 7d and 7e, please see:
(a) H. S. Sutherland, A. S. T. Tong, P. J. Choi, D. Conole, A. Blaser, S. G. Franzblau, C. B. Cooper, A. M. Upton, M. U. Lotlikar, W. A. Denny and B. D. Palmer, Bioorg. Med. Chem., 2018, 26, 1797 CrossRef CAS PubMed
;
(b) T. D. Aicher, C. B. Taylor and C. A. Vanhuis, WO2015171610, A2, 2015
.
Footnote |
† Electronic supplementary information (ESI) available. See DOI: 10.1039/d1ra04901a |
|
This journal is © The Royal Society of Chemistry 2021 |
Click here to see how this site uses Cookies. View our privacy policy here.