DOI:
10.1039/D1RA04708F
(Paper)
RSC Adv., 2021,
11, 27837-27844
A novel quercetin/β-cyclodextrin transdermal gel, combined or not with therapeutic ultrasound, reduces oxidative stress after skeletal muscle injury
Received
17th June 2021
, Accepted 31st July 2021
First published on 17th August 2021
Abstract
A gel containing the inclusion complex of quercetin and β-cyclodextrin was developed in order to verify its effects, isolated or using phonophoresis, on oxidative biomarkers after skeletal muscle injury. 30 male rats were divided into one of five groups: Control (CTRL), Muscle Injury (MI), Therapeutic Pulsed Ultrasound (TPU), Therapeutic Pulsed Ultrasound plus Quercetin (TPU plus gel-QUE) or Quercetin gel (QUE). Quercetin gel was complexed with β-Cyclodextrin (β-CD) using chromatography (HPLC). TPU and quercetin application occurred with 2, 12, 24, 48, 72, 96 hours intervals after injury. Gastrocnemius muscle was injured by mechanical trauma. Lipid peroxidation, superoxide dismutase activity, and catalase activity were assessed. The inclusion complex exhibited adequate entrapment efficiency, relative density and pH. The viscosity of the complex showed a non-Newtonian pseudoplastic behavior. Quercetin/β-cyclodextrin gel reduced lipid peroxidation, superoxide dismutase activity and catalase activity compared to muscle injury group. Similarly, phonophoresis and TPU also reduced the levels of these oxidative biomarkers. In conclusion, quercetin/β-cyclodextrin transdermal gel reduces oxidative stress biomarkers after skeletal muscle injury irrespective of using phonophoresis.
Introduction
Muscle injury can occur in leisure or sports activity, but it is more common in sports with an estimated incidence of 40% in international athletics championships.1 It involves many tissue changes such as fiber damage, edema and inflammatory cell infiltration, which are related to the oxidative stress.2 Indeed, excessive oxidative stress is harmful to the cells, causing oxidation of important biomolecules such as lipids and carbonyls.3 Therefore, novel therapeutic modalities, such as phonophoresis, have been proposed to address the negative effects of the oxidative stress on the pathway of the musculoskeletal injuries.4
Increasing antioxidant capacity can reduce oxidative stress after muscle injuries. Natural antioxidants drugs have been suggested to prevent and to manage muscle injuries.5–7 Plant extracts and oils have shown notable effects on reducing oxidative and inflammatory markers.8–10 However, the administration mode of these drugs has some implications. In fact, oral administration causes more adverse events than topical application and it is important to highlight that topical application has been shown to be equally effective to the oral administration but with fewer adverse events.11 At the same time, some drugs have poor solubility making topical application difficult. For instance, quercetin is a potent plant-derived antioxidant but it has low water solubility, thus its topical use is limited. A strategy to improve permeability of drugs with a low solubility profile is the inclusion with β-cyclodextrin (β-CD), cyclic oligosaccharides with a hydrophilic external surface and a hydrophobic internal surface.12
Quercetin is widely researched and is the most abundant flavonoids in plants and plant-derived food. It is mainly highlighted for its prominent antioxidant capacity, which provides several health benefits. Quercetin seems to be effective in the management of several diseases related to ageing, such as cancer, cardiovascular and neurodegenerative pathologies.13 Among the flavonoids, quercetin is a potent scavenger of reactive oxygen species (ROS) and reactive nitrogen species (TNS), including O2−, NO and ONOO−. The antioxidant activity of this flavonoid measured by DPPH (stable 2,2-diphenylpicrylhydrazyl free radical) method is reported as 89.8%.14 This potent antioxidant capacity is attributed, in part, to the presence of two pharmacophores in the molecule that have the optimal configuration for free radical scavenging, i.e. catechol group as ring B and 3-OH group in the A–C ring.15
Phonophoresis has been used to enhance topical drugs delivery by using Therapeutic Pulsed Ultrasound (TPU) and potentially can reduce oxidative stress in muscle injuries.4–10 It is already known that phonophoresis has marked effects on inflammatory response, reducing inducible nitric oxide synthase (iNOS), inflammatory infiltrate and edema.16–18 Its effects on oxidative stress have been observed in distinct experimental models and with many different gel formulations. In models of experimentally induced muscle injury, phonophoresis with dimethylsulfoxide (DMSO) and phonophoresis with gold nanoparticles decreased lipid peroxidation and superoxide dismutase activity compared to muscle injury without treatment in rats.4–19 In these studies, neither DMSO nor gold nanoparticles were formulated using solubility-enabling formulation approaches to enhance skin penetration.
Given that quercetin is a flavonoid with antioxidant and anti-inflammatory activity and that it has poor solubility, the aim of this study was to develop a gel containing quercetin/β-cyclodextrin inclusion complex and to verify its effects, isolated or in combination with TPU (phonophoresis), on muscle oxidative markers in rats.
Experimental section
Sample preparation
The formulation was obtained by the addition 302.2 mg of quercetin (C15H10O7·2H2O, FW 338.3, lot: 109HO882, Sigma-Aldrich Saint Louis-USA) to a beaker containing 1.135 mg of β-CD (purity: 97%, Sigma-Aldrich, lot number: #041M1759V), dissolved in water (20 mL/40 °C), 1
:
1 molar ratio. The mixture was stirred for 36 hat room temperature. For obtainment of semisolid formulation, the suspension was incorporated in Carbopol gel at 10% for the physicochemical characterization and biological tests.
Karl Fisher titration
The water contents of quercetin free was determined through the Karl Fischer method (Metrohm, 870 KF Titrino Plus, USA) and methanol (Fluka, USA) as titrating solution. The analyses were carried out in triplicate.
Physicochemical characterization of suspension
Differential scanning calorimetry (DSC). DSC curves of quercetin free and suspension were obtained in a DSC-50 cell (Shimadzu) using aluminum crucibles with about 2 mg of samples, under dynamic nitrogen atmosphere (50 mL min−1) and heating rate of 10 °C min−1 in the temperature range from 25 to 600 °C. The DSC cell was calibrated with indium (mp 156.6 °C; ΔHmelting = 28.54 J g−1) and zinc (mp 419.6 °C).
Entrapment efficiency (EE%) of quercetin by high performance liquid chromatography (HPLC). For the chromatographic analysis, was prepared a standard solution (100 μg mL−1) of quercetin free (C15H10O7; Sigma-Aldrich) dissolved in methanol (HPLC grade, Panreac, Darmstadt, Germany) which was submitted ultrasonic bath for 10 minutes. Before the HPLC injection, the solution was filtered through a 0.45 μm membrane filter (PTFE). The HPLC analysis were performed using the high performance liquid chromatography system that consisted of a degasser DGU-20A3, two LC-20AD pumps, a SIL-20A HT auto injector, CTO-20A column oven, SPDM20Avp photodiode array detector (DAD) and a CBM-20A system controller (Shimadzu Co., Kyoto, Japan). The chromatographic separation was performed using a Phenomenex Luna C18 analytical column 150 × 4.6 mm (5 μm particle size). The injection volume was 20 μL and the mobile phase flow rate was 1.0 mL min−1. The solvents used to the mobile phase were: A, 0.1% acetic acid (Neon, São Paulo, Brazil) in water (Milli-Q system, Millipore, Bedford, USA) and B, methanol. The elution profile was an isocratic mode (30/70 – A/B) during 10 minutes of analysis. Detector was set at 320 nm for acquiring chromatogram. The method linearity was evaluated with a calibration curve in five concentrations (20–100 μg mL−1).The amount of active compound entrapped in the inclusion complex particles was determined by HPLC for suspension 1
:
1 method, 10 mg of sample was dissolved in 10 mL of methanol and left for 24 h after being well mixed (250 rpm). After this procedure, the solution were centrifuged at 3500 rpm for 20 minutes to remove any β-CD from the solution, leaving only the active compound. 1 mL of the supernatant was collected, diluted in 5 mL of methanol, filtered on a 0.45 μm membrane filter (PTFE) and analyzed by HPLC. The EE% was calculated:
where,
C1 is the quercetin amount present in the inclusion complex and
C2 is quercetin amount initially used to prepare the inclusion complex with β-CD.
Physicochemical characterization of gel
The physicochemical properties were evaluated in terms of pH, specific gravity and spreadability.
pH
The pH of the formulation was evaluated using a digital potentiometer with a glass electrode and temperature sensor pH Phtek 3B mark, previously calibrated with pH 4.0 and 7.0 buffer solutions at a temperature of 25 ± 0.5 °C. To perform the analysis, 1 g sample was diluted in 10 mL of water is stirred on a magnetic stirrer for 5 minutes.
Density
The relative density of the formulation was determined by pycnometer method and performed in triplicate. The value of the relative density is obtained by the equation: |
Psample = Msample/Mwater
| (2) |
at where Msample = m2 − m1 is the mass of the sample in question occupying the volume V of the pycnometer, and Mwater = m3 − m1 is the mass of pure water occupying the same volume V, where m1 the mass of the empty pycnometer; m2 the mass of the pycnometer filled with the sample and m3 mass of the pycnometer filled with pure water.
Spreadability
The spreadability of the samples was determined by the method of successive plates.20 The sample was placed on a glass plate on a graph paper. Then other known weight plates are superposed successively on the sample after one minute intervals. At each time interval was performed to read the covered diameters. Readings were taken in triplicate and results are expressed as spreadability of the sample as a function of the applied weight, according to the equation below.at where S = the sample weight spreadability for a given (mm2); d = average diameter.
Rheology study
The rheological analysis of the formulation was carried out using a rotational rheometer (DHR, Discovery Hybrid Rheometer: TA instrument). The upward flow curve was measured at 25 °C, shear rate of 0 to 100 rpm. Data were obtained every 5 seconds, totaling 50 points. The result was expressed by relating the viscosity with change in shear rate.
Lipid peroxidation, superoxide dismutase and catalase activity
Animals. Male Wistar rats (10–12 weeks old, 250–300 g) were obtained from the Central Animal Facility of the Federal University of Sergipe (UFS). It was maintained in an animal cage (5 rats per cage) with food and water free, in a room at temperature of 24 °C and relative humidity of 55%, and under 12 hour alternating light–dark cycles. The study protocol was revised and approved by the Institutional Animal Ethic Committee (CEPA/UFS, protocol #48/2015) following norms and ethical principles of National Health Institute.
Experimental procedures
The animals were allocated in five different groups with 8 animals in each group. Control group (CTRL) – animals without injury and no treatment; muscle injury group (MI) – animals with injury but without treatment; therapeutic pulsed ultrasound group (TPU) – animals with injury treated with pulsed ultrasound and saline gel (0.9%); therapeutic pulsed ultrasound plus quercetin group (TPU plus gel-QUE) – animals with injury treated with pulsed ultrasound plus quercetin complexed gel (5%, 70 mg); quercetin group (QUE) – animals with injury treated with quercetin complexed gel (5%, 70 mg) applied topically. The rats were anaesthetized with ketamine (70 mg kg−1, Ceva/Sao Paulo, Brazil) and xilazin (15 mg kg−1, Ceva/Sao Paulo Brazil), then the paw was shaved and gastrocnemius muscle was injured by single and abrupt impact though mechanical press (CIDEP/RS, Brazil).21 The trauma was produced by a metallic mass (0.459 kg) falling from height of 18 cm on the gastrocnemius muscle (Fig. 1). To better define animal positioning in the device, a simulation, where the mass was put close to the muscle (without falling), was performed before the induction of the injury. Therapeutic pulsed ultrasound (Ibramed, Amparo, Brazil) treatment was conducted by pulsed mode, effective radiating area (ERA) of 1 cm2, 50% duty cycle, frequency of 1 MHz, duration of 6 min and a spatial and temporal average (SATA) intensity of 0.8 W cm−2. The pulse repetition frequency (PRF) was 100 Hz (pulses 10 ms long). The area was treated about 2 cm (ref. 21) with circular movements and following lesion focus.22 Groups treated by TPU (TPU and TPU plus gel-QUE groups) received 6 minutes application with 2, 12, 24, 48, 72, 96 hours intervals after injury. Group QUE received quercetin complexed gel administered topically during 6 minutes in these same hour intervals. Quercetin complexed gel (5%, 70 mg) was applied over the injury site through a sterile cotton swab and performing circular movements to facilitate the spreading of the gel. The gel was stored in a refrigerator at 4–8 °C throughout the experiment (96 h).
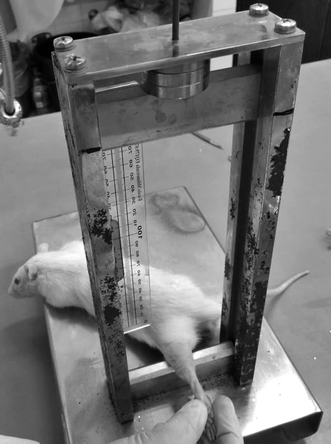 |
| Fig. 1 Muscle injury device. The trauma was produced by the metallic mass (0.459 kg) falling from height of 18 cm on the gastrocnemius muscle. | |
Two hours after last treatment application, that is, 98 h after lesion induction, the animals were sacrificed by guillotine. Injured muscle was surgically removed and stocked at −70 °C. Muscle samples were homogenized in specific buffers, according to each analysis protocol. Lipid peroxidation was analyzed by thiobarbituric acid reactive substances formation during TBA reaction, as described by Draper & Hadley (1990).23 Samples were mixed with tricloroacetic acid (10%) 1 mL plus thiobarbituric acid (0.67%) 1 mL. Later, it was heated in a water bath for 30 min. TBARS were determined by absorbance at 532 nm. The results were expressed in nmol mg−1 protein. Superoxide dismutase (SOD) activity was assessed by measuring the inhibition of epinephrine auto oxidation in absorbance at 480 nm as described by Bannister & Calabrese (1987).24 The results were expressed as U SOD per mg protein. Catalase (CAT) was measured by the drop rate of hydrogen peroxide by absorbance at 240 nm (Aebi 1984)25 and the results were expressed as U CAT per mg protein.
Statistical analysis
Data are expressed as mean ± standard deviation (SD). After assessing Q–Q plots, the distribution of data was considered to be normal. The results were statistically analyzed using one-way analysis of variance (ANOVA) followed by Bonferroni test, considering P < 0.05 significant. The software used for the analysis was GraphPad Prism 8.
Results and discussion
The DSC curves are shown in the Fig. 2. Quercetin free form DSC curve showed two endothermic events in the range of 25–500 °C. The first event was observed in the range of 109–136 °C and was related to release of water from the your crystal lattice as reported by Koontz et al. (2009).26 The quercetin exists as a mixture of dehydrate and monohydrate forms at ambient humidity with a calculated 1.7 mol of H2O per mole of quercetin or 9.03 ± 0.36% of H2O as been determined by Karl Fisher titration. Thus, this temperature is much higher than the boiling point of water, which indicates that the water molecules are strongly held by quercetin through hydrogen bonding.27 The second endothermic event was observed at 318–328 °C indicating the melting point of quercetin as reported by Sri et al. (2007).28 β-CD showed a very broad endothermic event, between 48 and 155 °C corresponding to dehydration molecule, followed a degradation process, which took place at around 300 °C.29 The DSC profile of suspension obtained by β-CD and quercetin was observed an endothermic event around 120–148 °C probably related some quercetin not complexed inside β-CD cavity. At 306–326 °C was observed another endothermic event related the degradation of compounds of system. In this event was not possible to differentiate the compounds because melting point of quercetin and β-CD occurred in the similar step.
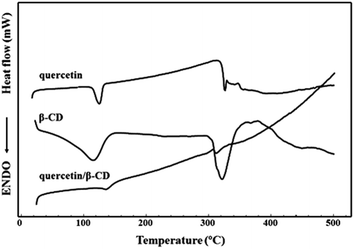 |
| Fig. 2 DSC curves of quercetin free form, β-CD and inclusion complex. | |
Fig. 3 shows quercetin chromatogram by HPLC (320 nm). The chromatographic peak had retention time 2.92 minutes. The EE% is a quantitative parameter used to calculate the amount of active compound entrapped in the inclusion complex. The EE% was calculated using eqn (1). The suspension 1
:
1 method showed EE 85%. This result corroborates with DSC analysis that showed a little amount of quercetin not complexed in the suspension (probably 15%). Thus, the suspension method was adequate to complexation of quercetin with β-CD once the most amount of quercetin was entrapped in the supramolecular structure.
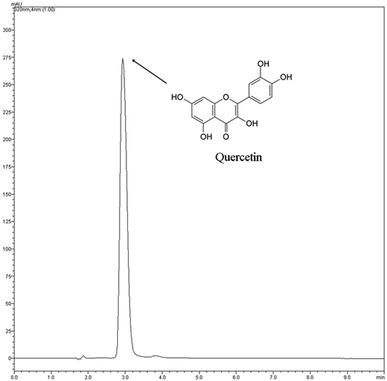 |
| Fig. 3 Quercetin chromatographic profile by HPLC (320 nm). | |
Regarding gel formulation, the Fig. 4 shows the aspect of the formulation. pH of the sample was analyzed in triplicate, yielding a mean value of 6.79 (SD 0.18). Although this pH value is slightly higher than the pH of the human skin, which is normally between 4.5 and 6.5,30 no signs of skin irritation were observed. Other studies have investigated formulations with pH values around 7 and have also not found skin changes.31–33 Therefore, the pH of 6.79 is considered to be adequate for drug permeation through skin.
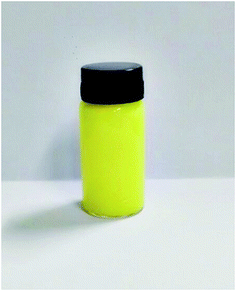 |
| Fig. 4 Macroscopic aspect of gel containing quercetin/β-CD 1 : 1. | |
The relative density of the formulation was measured by relating its absolute density with absolute density of water. The value obtained was 0.97 g mL−1 (SD 0.01), this being appropriate value as it approaches the relative density of water (approximately 1 g mL−1). Values below or above 1 g mL−1 can trigger physical instability of the formulation and favor microbial growth, respectively.34
Through the spread ability test formulation was possible to relate the spreading area with the force applied on it. In the present study, it was observed that the formulation developed is easily spread with a small amount of force (Fig. 5) and the area covered by scattering increases as the strength is increased. This result indicates that the application of the formulation will be pleasant and to a lesser extent, ensuring its effectiveness.20,34
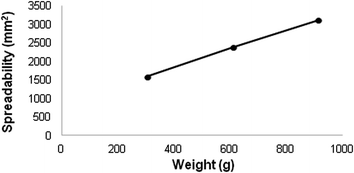 |
| Fig. 5 Spread ability profile of Carbopol gel containing quercetin/β-CD 10%. | |
Fig. 6 represents the viscosity obtained with varying shear rates. According to the results, it can be seen that the viscosity decreases as the shear rate increases, revealing that the developed formulation has a non-Newtonian pseudoplastic behavior. Such behavior indicates that the initial resistance of the formulation decreases as a force is exerted on it, facilitating its implementation, which is preferable for topical formulations and reflects directly on its use and effectiveness.35,36
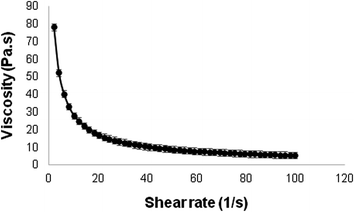 |
| Fig. 6 Rheological curve of formulation showing the pseudoplastic behavior of the sample. | |
Many drugs used to manage skeletal muscle injuries have showed side effects and have not focused on oxidative stress pathways.37,38 Therefore, natural products have been investigated for reducing oxidative stress and improving muscle injuries. This study observed that quercetin/β-cyclodextrin inclusion complex was as effective as phonophoresis for reducing oxidative stress parameters. Due to its poor solubility and bioavailability, quercetin was complexed with β-cyclodextrin and incorporated in Carbopol gel for topical administration. The formulation showed suitable physicochemical characteristics with high percentage of quercetin inside β-CD cavity and rheological properties giving the gel excellent potential to be tested in an animal model. The reduction of oxidative stress markers by both quercetin and TPU has some implications.
Oxidative stress indicates a high level of ROS and a less efficient antioxidant defense system. It can damage proteins, lipids and DNA in the cells.39 Muscle trauma increases the levels of oxidative stress-related biomarkers. In this study, 98 hours after the experimentally induced muscle injury, the animals exhibited increased TBARS levels, SOD activity and CAT activity, as showed in Fig. 7–9. This biologic response confirms that the muscle injury was significantly induced as the oxidative stress increased. Reactive oxygen species generation can increase by 60% and lipid peroxidation is likely to be three times higher during muscle lesion.40 To overcome oxidative stress-related biomarkers elevation, treatments with antioxidant therapies are suggested.
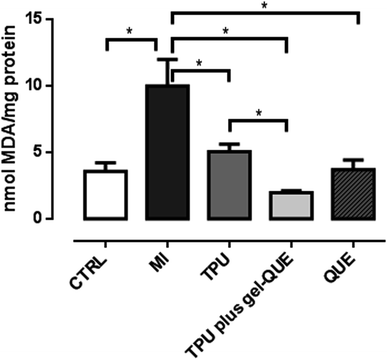 |
| Fig. 7 Lipid peroxidation levels (mean ± SD) 98 hours after muscle injury for each experimental group: CTRL, control; MI, muscle injury; TPU, therapeutic pulsed ultrasound; TPU plus gel-QUE, therapeutic pulsed ultrasound plus quercetin; and QUE, quercetin. *p < 0.05 compared to control. | |
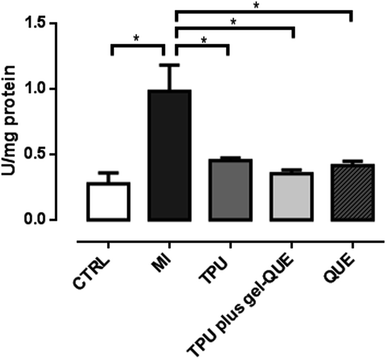 |
| Fig. 8 Superoxide dismutase activity (mean ± SD) 98 h after muscle injury for each experimental group: CTRL, control; MI, muscle injury; TPU, therapeutic pulsed ultrasound; TPU plus gel-QUE, therapeutic pulsed ultrasound plus quercetin; and QUE, quercetin. *p < 0.05 compared to control. | |
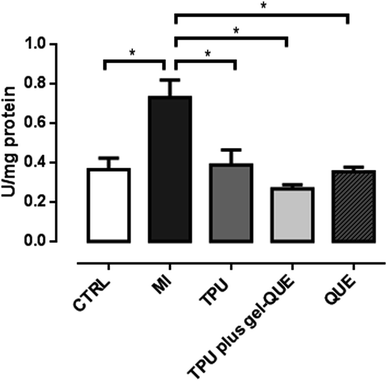 |
| Fig. 9 Catalase activity (mean ± SD) 98 h after muscle injury for each experimental group: CTRL, control; MI, muscle injury; TPU, therapeutic pulsed ultrasound; TPU plus gel-QUE, therapeutic pulsed ultrasound plus quercetin; and QUE, quercetin. *p < 0.05 compared to control. | |
QUE (3.6 ± 0.7 nmol MDA per mg protein, p < 0.001) reduced lipid peroxidation after MI (9.9 ± 2.0 nmol MDA per mg protein) through TBARS reduction, as showed in Fig. 7. The other treatment groups, TPU (5.04 ± 0.5 nmol MDA per mg protein p < 0.001) and phonophoresis using quercetin gel complexed in β-CD (TPU plus gel-QUE, 1.96 ± 0.15 nmol MDA per mg protein p < 0.001) also reduced TBARS compared to MI group (Fig. 7).
Lipid peroxidation following skeletal muscle injury damage cell membranes through changes in permeability and fluids pressure.41 In previous research, phonophoresis with dimethylsulfoxide (DMSO) gel attenuated lipid peroxidation levels in mechanically traumatized muscle.19 In the same experimental model, phonophoresis using gold nanoparticles reduced lipid peroxidation levels.4 The hydroxyl radical, which is increased in muscle injury, abstract hydrogen atoms oxidizing fatty acids and degrading the deoxyribose, which results in lipid membrane cell damage.42 Once installed, the lipid peroxidation process generates deleterious products as malondialdehyde (MDA).23 We suppose that the treatment groups with quercetin in this study acted by scavenging hydroxyl radicals, thus reducing indirectly reactive substances for thiobarbituric acid. Flavonoids plays an important function in scavenging free radicals or ROS.43 Previously, it was observed that quercetin inhibits deoxyribose degradation indicating its possible ability of scavenger hydroxyl radicals.44
Other pathway in the lipid oxidation process involves nitric oxide (NO). There is a relationship between NO and lipid peroxidation. Hydroxyl radicals, which induce lipid peroxidation, are formed by the Fenton reaction and NO acts by inhibiting this reaction, thus preventing hydroxyl radical formation.45 As therapeutic ultrasound has previously been shown to stimulate NO production,46 it is reasonable to believe that therapeutic ultrasound would reduce hydroxyl radical levels and then reduce lipid peroxidation markers such as TBARS, as showed in our results.
Although it has been suggested that some effects of ultrasound could be mediated by NO signaling, evidence to support this claim is still controversial.47–49 For example, whereas Wang (2004)49 found that NO was associated with angiogenesis after ultrasound stimulation, Chongsatientam and Yimlamai (2016)47 observed that therapeutic ultrasound promoted angiogenesis but that effect was not mediated by mRNA expression of NO synthase. The most common mechanism linked to the therapeutic ultrasound action involves the stimulation of the production of vascular endothelial growth factor (VEGF), which promotes angiogenesis by increasing capillary density and consequently facilitates tissue healing.47,48,50
Fig. 8 shows that QUE (0.41 ± 0.02 U per mg protein p < 0.01), TPU plus gel-QUE (0.34 ± 0.03 U per mg protein p < 0.01) and TPU (0.46 ± 0.02 U per mg protein p < 0.05) groups reduced significantly superoxide dismutase (SOD) activity compared to MI group (0.93 ± 0.2 U per mg protein). In the Fig. 9, QUE (0.36 ± 0.02 U per mg protein p < 0.05), TPU plus gel-QUE (0.26 ± 0.02 U per mg protein p < 0.05), and TPU (0.36 ± 0.07 U per mg protein p < 0.05) groups also reduced catalase (CAT) activity compared to MI group (0.78 ± 0.08 U per mg protein).
Superoxide dismutase and catalase are enzymes that work subsequently on the antioxidant reactions systems. According to our results, CAT and SOD activity was reduced 98 hours after injury induction by all treatment groups. Previous studies investigating skeletal muscle injury also observed a reduction on CAT and SOD activity.4,10,19 Two factors may be taken into account for these findings. Firstly, a significant reduction of antioxidant enzymes can indicate that the pro oxidative status is lower. When muscle injury is installed, the production of reactive oxygen species is increased, which promotes damage to the cells. In response to this increased production of ROS, during the first hours after lesion, the antioxidant enzymes become more active.51 With the treatments, the elevated generation of ROS is reduced and so the activity of the antioxidant enzymes is also decreased.4,10,19 Secondly, antioxidant mechanisms can be related to the inflammatory process of the muscle injury. Although we did not assess the inflammatory status, it is argued that ROS are involved on the promotion of inflammation by activating transcription factors such as NF-κB (nuclear factor-kappa B) and activator protein (AP)-1.13 The factor NF-κB is an inflammation mediator that can elevate SOD activity.13,52–54 As during inflammation phase NF-κB is usually active, it could possibly increase SOD activity, and consequently CAT activity. Considering that both therapeutic ultrasound and quercetin have well-established anti-inflammatory properties, it is reasonable to believe that these therapies could reduce inflammation and the activity of the antioxidant enzymes. The interplay between these antioxidant and anti-inflammatory mechanisms may be better elucidated.
The finding that quercetin/β-cyclodextrin transdermal gel attenuates oxidative stress irrespective of using TPU is especially relevant because this formulation could be a promising therapeutic option for skeletal muscle injuries. However, it is important to highlight that the effects of quercetin/β-cyclodextrin gel in this study were only observed in comparison to the muscle injury without treatment (no superior effect over the other treatments) and there was not a comparison between quercetin/β-cyclodextrin gel and isolated quercetin gel (without β-cyclodextrin). In this way, despite quercetin/β-cyclodextrin gel was as effective as phonophoresis, the results may be interpreted with caution. As skeletal muscle injuries present other associated signaling mechanisms such as inflammation and regeneration, future studies may consider to assess the effects of quercetin/β-cyclodextrin gel on inflammatory and histopathological markers.
Conclusions
In conclusion, our results show that quercetin/β-cyclodextrin transdermal gel was as effective as phonophoresis for reducing oxidative stress. Applied alone or combined, TPU and quercetin/β-cyclodextrin gel reduced lipid peroxidation levels and superoxide dismutase and catalase activity 98 hours after skeletal muscle injury.
Funding
This research did not receive any specific grant from funding agencies in the public, commercial, or not-for-profit sectors.
Author contributions
LFSF: conceptualization, methodology, investigation, writing – original draft MMBS: investigation, visualization, writing – original draft PPM: investigation, resources, writing – original draft BSL: investigation, resources AASA: resources, supervision EDO: conceptualization, writing – review & editing, resources, project administration.
Conflicts of interest
There are no conflicts to declare.
References
- P. Edouard, P. Branco and J. M. Alonso, Br. J. Sports Med., 2016, 50, 619–630 CrossRef PubMed.
- X. Liu, Z. Zeng, L. Zhao, W. Xiao and P. Chen, Exp. Ther. Med., 2018, 15, 2196–2202 CAS.
- H. Sies and E. Cadenas, Philos. Trans. R. Soc. London, Ser. B, 1985, 311, 617–631 CrossRef CAS PubMed.
- P. C. L. Silveira, E. G. Victor, F. D. S. Notoya, D. D. L. Scheffer, L. Silva, R. B. Cantú, V. H. Martinez, R. A. Pinho and M. M. Paula, Drug Delivery, 2016, 23, 926–932 CrossRef PubMed.
- C. D. Black, M. P. Herring, D. J. Hurley and P. J. O'Connor, J. Pain, 2010, 11, 894–903 CrossRef PubMed.
- L. M. Nicol, D. S. Rowlands, R. Fazakerly and J. Kellett, Eur. J. Appl. Physiol., 2015, 115, 1769–1777 CrossRef CAS PubMed.
- M. Rondanelli, A. Miccono, G. Peroni, F. Guerriero, P. Morazzoni, A. Riva, D. Guido and S. Perna, Evid.-Based Complementary Altern. Med., 2016, 2016, 5970367 CAS.
- P. P. Alfredo, C. A. Anaruma, A. C. S. Pião, S. M. A. João and R. A. Casarotto, Ultrasonics, 2009, 49, 466–471 CrossRef PubMed.
- A. C. Cabral de Oliveira, A. C. Perez, G. Merino, J. G. Prieto and A. I. Alvarez, Comp. Biochem. Physiol., Part C: Toxicol. Pharmacol., 2001, 130, 369–377 CAS.
- L. F. Sousa Filho, P. P. Menezes, D. V. S. Santana, B. S. Lima, S. Saravanan, G. K. M. Almeida, J. E. R. M. Filho, M. M. B. Santos, A. A. S. Araujo and E. D. Oliveira, Ultrasound Med. Biol., 2018, 44, 359–367 CrossRef PubMed.
- S. A. Klinge and G. A. Sawyer, Phys. Sportsmed., 2013, 41, 64–74 CrossRef PubMed.
- Z. Aytac, S. I. Kusku, E. Durgun and T. Uyar, Food Chem., 2016, 197, 864–871 CrossRef CAS PubMed.
- P. Zizkova, M. Stefek, L. Rackova, M. Prnova and L. Horakova, Chem. Biol. Interact., 2017, 265, 36–46 CrossRef CAS PubMed.
- M. E. M. B. Araújo, Y. Moreira Franco, T. Grando Alberto, M. Alves Sobreiro, M. A. Conrado, D. Gonçalves Priolli and P. Oliveira Carvalho, Food Chem., 2013, 141, 266–273 CrossRef PubMed.
- C. G. Heiknen, G. R. M. M. Haenen, R. M. Oostveen, E. M. Stalpers and A. Bast, Free Radic. Res., 2002, 36, 575–581 CrossRef PubMed.
- V. G. C. Abreu, G. M. Correa, T. M. Silva, H. S. Fontoura, D. C. Cara, D. Piló-Veloso and A. F. C. Alcântara, BMC Complementary Altern. Med., 2013, 13, 270 CrossRef PubMed.
- Y. L. Hsieh, Phys. Ther., 2006, 86, 39–49 CrossRef PubMed.
- A. L. M. Maia Filho, A. B. Villaverde, E. Munin, F. Aimbire and R. Albertini, Ultrasound Med. Biol., 2010, 36, 1682–1690 CrossRef PubMed.
- P. C. L. Silveira, L. A. da Silva, P. T. Camila Tromm, D. D. L. Scheffer, C. T. de Souza and R. A. Pinho, Ultrasonics, 2012, 52, 650–654 CrossRef CAS PubMed.
- V. Avasatthi, H. Pawar, C. P. Dora, P. Bansod, M. S. Gill and S. Suresh, Pharm. Dev. Technol., 2016, 21, 554–562 CrossRef CAS PubMed.
- C. F. Rizzi, J. L. Mauriz, D. S. F. Corrêa, A. J. Moreira, C. G. Zettler, L. I. Filippin, N. P. Marroni and J. Gonzalez Galego, Lasers Surg. Med., 2006, 38, 704–713 CrossRef PubMed.
- S. Saliba, D. J. Mistry, D. H. Perrin, J. Gieck and A. Weltman, J. Athl. Train., 2007, 42, 349–354 Search PubMed.
- H. H. Draper and M. Hadley, Methods Enzymol., 1990, 186, 421–431 CAS.
- J. Bannister and L. Calabrese, Methods Biochem. Anal., 1987, 32, 279–312 CAS.
- H. Aebi, Methods Enzymol., 1984, 105, 121–126 CAS.
- J. L. Koontz, J. E. Marcy, S. F. O'Keefe and S. E. Duncan, J. Agric. Food Chem., 2009, 57, 1162–1171 CrossRef CAS PubMed.
- S. Olejniczak and M. J. Potrzebowski, Org. Biomol. Chem., 2004, 2, 2315–2322 RSC.
- K. V. Sri, A. Kondaiah, J. V. Ratna and A. Annapurna, Drug Dev. Ind. Pharm., 2007, 33, 245–253 CrossRef CAS PubMed.
- P. Passos Menezes, P. B. P. dos Santos, G. A. A. Doria, B. M. H. de Sousa, M. R. Serafini, P. S. Nunes, L. J. Quintas-Junior, L. I. de Matos, P. B. Alves, D. P. Bezerra, F. J. Mendonça Júnior, G. F. da Silva, T. M. de Aquino, E. Souza Bento, M. T. Scotti, L. Scotti and A. A. de Souza, AAPS PharmSciTech, 2017, 18, 49–57 CrossRef PubMed.
- L. Djekic, D. Krajisnik, M. Martinovic, D. Djordjevic and M. Primorac, Int. J. Pharm., 2015, 490, 180–189 CrossRef CAS PubMed.
- D. Chantasart, S. Chootanasoontorn, J. Suksiriworapong and S. K. Li, J. Pharm. Sci., 2015, 104, 3459–3470 CrossRef CAS PubMed.
- K. V. Nikumbh, S. G. Sevankar and M. P. Patil, Drug Delivery, 2015, 22, 509–515 CrossRef CAS PubMed.
- M. Pandey, V. Belgamwar, S. Gattani, S. Surana and A. Tekade, Drug Delivery, 2010, 17, 38–47 CrossRef CAS PubMed.
- A. Gupta, A. K. Mishra, A. K. Singh, V. Gupta and P. Bansal, Drug Invent. Today, 2010, 2, 250–253 CAS.
- E. Di Giuseppe, F. Corbi, F. Funiciello, A. Massmeyer, T. N. Santimano, M. Rosenau and A. Davaile, Tectonophysics, 2015, 642, 29–45 CrossRef CAS.
- F. M. Mady, H. Essa, T. El-Ammawi, H. Abdelkader and A. K. Hussein, Drug Des., Dev. Ther., 2016, 10, 1101–1110 CAS.
- C. H. Liao, L. P. Lin, T. Y. Yu, C. C. Hsu, J. S. Pang and W. C. Tsai, Skeletal Muscle, 2019, 9, 23 CrossRef PubMed.
- K. M. Morelli, L. B. Brown and G. L. Warren, Am. J. Sports Med., 2018, 46, 224–233 CrossRef PubMed.
- M. Kozakowska, K. Pietraszek-Gremplewicz, A. Jozkowicz and J. Dulak, J. Muscle Res. Cell Motil., 2015, 36, 377–393 CrossRef CAS PubMed.
- C. N. Martins, M. B. Moraes, M. Hauck, L. F. Guerreiro, D. D. Rossato, A. S. Varela, C. E. Rosa and S. U. Signori, Physiotherapy, 2016, 102, 377–383 CrossRef CAS PubMed.
- T. Zhou, E. R. Prather, D. E. Garrison and L. Zuo, Int. J. Mol. Sci., 2018, 19, 417 CrossRef PubMed.
- S. Luqman and R. Kumar, Int. J. Food Prop., 2012, 15, 942–948 CrossRef CAS.
- J. Treml and K. Smejkal, Compr. Rev. Food Sci. Food Saf., 2016, 15, 720–738 CrossRef CAS PubMed.
- J. M. D. Markovic, Z. S. Markovic, I. A. Pasti, T. P. Brdaric, A. Popovic-Bijelic and M. Mojovic, Dalton Trans., 2012, 41, 7295–7303 RSC.
- D. A. Wink, K. M. Miranda, M. G. Espey, R. M. Pluta, S. J. Hewett, C. Colton, M. Vitek, M. Feelisch and M. B. Grisham, Antioxid. Redox Signaling, 2001, 3, 203–213 CrossRef CAS PubMed.
- J. T. Sutton, J. L. Raymond, M. C. Verleye, G. J. Pyne-Geithman and C. K. Holland, Int. J. Nanomed., 2014, 9, 4671–4683 CrossRef CAS PubMed.
- A. Chongsatientam and T. Yimlamai, Ultrasound Med. Biol., 2016, 42, 2938–2949 CrossRef PubMed.
- P. Reher, M. Harris, M. Whiteman, H. K. Hai and S. Meghji, Bone, 2002, 31, 236–241 CrossRef CAS PubMed.
- F. S. Wang, Y. R. Kuo, C. J. Wang, K. D. Yang, P. R. Chang, Y. T. Huang, H. C. Huang, Y. C. Sun, Y. J. Yang and Y. J. Chen, Bone, 2004, 35, 114–123 CrossRef CAS PubMed.
- P. Reher, N. Doan, B. Bradnock, S. Meghji and M. Harris, Cytokine, 1999, 11, 416–423 CrossRef CAS PubMed.
- G. Bosco, Z. J. Yang, J. Nandi, J. Wang, C. Chen and E. M. Camporesi, Clin. Exp. Pharmacol. Physiol., 2007, 34, 70–76 CrossRef CAS PubMed.
- M. Djavaheri-Mergny, D. Javelaud, J. Wietzerbin and F. Besançon, FEBS Lett., 2004, 578, 111–115 CrossRef CAS PubMed.
- J. Hollander, R. Fiebig, M. Gore, T. Ookawara, H. Ohno and L. L. Ji, Pflugers Arch., 2001, 442, 426–434 CrossRef CAS PubMed.
- M. Kairisalo, L. Korhonen, K. Blomgren and D. Lindholm, Biochem. Biophys. Res. Commun., 2007, 364, 138–144 CrossRef CAS PubMed.
|
This journal is © The Royal Society of Chemistry 2021 |
Click here to see how this site uses Cookies. View our privacy policy here.