DOI:
10.1039/D1RA04384F
(Paper)
RSC Adv., 2021,
11, 24949-24957
Development of a cyan blue-emitting Ba3La2(BO3)4:Ce3+,Tb3+ phosphor for use in dental glazing materials: color tunable emission and energy transfer†
Received
7th June 2021
, Accepted 8th July 2021
First published on 16th July 2021
Abstract
The Ce3+/Tb3+ doped Ba3La2(BO3)4 phosphors were synthesized by a conventional solid state reaction method. The synthesized phosphor samples are a single phase of Ba3La2(BO3)4 and showed angular-shaped fine grains with average particle size from 5 μm to 10 μm. The Ba3La2(BO3)4:Ce3+ phosphors showed an asymmetric broad blue emission under excitation at 365 nm and the Ba3La2(BO3)4:Tb3+ phosphors exhibited typical green emission assigned to the 4f–4f transition of Tb3+ under excitation at 254 nm. Under near-UV (365 nm) excitation, Ba3La2(BO3)4:Ce3+,Tb3+ phosphors showed both a blue emission band and green emission peaks due to Ce3+ and Tb3+, respectively. By optimizing the composition, cyan-blue emission with high color purity (CIE chromaticity coordinate values x = 0.2557 and y = 0.3839) was obtained for the Ba3La2(BO3)4:0.05Ce3+,0.03Tb3+ phosphor, and the internal quantum efficiency of the phosphor at the excitation wavelength of 365 nm is estimated to be 50%. The dental glazing paste prepared by mixing organic binder, Ba3La2(BO3)4:Ce3+,Tb3+ phosphors, and low Tg glass was successfully vitrified when it was heated at 600 and 700 °C, and showed high chemical stability of the luminescence properties in acidic aqueous solution (pH = 4).
Introduction
Phosphors have been widely applied in displays and lamps and are significantly important materials in our daily life. In particular, rare earth ion doped phosphors have been widely studied for application in a variety of luminescent materials such as general solid state lighting, display devices, electroluminescent devices, optical information storage, X-ray detectors, and TV monitors due to their excellent photoluminescence properties.1–3 Among these phosphor applications, phosphor-converted light-emitting diodes (pc-LEDs) have great potential in the field of high performance solid-state lighting system such as flashlights, indicating lights, and automobile headlights because of their lower power consumption, long life, high color rending index and conversion efficiency.4–6
Recently, many investigations have been devoted to the search for new application fields for phosphor materials, such as plant growth,7–11 optical sensors,12–17 cosmetics18,19 anti-counterfeiting20 and road signs/lanes.21,22 In addition, the phosphors have been used in the dental field to enhance the aesthetics of artificial crowns and restoration.21–29 Under UV-light irradiation, human natural teeth emit cyan blue color with peak at 450 nm and the photoluminescence (PL) properties of teeth make whiter and brighter for human natural teeth in daylight.30 The artificial crowns and restorative materials can match the shade of the natural teeth to satisfy aesthetic requirements by adding phosphors or pigments.25,26,31 The studies to develop the paste containing phosphor powder and glass frit, which called the dental glazing, are recently conducted to improve the aesthetics of artificial crowns and restoration.
In the present study, therefore, we have focused on the Ce3+ and Tb3+ co-doped phosphors to realize a development of the dental glazing paste in accordance with aesthetic requirements for artificial teeth. It is well-known that the Tb3+ ion-doped phosphors show the sharp green emission due to the 4f–4f transition of Tb3+ under UV excitation and the Ce3+ ion-doped phosphors show the broad blue emission due to the 5d–4f transition of Ce3+ under UV excitation.32–37 The PL properties of the Ce3+ and Tb3+ co-doped phosphors have been investigated by several researchers.38–45 Although Tb3+ ion-doped phosphors have a weak optical absorption in near-UV region, the Ce3+ and Tb3+ co-doped phosphors show a strong green emission under excitation which is considered to be due to the energy transfer from Ce3+ to Tb3+. These phosphors exhibit a color tunable emission by changing the concentration of Ce3+ and Tb3+ ions in the host lattice. These results indicate that the Ce3+ and Tb3+ co-doped phosphors can be expected as one of the suitable candidate for the cyan blue emission phosphor to achieve aesthetic requirements for teeth application.
In this study, using Ce3+ and Tb3+ ions as activators, cyan blue emitting Ba3La2(BO3)4:Ce3+,Tb3+ phosphors were synthesized by a conventional solid state reaction and the photoluminescence properties were characterized. The dental glazing pastes were prepared by mixing the as-prepared Tb3+/Ce3+ co-doped phosphor powders, an organic binder and a low Tg glass frits with a composition of (K,Na)2O–B2O3–Al2O3–SiO2. The photoluminescence properties and chemical stability against acid aqueous solution of the dental glazing pastes were investigated.
Experimental
Ce3+/Tb3+ doped Ba3La2(BO3)4 phosphors were synthesized by a conventional solid state reaction method. BaCO3 (Junsei Chemical Co., Ltd., 99%), La2O3 (Daejung Chemical & Metal Co., Ltd., 99.9%), B2O3 (Junsei, >95%), CeO2 (LTS, 99.98%), Tb4O7 (LTS, 99.95%) were used as starting materials with no further purification. In Ce3+/Tb3+ doped Ba3La2(BO3)4 phosphor, the amount of Ce3+ was adjusted between 1 to 15 mol% and the Tb3+ content was varied from 1 to 15 mol%. These raw materials were mixed in a stoichiometric ratio using an agate mortar for 5 times, and then the mixture was calcined at 800 °C for 6 h in a flow of 5% H2–95% N2 gas to reduce Ce4+ to Ce3+ and Tb4+ to Tb3+. After the calcination, the samples were reground in a mortar and heated again at 1200 °C for 6 h in a flow of 5% H2–95% N2 gas.
To prepare the dental glazing paste, the as-prepared Tb3+/Ce3+ co-doped phosphor powders mixed with an organic binder and a low Tg glass with a composition of (K,Na)2O–B2O3–Al2O3–SiO2. Then the homogeneous mixture was mixed and aged using wet ball milling process at room temperature for 24 h. The as-prepared dental glazing pastes coated on alumina plate using brushing method and then heated at 300–700 °C for 0.5 h in air atmosphere.
The crystal structure of the resultant powder samples was identified using X-ray powder diffraction (XRD, Bruker D8 advance), and the detailed crystallographic data were obtained by Rietveld analysis using the RIETAN-FP package.46 To determine the valence state of Ce and Tb ion in the Ba3La2(BO3)4:Ce3+,Tb3+ phosphor, X-ray photoelectron spectroscopy (XPS; JEOL, JPS-9000) was measured at room temperature. The morphology of the samples was characterized using scanning electron microscope (JEOL, JSM6700F). The photoluminescence emission (PL) and excitation (PLE) spectra were measured at room temperature using a fluorescence spectrophotometer (PSI, DARSA PRO 3400). PL spectra of Ce3+/Tb3+ doped Ba3La2(BO3)4 phosphor were obtained for excitation at 365 nm, and PLE spectra were recorded for emission at 450 and 543 nm. The internal quantum yield was measured using a spectrophotometer (PSI, DARSA PRO 3400) with a fluorescence integrating sphere unit (PSI, DARSA PRO 3400) at excitation wavelength of 365 nm. The photoluminescence properties of dental glazing paste were also characterized using a fluorescence spectrophotometer (PSI, DARSA PRO 3400). The time-resolved photoluminescence (TR-PL) was carried out using a confocal microscope (MicroTime-200, Picoquant, Germany). A single-mode pulsed diode laser (375 nm with 30 ps pulse width and average power of ∼1 μW operating in 1 MHz repetition rate) was used as an excitation source. To evaluate the chemical stability of the photoluminescence properties for the dental glazing paste, the acid resistance was investigated with HCl aqueous solution (pH = 4).
Results and discussion
Crystal structure analysis of Ce3+/Tb3+ doped Ba3La2(BO3)4 phosphors
Crystallographic data and structure refinement parameters of the Rietveld refinement of the XRD patterns of the Ce3+/Tb3+ doped Ba3La2(BO3)4 phosphor are summarized in Table 1, and the representative XRD pattern of the Ba3La2(BO3)4 host material is shown in Fig. 1. The representative XRD patterns of the Ce3+/Tb3+ doped Ba3La2(BO3)4 phosphors are shown in Fig. S1–S3† and the refined structural parameters of the Ba3La2(BO3)4 host material and Ce3+/Tb3+ doped Ba3La2(BO3)4 phosphors are summarized in Tables S1–S4.† The data of the orthorhombic structure Ba3La2(BO3)4 from the inorganic crystal structure database (ICSD no. 98-003-9277) were used for the starting model. As shown in Table 1, the final R-factor values, Rwp, Rp, and S, converged to 10.99, 8.10, and 2.36, respectively, which verify the phase purity of the as-prepared sample. The representative XRD pattern of Ba3La2(BO3)4 was well indexed to the reported data and no impurity phases were detected in the XRD pattern. The final refined results confirm a single phase of the Ba3La2(BO3)4 that is crystallized in a orthorhombic structure in space group Pnma (no. 62) with refined lattice parameters a = 0.77735(4) nm, b = 1.70989(1) nm, c = 0.90422(3) nm, and V = 1.20187 nm3. A schematic of the Ba3La2(BO3)4 crystal structure is shown in Fig. 2, produced using VESTA.47 According to the crystal structure analysis, Ba2+ and La3+ ions occupied in three different dodecahedral sites (8d and 4c) and there are three kinds of boron sites (8d and 4c) with 3-fold coordination. The lattice volume of the Ba3La2(BO3)4 phase decreased with doping the Ce3+ and Tb3+ in the crystal lattice, which indicates that the small size of Ce3+ (ionic radius: 0.1143 nm for 8 coordination48) and Tb3+ (ionic radius: 0.1040 nm for 8 coordination48) are successfully substituted into the larger La3+ ions sites (ionic radius: 0.1160 nm for 8 coordination48). Fig. 3 shows the XRD patterns of Ba3La2(BO3)4:Ce3+, Ba3La2(BO3)4:Tb3+, Ba3La2(BO3)4:Ce3+,Tb3+ phosphor samples with different concentrations. The XRD patterns of all samples were in good agreement with a single phase of highly crystalline orthorhombic structure (ICSD no. 98-003-9277) with space group Pnma (#62). With increasing the Ce3+ and Tb3+ ion contents in the Ce3+/Tb3+ doped Ba3La2(BO3)4 phosphors, a peak shift to a higher diffraction angle is observed because La3+ in the host material is partially substituted with the smaller Ce3+ and Tb3+ ions to form solid solutions. To investigate the valence state of Ce and Tb ions, binding energy of electrons in Ba3La2(BO3)4:Ce3+,Tb3+ phosphors was measured using XPS and the result is shown in Fig. S4.† The Ce 3d and Tb 3d XPS spectra are well known to split into two peaks of 3d3/2 and 3d5/2 due to the spin–orbital interaction. The XPS spectra of both Ce 3d and Tb 3d state exhibit two clear peaks at 889.9 eV (3d3/2) and 901.9 eV (3d5/2), and at 1242.8 eV (3d3/2) and 1275.9 eV (3d5/2), respectively, which can be assigned to those of 3+ oxidation state of Ce and Tb ions. In addition, no peaks that would suggest the presence of other oxidation states of Ce and Tb ions were observed. These results support that the Ce and Tb ions successfully substituted in the La3+ sites as Ce3+ and Tb3+ in the Ba3La2(BO3)4 host lattice. The morphologies of the Ce3+/Tb3+ doped Ba3La2(BO3)4 phosphors were observed by SEM as seen in Fig. 4. The particles of all powder samples were composed of angular-shape fine grain with the average size of around 5–10 μm.
Table 1 Crystallographic data of Ba3La2(BO3)4 and Ce3+/Tb3+ doped Ba3La2(BO3)4 phosphors
Formula |
Ba3La2(BO3)4 |
Ba3(La,Ce)2(BO3)4 |
Ba3(La,Tb)2(BO3)4 |
Ba3(La,Ce,Tb)2(BO3)4 |
Crystal system |
Orthorhombic |
Orthorhombic |
Orthorhombic |
Orthorhombic |
Space group |
Pnma |
Pnma |
Pnma |
Pnma |
a (nm) |
0.77735(4) |
0.77692(5) |
0.77708(2) |
0.77610(2) |
b (nm) |
1.70989(1) |
1.71059(12) |
1.70686(5) |
1.70481(4) |
c (nm) |
0.90422(3) |
0.90271(4) |
0.90220(2) |
0.90225(1) |
Rwp (%) |
10.99 |
11.36 |
10.00 |
10.64 |
Rp (%) |
8.10 |
8.40 |
7.31 |
7.84 |
S (%) |
2.36 |
2.65 |
2.57 |
3.08 |
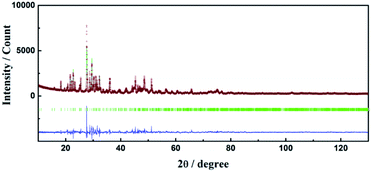 |
| Fig. 1 Rietveld refinement result for X-ray powder diffraction data of Ba3La2(BO3)4. Red symbol: measured pattern, green solid line: calculated pattern, blue solid line: difference between their intensities. | |
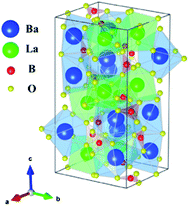 |
| Fig. 2 A schematic of the Ba3La2(BO3)4 crystal structure. | |
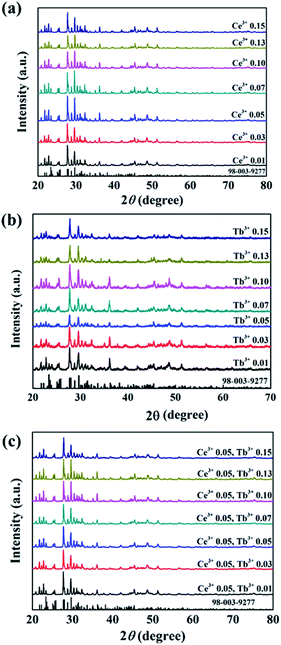 |
| Fig. 3 XRD patterns of (a) Ba3La2(BO3)4:Ce3+, (b) Ba3La2(BO3)4: Tb3+, (c) Ba3La2(BO3)4:Ce3+,Tb3+ phosphors doped with different concentrations and Ba3La2(BO3)4 reference (ICSD 98-003-9277). | |
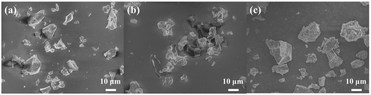 |
| Fig. 4 SEM images of (a) Ba3La2(BO3)4:Ce3+, (b) Ba3La2(BO3)4: Tb3+, (c) Ba3La2(BO3)4:Ce3+,Tb3+ phosphors. | |
Photoluminescence properties of Ce3+ doped Ba3La2(BO3)4 phosphor
The photoluminescence emission (PL) and excitation (PLE) spectra of Ba3La2(BO3)4:Ce3+ phosphors with different concentration of Ce3+ ions are presented in Fig. 5. The PLE spectra recorded by monitoring the emission at 470 nm exhibited a broad excitation band from 270 to 400 nm, which was attributed to 4f → 5d transition of Ce3+ ions. The PL spectra of Ba3La2(BO3)4:Ce3+ phosphors recorded under 365 nm excitation showed a broad non-symmetry blue emission band from 400 nm to 650 nm with a maximum peak at 470 nm due to 5d → 4f transition of Ce3+ ions. The emission of Ce3+ usually includes two bands of the transitions of 5d-excited state to 2F7/2 and 2F5/2 states, and the emission spectra seem to consist of two of them in a single band. The emission peak wavelength of the Ce3+ 5d → 4f transition shifts to the longer wavelength (lower energy) direction with increasing Ce3+ concentration for the sample, which can be attributed to the change of the crystal field strength around Ce3+ in the host lattice. The crystal field strength increases with increasing the amount of Ce3+ substitution for La3+ in the Ba3La2(BO3)4 lattice because the average bond length of Ce3+–O2− becomes progressively shorter by lattice shrinkage.49 The emission peak intensity of Ba3La2(BO3)4:Ce3+ phosphors was increased with the Ce3+ concentration up to 0.05 mol%, and then decreased probably due to the concentration quenching effect. The internal quantum yield of the 0.05 mol% Ce3+ doped Ba3La2(BO3)4 phosphor at the excitation wavelength of 365 nm was estimated to be 50%. Thus, the optimum concentration of Ce3+ ions was confirmed to be 0.05, and it was kept as a constant value to prepare Ce3+/Tb3+ co-doped Ba3La2(BO3)4 phosphors.
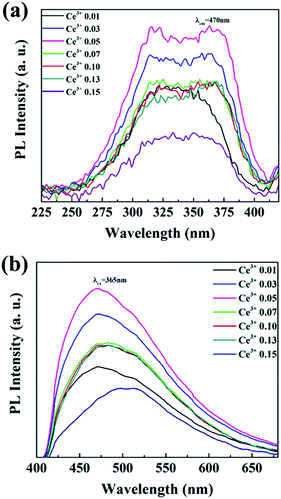 |
| Fig. 5 The (a) excitation (λem = 470 nm) and (b) emission (λex = 365 nm) and spectra of Ba3La2(BO3)4:Ce3+ phosphors with different concentrations of Ce3+. | |
Photoluminescence properties of Tb3+ doped Ba3La2(BO3)4 phosphor
Fig. 6 shows the PL and PLE spectra of Ba3La2(BO3)4:Tb3+ phosphors with different concentration of Tb3+ ions. The PLE spectra recorded by monitoring the emission at 543 nm, there are a broad band from 225 to 300 nm, corresponding to the spin-allowed energy transition from the 4f8 to 4f75d configuration of Tb3+. A number of small absorption peaks at 300–400 nm correspond to the spin-forbidden 4f–4f transition (7F6 → 5L1,5,10, 7F6 → 5D3). Under 254 nm excitation, the Ba3La2(BO3)4:Tb3+ phosphors exhibited a well-known characteristic Tb3+ green emission, and no self-activated emission was observed in the undoped samples. The emission peaks of Ba3La2(BO3)4:Tb3+ phosphors at 490, 547, 585, and 623 nm correspond to the 5D4 → 7F6, 5D4 → 7F5, 5D4 → 7F4, and 5D4 → 7F3 transitions of Tb3+ ions, respectively.50–52 The emission intensity of Ba3La2(BO3)4:Tb3+ phosphors was increased with increasing the Tb3+ concentration up to 0.10, and then decreased due to the concentration quenching effect. In particular, the emission intensity of 0.15 showed a dramatically decrease. The concentration quenching of luminescence is generally observed in phosphor materials in which a large amount of luminescence ion is doped into the host lattice.53,54 By increasing the luminescence ion concentration in host lattice, the distance between two adjacent luminescence ions is decreased and the interaction between the luminescence ions is enhanced, which usually results in the concentration quenching due to the nonradiative energy transfer between two luminescence ions.53,54 The internal quantum yield of the 0.10 mol% Tb3+ doped Ba3La2(BO3)4 phosphor at the excitation wavelength of 365 nm was estimated to be 60%.
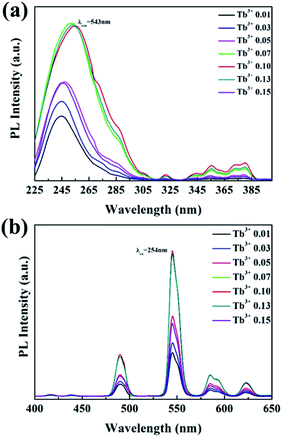 |
| Fig. 6 The (a) excitation (λem = 543 nm) and (b) emission (λex = 254 nm) spectra of Ba3La2(BO3)4 phosphors doped with different concentrations of Tb3+. | |
Photoluminescence properties of Ce3+ and Tb3+ doped Ba3La2(BO3)4 phosphor
To develop the cyan blue-emitting phosphors with similar to that of human teeth, Ce3+ and Tb3+ co-activated Ba3La2(BO3)4 phosphors synthesized and their optical properties were characterized. In Ce3+/Tb3+ co-activated Ba3La2(BO3)4 phosphors, the concentration of Ce3+ was fixed at the optimal value of 0.05 mol% and the concentration of Tb3+ was varied in the range of 0.01–0.15 mol%. The PLE and PL spectra of Ce3+/Tb3+ co-activated Ba3La2(BO3)4 phosphors are shown in Fig. 7. The PLE spectra monitored at 547 nm (Tb3+ 5D4–7F5 transition emission) consists of two strong broad bands with peaks at about 247 nm and 330 nm due to the 4f8 → 4f75d transition of Tb3+ and 4f1 → 4f05d transition of Ce3+, respectively. These PLE spectra are evidence of energy transfer from Ce3+ to Tb3+ in Ba3La2(BO3)4:Ce3+,Tb3+ phosphors. The intensities of the optical absorption bands due to 4f8 → 4f75d transition of Tb3+ and 4f1 → 4f05d transition of Ce3+ were effectively enhanced by increasing the Tb3+ ion contents in Ba3La2(BO3)4:Ce3+,Tb3+ phosphors. Under the excitation of 365 nm, the emission spectra exhibit a broad band of Ce3+ ions in the blue light region and a series of strong emission lines at 490, 545, 585, and 623 nm due to the 5D4–7FJ (J = 6, 5, 4, and 3) transitions of Tb3+ ions in which the green emission line at 542 nm from 5D4–7F5 transitions dominate. Although the emission spectra of the phosphors were recorded for excitation at 365 nm (optical absorption due to Ce3+), the Ce3+ emission intensity increased with increasing the concentration of Tb3+ in Ba3La2(BO3)4:Ce3+,Tb3+ phosphors, whereas Tb3+ emission effectively enhanced. The energy-transfer efficiency of Ce3+ → Tb3+ in Ba3La2(BO3)4:Ce3+,Tb3+ phosphors increased remarkably with increasing the Tb3+ contents. These results are evidence of energy transfer from Ce3+ to Tb3+ in the Ba3La2(BO3)4:Ce3+,Tb3+ phosphors. As shown in Fig. 7 inset, it can be found that color-tunable blue-to-green emission can be obtained with increasing Tb3+ content due to Ce3+ → Tb3+ energy transfer. Additionally, the CIE coordinates for the Ba3La2(BO3)4:Ce3+,Tb3+ phosphors could be changed from the (x = 0.2368, y = 0.3388; blue) to (x = 0.3006, y = 0.4914; green) by controlling the different emission compositions of the Ce3+ and Tb3+ contents. Among the different amount of Ce3+/Tb3+ co-doped Ba3La2(BO3)4 phosphors, the emission color of the Ba3La2(BO3)4:0.05Ce3+,0.03Tb3+ phosphor is very close to cyan light of commercial materials for use in dental glassing.
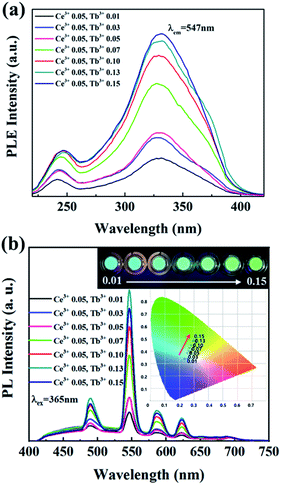 |
| Fig. 7 The (a) excitation (λem = 547 nm) and (b) emission (λex = 365 nm) spectra of Ba3La2(BO3)4:Ce3+,Tb3+ phosphors with different concentrations of Tb3+. | |
To determine the energy transfer from Ce3+ to Tb3+ in Ba3La2(BO3)4:Ce3+,Tb3+ phosphors, the luminescence lifetime of the phosphors were recorded by confocal microscope. Fig. 8 illustrates the decay curves of Ba3La2(BO3)4:0.05Ce3+ and Ba3La2(BO3)4:0.05Ce3+,0.13Tb3+ phosphors monitored at 470 nm for Ce3+ emission. The decline of lifetime further demonstrated the presence of energy transfer from Ce3+ to Tb3+. Additionally, efficiency of energy transfer (ηET) between Ce3+ and Tb3+ can be calculated by following equation:55
where
τ and
τ0 represent fluorescence lifetime of phosphors with and without Tb
3+ doping. The average lifetime of Ba
3La
2(BO
3)
4:0.05Ce
3+,0.13Tb
3+ phosphors with and without Tb
3+ doping can be calculated as 14 ns and 11 ns, respectively. It obviously shows that the average lifetime of Ce
3+ ions decreases with Tb
3+ ions doping. The efficiency of energy transfer from Ce
3+ to Tb
3+ in the Ba
3La
2(BO
3)
4:0.05Ce
3+,0.13Tb
3+ phosphors was estimated to be approximately 21.5%.
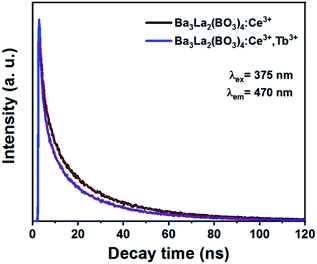 |
| Fig. 8 The decay curves of Ba3La2(BO3)4:0.05Ce3+ and Ba3La2(BO3)4:0.05Ce3+,0.13Tb3+ phosphors. | |
Characterization of dental glazing paste using Ce3+/Tb3+ co-doped Ba3La2(BO3)4 phosphor
The dental glazing paste using the Ce3+/Tb3+ co-doped Ba3La2(BO3)4 phosphor was prepared by mixing with an organic binder and a low Tg glass with a composition of (K,Na)2O–B2O3–Al2O3–SiO2. The as-prepared dental glazing pastes coated on alumina plate using brushing method and then heated at 300–700 °C for 0.5 h in air atmosphere. Fig. 9 shows the photograph of dental glazing pastes coated on alumina plate and the PL spectra of the dental glazing pastes heated at 300–700 °C for 0.5 h. The pastes heated at 300–500 °C was observed no vitrification, whereas, in the case of the pastes heated at 600 and 700 °C, vitrification was successfully observed. The emission peak intensity of the dental glazing pastes decreases with increasing the heating temperature (Fig. 9(a)). The reduction of the emission intensity of the Ce3+ or Eu2+ doped phosphors owing to re-heating is well known in phosphor-in-glass (PiG) application.56–62 The reduction of the emission intensity is well known to be due to the oxidation of Ce3+ into Ce4+ (or Eu2+ into Eu3+) in the phosphors, and the oxidation of luminescence ions usually results in the thermal degradation of the photoluminescence efficiency of the phosphors.63,64
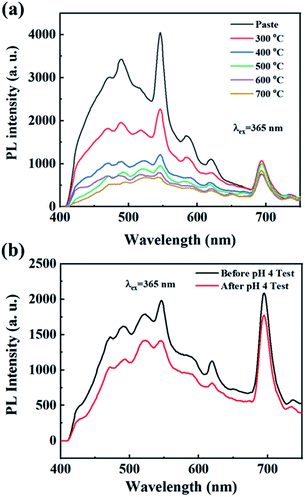 |
| Fig. 9 (a) The photograph of dental glazing pastes coated on alumina plate and the PL spectra of the dental glazing pastes heated at 300–700 °C for 0.5 h, (b) the emission spectra of the dental glazing paste before and after pH 4 test. | |
To evaluate the chemical stability of the photoluminescence efficiency of the as-prepared dental glazing pastes using the Ce3+/Tb3+ co-doped Ba3La2(BO3)4 phosphor, the acid resistance of the as-prepared dental glazing pastes was carried out in HCl aqueous solution (pH = 4). The pH values of drink such as an orange juice, coke, ade and etc. is about 3–4. The dental glazing pastes coated on alumina plate, which prepared by heating at 600 °C, was treated with HCl aqueous solution and soaked for 48 h, and then washed with water and dried at 80 °C for 24 h. After the HCl aqueous solution treatment for 48 h, which is very hard condition, the emission intensity of the dental glazing paste was decreased to 85% of the non-treatment sample as shown in Fig. 9(b). This result evidences that the dental glazing paste possesses high resistance to the acid solution.
Conclusions
In this study, we demonstrated the possibility of the Ba3La2(BO3)4:Ce3+,Tb3+ phosphors for use in dental glazing paste. The Ba3La2(BO3)4:Ce3+,Tb3+ phosphors were synthesized in a single phase form by a conventional solid state reaction method. The Ba3La2(BO3)4:Ce3+ phosphors showed a asymmetric broad blue emission with maximum peak at 470 nm under excitation at 365 nm due to the 5d → 4f transition of Ce3+, and the maximum emission peak intensity was obtained for the Ba3La2(BO3)4:0.05Ce3+. The Ba3La2(BO3)4:Tb3+ phosphors exhibited typical green emission assigned to the 4f–4f transition of Tb3+ under excitation at 254 nm, and the highest green emission intensity was obtained for the Ba3La2(BO3)4:0.10Tb3+. Under near-UV (365 nm) excitation, Ba3La2(BO3)4:Ce3+,Tb3+ phosphors showed both blue emission band and green emission peaks due to the Ce3+ and Tb3+, respectively, and the emission color of the phosphors can be tuned from blue to green by changing Ce3+/Tb3+ ratio. By optimization of the composition, cyan-blue emission with high color purity was obtained for the Ba3La2(BO3)4:0.05Ce3+,0.03Tb3+ phosphor, and the CIE chromaticity coordinate values for the phosphor were x = 0.2557 and y = 0.3839 under excitation at 365 nm, which is close to the cyan-blue emission for natural teeth. The internal quantum efficiency of the phosphor at the excitation wavelength of 365 nm is estimated to be 50%. To prepare the dental glazing paste, the Ce3+/Tb3+ co-doped Ba3La2(BO3)4 phosphor powders mixed with an organic binder and a low Tg glass with a composition of (K,Na)2O–B2O3–Al2O3–SiO2. For the samples heated at 600 and 700 °C, the dental glazing paste showed clear vitrification and they showed a high chemical stability of the luminescence properties for acid aqueous solution (pH = 4). These results indicate that the Ce3+/Tb3+ co-doped Ba3La2(BO3)4 phosphor are a suitable candidate as a luminescent material to produce the dental glazing paste with a high aesthetic.
Conflicts of interest
There are no conflicts to declare.
Acknowledgements
This work was supported by the Technology Development Program (S2800580) funded by the Ministry of SMEs and Startups (MSS, Korea).
Notes and references
- N. Avci, J. Musschoot, P. Smet, K. Korthout, A. Avci, C. Detavernier and D. Poelman, Microencapsulation of moisture-sensitive CaS: Eu2+ particles with aluminum oxide, J. Electrochem. Soc., 2009, 156, J333 CrossRef CAS.
- V. Gapontsev, S. Matitsin, A. Isineev and V. Kravchenko, Erbium glass lasers and their applications, Opt. Laser Technol., 1982, 14, 189 CrossRef CAS.
- K. Hirao, S. Todoroki, D. Cho and N. Soga, Room-temperature persistent hole burning of Sm2+ in oxide glasses, Opt. Lett., 1993, 18, 1586 CrossRef CAS PubMed.
- S. Ye, F. Xiao, Y. Pan, Y. Ma and Q. Zhang, Phosphors in phosphor-converted white light-emitting diodes: Recent advances in materials, techniques and properties, Mater. Sci. Eng., R, 2010, 71, 1 CrossRef.
- S. Pimputkar, J. S. Speck, S. P. DenBaars and S. Nakamura, Prospects for LED lighting, Nat. Photonics, 2009, 3, 180 CrossRef CAS.
- Y. H. Kim, N. S. Viswanath, S. Unithrattil, H. J. Kim and W. B. Im, phosphor plates for high-power LED applications: challenges and opportunities toward perfect lighting, ECS J. Solid State Sci. Technol., 2017, 7, R3134 CrossRef.
- Z. Zhou, M. Xia, Y. Zhong, S. Gai, S. Huang, Y. Tian, X. Lu and N. Zhou, Dy3+@Mn4+ co-doped Ca14Ga10−mAlmZn6O35 far-red emitting phosphors with high brightness and improved luminescence and energy transfer properties for plant growth LED lights, J. Mater. Chem. C, 2017, 5, 8201 RSC.
- Y. Zheng, H. Zhang, Z. Xia, Y. Liu, M. Molokeev and B. Lei, Co-substitution of Y3+/Mg2+ for Ca2+/Al3+ in CaAl12O19:Mn4+ phosphors: local structure evolution, photoluminescence tuning and application for plant growth LEDs, J. Mater. Chem. C, 2018, 6, 4217 RSC.
- X. Yang, Y. Zhang, X. Zhang, J. Chen, H. Huang, D. Wang, X. Chai, G. Xie, M. S. Molokeev and H. Zhang, Facile synthesis of the desired red phosphor Li2Ca2Mg2Si2N6:Eu2+ for high CRI white LEDs and plant growth LED device, J. Am. Ceram. Soc., 2020, 103, 1773 CrossRef CAS.
- M. Rajendran and S. Vaidyanathan, Zero-concentration quenching: a novel Eu3+ based red phosphor with non-layered crystal structure for white LEDs and NaSrY (MoO4)3:Sm3+ based deep-red LEDs for plant growth, Dalton Trans., 2020, 49, 9239 RSC.
- S. Gu, M. Xia, C. Zhou, Z. Kong, M. S. Molokeev, L. Liu, W.-Y. Wong and Z. Zhou, Red shift properties, crystal field theory and nephelauxetic effect on Mn4+-doped SrMgAl10-yGayO17 red phosphor for plant growth LED light, Chem. Eng. J., 2020, 396, 125208 CrossRef CAS.
- B. Yan, Y. Wei, W. Wang, M. Fu and G. Li, Red-tunable LuAG garnet phosphors via Eu3+→ Mn4+ energy transfer for optical thermometry sensor application, Inorg. Chem. Front., 2021, 8, 746 RSC.
- Y. Hu, F. Zhou, X. Tian, C. Ji, Z. Huang, J. Wen, F. Luo, Z. Chen, X. Liu and Y. Peng, CaSnO3: Pr3+ phosphor for new application in temperature sensing, Spectrochim. Acta, Part A, 2020, 243, 118799 CrossRef CAS PubMed.
- G. Xiang, X. Liu, Q. Xia, S. Jiang, X. Zhou, L. Li, Y. Jin, L. Ma, X. Wang and J. Zhang, Deep-Tissue Temperature Sensing Realized in BaY2O4:Yb3+/Er3+ with Ultrahigh Sensitivity and Extremely Intense Red Upconversion Luminescence, Inorg. Chem., 2020, 59, 11054 CrossRef CAS PubMed.
- G. Xiang, Q. Xia, X. Liu, Y. Wang, S. Jiang, L. Li, X. Zhou, L. Ma, X. Wang and J. Zhang, Upconversion nanoparticles modified by Cu2S for photothermal therapy along with real-time optical thermometry, Nanoscale, 2021, 13, 7161 RSC.
- H. Suo, X. Zhao, Z. Zhang, Y. Wu and C. Guo, Upconverting LuVO4:Nd3+/Yb3+/Er3+@SiO2@Cu2S Hollow Nanoplatforms for Self-monitored Photothermal Ablation, ACS Appl. Mater. Interfaces, 2018, 10, 39912 CrossRef CAS PubMed.
- H. Suo, X. Zhao, Z. Zhang and C. Guo, Ultra-sensitive optical nano-thermometer LaPO4:Yb3+/Nd3+ based on thermo-enhanced NIR-to-NIR emissions, Chem. Eng. J., 2020, 389, 124506 CrossRef CAS.
- A. Verma and A. Verma, Synthesis, characterization, mechano-luminescence, thermoluminescence, and antibacterial properties of SrMgAl10O17: Eu phosphor, J. Alloys Compd., 2019, 802, 394 CrossRef CAS.
- S. W. Kim, T. Hasegawa, M. Watanabe, K. Sugimoto, Y. Saito, K. Uematsu, K. Toda and M. Sato, Environmentally friendly Rb3V5O14 fluorescent red pigment, Dyes Pigm., 2017, 136, 219 CrossRef CAS.
- D. Jiang and C. N. Chong, 2nd International Conference on Anti-counterfeiting, Security and Identification, China, August, 2008 Search PubMed.
- B. Zhu, C. Song, Z. Guo, Y. Zhang and Z. Zhou, Effectiveness of Active Luminous Lane Markings on Highway at Night: A Driving Simulation Study, Sustainability, 2021, 13, 1043 CrossRef.
- J. I. Park, S. H. Jeong and I. W. Cheong, Microencapsulation of SrAl2O4:Eu2+, Dy3+ Phosphorescent Phosphor for Enhanced Visibility of Road Lanes, J. Adhes. Interface, 2016, 17, 110 CrossRef.
- I. Ahmad, Anterior dental aesthetics: Dental perspective, Br. Dent. J., 2005, 199, 135 CrossRef CAS PubMed.
- W. Buchalla, Comparative fluorescence spectroscopy shows differences in noncavitated enamel lesions, Caries Res., 2005, 39, 150 CrossRef CAS PubMed.
- P.-H. Chuang, Y.-J. Lai, C. C. Lin, T.-M. Wang, H. Yang, L.-D. Lin and R.-S. Liu, Facile dental resin composites with tunable fluorescence by tailoring Cd-free quantum dots, RSC Adv., 2013, 3, 16639 RSC.
- Y. K. Lee, Fluorescence properties of human teeth and dental calculus for clinical applications, J. Biomed. Opt., 2015, 20, 040901 CrossRef PubMed.
- A. A.-R. S. Marouf and Y. A. Khairallah, Photoemission Spectra of Sound Tooth and Those of Different Carious Stages, Eur. Biophys. J., 2019, 7, 23 CrossRef.
- D. Goloshchapov, P. Seredin, D. Minakov and E. Domashevskaya, Photoluminescence Properties of Nanoporous Nanocrystalline Carbonate-Substituted Hydroxyapatite, Opt. Spectrosc., 2018, 124, 187 CrossRef CAS.
- X. Li, C. Luo, Q. Fu, C. Zhou, M. Ruelas, Y. Wang, J. He, Y. Wang, Y. S. Zhang and J. Zhou, A Transparent, Wearable Fluorescent Mouthguard for High-Sensitive Visualization and Accurate Localization of Hidden Dental Lesion Sites, Adv. Mater., 2020, 32, 2000060 CrossRef CAS PubMed.
- H. Panzeri, L. T. Fernandes and C. J. Minelll, Spectral fluorescence of direct anterior restorative materials, Aust. Dent. J., 1977, 22, 458 CrossRef CAS PubMed.
- A. S. Hermanson, M. A. Bush, R. G. Miller and P. J. Bush, Ultraviolet illumination as an adjunctive aid in dental inspection, J. Forensic Sci., 2008, 53, 408 CrossRef PubMed.
- Z. Sun, Z. Zhu, Z. Guo, Z.-c. Wu, Z. Yang, T. Zhang and X. Zhang, Electronic structure and luminescent properties of Ce3+-doped Ba3Lu2B6O15, a high-efficient blue-emitting phosphor, Ceram. Int., 2019, 45, 7143 CrossRef CAS.
- S. W. Kim, T. Masui, H. Matsushita and N. Imanaka, Enhancement in photoluminescence of Gd2O2CO3:Tb3+ submicron particles by introducing yttrium into the oxycarbonate lattice, J. Electrochem. Soc., 2010, 157, J181 CrossRef CAS.
- K. Park, D. Hakeem, J. Pi and S. Kim, Improvement of photoluminescence properties of Ce3+-doped CaSrAl2SiO7 phosphors by charge compensation with Li+ and Na+, Ceram. Int., 2018, 44, 1929 CrossRef CAS.
- R. Yu, J. Wang, M. Zhang, J. Zhang, H. Yuan and Q. Su, A new blue-emitting phosphor of Ce3+-activated CaLaGa3S6O for white-light-emitting diodes, Chem. Phys. Lett., 2008, 453, 197 CrossRef CAS.
- S. W. Kim, K. Jyoko, T. Masui and N. Imanaka, Synthesis of green-emitting (La,Gd)OBr: Tb3+ phosphors, Materials, 2010, 3, 2506 CrossRef CAS.
- S. Zhang, Z. Mu, Y. Lv, L. Fan, Y. Li, G. Ju and Y. Hu, White-light long persistent luminescence of Tb3+-doped Y3Al2Ga3O12 phosphor, J. Alloys Compd., 2017, 729, 418 CrossRef CAS.
- Y. Li, W. Chen, L. Zhao, D. Meng, Y. Zhang and C. Wang, Ce3+ and Tb3+ activated Ba3P4O13 phosphors based on energy transfer behavior, New J. Chem., 2017, 41, 14876 RSC.
- M. Jose and A. Lakshmanan, Ce3+ to Tb3+ energy transfer in alkaline earth (Ba, Sr or Ca) sulphate phosphors, Opt. Mater., 2004, 24, 651 CrossRef CAS.
- P. Liang, Co-existence phenomenon of Ce3+/Ce4+ and Tb3+ in Ce/Tb co-doped Zn2(BO3)(OH)0.75F0.25 phosphor: luminescence and energy transfer, Adv. Powder Technol., 2019, 30, 974 CrossRef CAS.
- S. Sun, L. Wu, H. Yi, L. Wu, J. Ji, C. Zhang, Y. Zhang, Y. Kong and J. Xu, Energy transfer between Ce3+ and Tb3+ and the enhanced luminescence of a green phosphor SrB2O4:Ce3+,Tb3+, Na+, Opt. Mater. Express, 2016, 6, 1172 CrossRef CAS.
- Y. Li, X. Wei, M. Yin and Y. Tao, Energy transfer processes in Ce3+ and Tb3+ co-doped Ln2Si2O7 (Ln = Y, Gd), Opt. Mater., 2011, 33, 1239 CrossRef CAS.
- D. Jia, J. Zhu and B. Wu, Luminescence and energy transfer in CaAl4O7: Tb3+, Ce3+, J. Lumin., 2001, 93, 107 CrossRef CAS.
- S. Lee and S. Park, Preparation and luminescent properties of Tb3+ and Tb3+–Ce3+ doped Ba9Y2Si6O24 phosphors, J. Lumin., 2013, 143, 215 CrossRef CAS.
- Z. Xia and R.-S. Liu, Tunable blue-green color emission and energy transfer of Ca2Al3O6F:Ce3+,Tb3+ phosphors for near-UV white LEDs, J. Phys. Chem. C, 2012, 116, 15604 CrossRef CAS.
- F. Izumi and K. Momma, Three-Dimensional Visualization in Powder Diffraction, Solid State Phenom., 2007, 130, 15 CAS.
- K. Momma and F. Izumi, VESTA: a three-dimensional visualization system for electronic and structural analysis, J. Appl. Crystallogr., 2008, 41, 653 CrossRef CAS.
- R. D. Shannon, Revised effective ionic radii and systematic studies of interatomic distances in halides and chalcogenides, Acta Crystallogr., Sect. A: Cryst. Phys., Diffr., Theor. Gen. Crystallogr., 1976, 32, 751 CrossRef.
- M. A. Lim, J. K. Park, C. H. Kim, H. D. Park and M. W. Han, Luminescence characteristics of green light emitting Ba2SiO4:Eu2+ phosphor, J. Mater. Sci. Lett., 2003, 22, 1351 CrossRef CAS.
- D. Hakeem, J. Pi, S. Kim and K. Park, New Y2LuCaAl2SiO12:Ln (Ln= Ce3+, Eu3+, and Tb3+) phosphors for white LED applications, Inorg. Chem. Front., 2018, 5, 1336 RSC.
- D. Hakeem, J. Pi, G. Jung, S. Kim and K. Park, Structural and photoluminescence properties of La1−xNaCaGa3PZrO12 doped with Ce3+, Eu3+, and Tb3+, Dyes Pigm., 2019, 160, 234 CrossRef CAS.
- D. Hakeem, D. Kim, S. Kim and K. Park, Crystal structure and photoluminescence properties of novel garnet Y2−xLaCaGa3ZrO12:xLn3+ (Ln = Eu and Tb) phosphors, Dyes Pigm., 2019, 163, 715 CrossRef CAS.
- T. Honma, K. Toda, Z.-G. Ye and M. Sato, Concentration quenching of the Eu3+-activated luminescence in some layered perovskites with two-dimensional arrangement, J. Phys. Chem. Solids, 1998, 59, 1187 CrossRef CAS.
- J. Hölsä, M. Leskelä and L. Niinistö, Concentration quenching of Tb3+ luminescence in LaOBr and Gd2O2S phosphors, Mater. Res. Bull., 1979, 14, 1403 CrossRef.
- U. Caldiño, A. Speghini, E. Álvarez, S. Berneschi, M. Bettinelli, M. Brenci and G. C. Righini, Spectroscopic characterization and optical waveguide fabrication in Ce3+, Tb3+ and Ce3+/Tb3+ doped zinc-sodium-aluminosilicate glasses, Opt. Mater., 2012, 33, 1892 CrossRef.
- C. Zhang, T. Uchikoshi, R.-J. Xie, L. Liu, Y. Cho, Y. Sakka, N. Hirosaki and T. Sekiguchi, Reduced thermal degradation of the red-emitting Sr2Si5N8:Eu2+ phosphor via thermal treatment in nitrogen, J. Mater. Chem. C, 2015, 3, 7642 RSC.
- Y. J. Park, S. W. Kim, C. J. Kim, Y. J. Lee and J. Hwang, Development of β-SiAlON:Eu2+ phosphor in glass for high-power LED-and LD-based lighting systems using original BaO-B2O3-ZnO-SiO2 (BBZS) composition glass, J. Alloys Compd., 2019, 794, 94 CrossRef CAS.
- Y. J. Park, G. J. Jeong, J. H. Kim, Y. Lee, S. W. Kim, C. J. Kim and J. Hwang, Development of high luminous efficacy red-emitting phosphor-in-glass for high-power LED lighting systems using our original low Tg and Ts glass, Opt. Lett., 2019, 44, 6057 CrossRef CAS PubMed.
- Y. Li, L. Hu, B. Yang, M. Shi and J. Zou, Effect of sintering temperature on the photoluminescence properties of red-emitting color conversion glass, J. Mater. Sci.: Mater. Electron., 2018, 29, 2035 CrossRef CAS.
- Y. K. Lee, Y. H. Kim, J. Heo, W. B. Im and W. J. Chung, Control of chromaticity by phosphor in glasses with low temperature sintered silicate glasses for LED applications, Opt. Lett., 2014, 39, 4084 CrossRef CAS PubMed.
- W. J. Chung and Y. H. Nam, A review on phosphor in glass as a high power LED color converter, ECS J. Solid State Sci. Technol., 2019, 9, 016010 CrossRef.
- L.-Y. Chen, W.-C. Cheng, C.-C. Tsai, Y.-C. Huang, Y.-S. Lin and W.-H. Cheng, High-performance glass phosphor for white-light-emitting diodes via reduction of Si–Ce3+:YAG inter-diffusion, Opt. Mater. Express, 2014, 4, 121 CrossRef.
- S. Oshio, T. Matsuoka, S. Tanaka and H. Kobayashi, Mechanism of Luminance Decrease in BaMgAl10O17:Eu2+ Phosphor by Oxidation, J. Electrochem. Soc., 1998, 145, 3903 CrossRef CAS.
- G. Bizarri and B. Moine, On BaMgAl10O17:Eu2+ phosphor degradation mechanism: thermal treatment effects, J. Lumin., 2005, 113, 199 CrossRef CAS.
Footnote |
† Electronic supplementary information (ESI) available. See DOI: 10.1039/d1ra04384f |
|
This journal is © The Royal Society of Chemistry 2021 |