DOI:
10.1039/D1RA04321H
(Paper)
RSC Adv., 2021,
11, 36494-36501
A newly designed sticker-plastic sheet platform and smartphone-based digital imaging for protein assay in food samples with downscaling Kjeldahl digestion†
Received
4th June 2021
, Accepted 25th October 2021
First published on 11th November 2021
Abstract
A low-cost and reliable analytical method based on the combination of a newly designed sticker-plastic sheet platform, digital image-based colorimetry and down scaled Kjeldahl digestion is proposed for the determination of protein content in food samples. The yellowish-brown colloidal products, obtained from the reaction between the ammonium–nitrogen after digestion and the working Nessler's reagent on the miniaturized sticker-plastic sheet platform, were captured for imaging with a smartphone camera. The operational parameters and reaction conditions were optimized. A down scaled Kjeldahl digestion procedure was performed using the newly designed digestion block. The parameters influencing the digestion efficiency, including the mass of the sample, volume of acid, mass of the catalyst and digestion time, were evaluated. Under the selected conditions, a linear calibration in the range of 5–60 mg L−1 ammonium–nitrogen was obtained with limits of detection and quantification of 2.8 and 7.6 mg L−1, respectively. The repeatability and reproducibility were 6.7% and 8.8%, respectively. The accuracy of the proposed method was evaluated by applying the developed procedure for milk powder and feeding stuff reference materials and comparing it with the conventional Kjeldahl method. The proposed method was successfully applied for the determination of protein contents in soy foods and protein-based foods. The results agreed well with those obtained from the conventional Kjeldahl method.
1 Introduction
Proteins are major essential nutrients for the human body needed for growing, repairing cells and maintaining their proper functions.1 A deficient level of protein can lead to kwashiorkor and marasmus disease.2 Food proteins are commonly obtained either from animals (eggs, meat, fish, milk and dairy products) or plants (seeds, and cereals). Non-fermented and fermented soy foods such as soy milk, tofu, miso, and soy sauce are reported as potential functional foods for protein.3 In the last few years, an increase in protein supplement consumption could be observed; therefore, a rapid and reliable method for determination of protein content in protein-based foods is of great importance in analytical chemistry, nutritional chemistry and food research.
Several methods have been reported for protein assays in food samples,4 such as the Kjeldahl method, Dumas method, infrared spectrometry, colorimetric methods (Bradford, biuret, Lowry, and bicinchoninic acid method), and ultraviolet absorption method. Although the Kjeldahl method is a classical method, it still remains popular in today's protein analysis for food science and technology.5 It is an officially recognized standard method. It provides advantages such as high precision and good reproducibility,6 without requiring sophisticated instrumentation and infrastructure.7 In addition, it remains an accurate and reliable method for protein analysis. However, this method is a time-consuming process (digestion, distillation and titration) that requires trained technical operators. It employs hazardous chemicals at high temperature for the measurement of total nitrogen instead of protein directly.8 Although the separation of non-protein nitrogen from true protein nitrogen can be carried out by adding a protein precipitating agent or removing the non-protein nitrogen by dialysis and gel filtration,5 this additional step remains unacceptable for routine analysis. The Kjeldahl method may be considered to consist of Kjeldahl digestion as the sample treatment step and titration as the detection step. Development for downscaling analysis would be of interest as an alternative to overcome the drawbacks, by saving time and minimizing the hazardous chemicals used. Downscaled devices would allow multi-sample analysis simultaneously. A number of methods have been developed for protein analysis in various food samples. Some engaged sample treatment in converting protein to a suitable species for the detection step, such as down scaled Kjeldahl digestion,9 microwave assisted digestion,10 ultrasound assisted digestion,11 precipitation,12,13 and extraction.14,15 For the detection step, the conventional one ammonia/ammonium resulted from Kjeldahl digestion and was quantified by titration.16 Instead of conventional titration, some development involved detection steps such as flow injection with conductometric detection,9,17 spectrophotometry with various reagents10,11,13–15 and digital image-based colorimetry12,18 (Table S1†).
It would be of interest to apply colorimetric determination for ammonia/ammonium produced from Kjeldahl digestion as an alternative to the conventional titration. Various reagents for the purpose may be available including indophenol or Berthelot (alkali–phenol),19–21 Nessler (potassium mercuric iodide in alkaline solution),10,19,22,23 ninhydrin,24,25 o-phthalaldehyde,26,27 and acetyl acetone.28,29 Among those, Nessler's reagent is a commonly used reagent in environmental laboratories. Furthermore, Nessler's reagent can be commercially available as a combined ready to use reagent without tedious and/or complicated procedures; however, a drawback of this reagent is its toxicity. Downscaling the colorimetric Nessler procedure for the product obtained from the downscale Kjeldahl digestion would offer minimizing the use of the toxic chemicals.
In recent years, digital imaging using common digital image capturing devices such as a smartphone with a built-in camera, a digital camera, a scanner and a webcam camera for a colorimetric reaction has been applied for protein analysis in various types of samples such as milk,30,31 egg white,32 rice,33 serum,34,35 natural rubber latex and medical latex gloves.36 However, most of the reports were based on the biuret method or modified Lowry method development together with the conventional sample preparation.
The commercially available microwell plates have been great platforms for chemical and biochemical assays due to their easy integration with scanners, smartphones or digital cameras. Various fabrication methods for downscaling chemical analysis platforms have been reported, including microzone plates,35,37,38 spot test arrays,39,40 and microfluidic based devices,41–46 aiming to lower sample and reagent volumes, to help in minimizing wastes.
In this work, downscaling Kjeldahl digestion using a newly designed Kjeldahl digestion device and operation, coupled with a miniaturized reaction device and digital image-based method, is proposed for the determination of protein in soy foods and protein-based food samples. The ammonia–ammonium ions produced in the digestion were detected with a newly designed device consisting of a platform made from sticker-plastic sheets as a platform for the reaction with working Nessler's reagent producing yellowish-brown colloidal color, readily for photos captured with a light control box coupled with a smartphone.
2 Experimental
2.1 Chemicals and reagents
All chemicals used were of analytical reagent grade unless otherwise stated. Deionized water (Milli-Q, Millipore, USA) was used throughout the experiments. An ammonium–nitrogen standard solution (1000 mg L−1) was prepared by dissolving 0.118 g of ammonium sulphate (QRëC, New Zealand) in 25 mL water. The working Nessler's reagent was prepared by diluting the purchased reagent (catalogue no.: 109028, Merck, Germany) with 20% w/v sodium hydroxide at a ratio of 1
:
1. Sulfuric acid, 95–97% (Merck, Germany), copper(II) sulphate anhydrous and sodium sulphate (Kemaus, Australia) were used. L-Tryptophan, 98% (Sigma-Aldrich, Canada), was used for the evaluation of the recovery value. Hydrochloric acid, 37% (QRëC, New Zealand), boric acid and sodium carbonate (Kemaus, Australia) were used for the conventional Kjeldahl method.
2.2 Samples
Six samples of soy foods (soybean powder, soy milk and soy sauce) and nine samples of protein-based foods (milk powder, whey protein, whey milk, soy peptide, essence of chicken, meal replacement, cricket powder and pickled fish powder) were obtained from local markets. Solid samples were oven dried at 95–105 °C overnight or until their weights were stable. The dried samples were then individually packed in clean polyethylene bags and stored in a desiccator. Liquid samples are thoroughly homogenized before sampling for analysis. Each sample was analysed in triplicate.
Accuracy was evaluated using reference materials: (1) QCFF-MP01-1901-I-275 (milk powder; protein 17.2 ± 0.3 g/100 g) and (2) RMFF-FA01-1901-461 (feeding stuffs; protein 42.55 ± 0.85 g/100 g) obtained from the Department of Science Service, Ministry of Higher Education, Science, Research and Innovation, Thailand.
2.3 Conventional Kjeldahl method
The protocol used for the conventional Kjeldahl method followed the method outlined in the AOAC Official Method47 for protein in animal feed and pet food (984.13) and total nitrogen in milk (991.20). Briefly, a sample (0.25–1 g for a solid sample or 5 mL for a liquid sample) was accurately weighed and transferred into a Kjeldahl flask. Potassium sulphate (15 g), anhydrous copper(II) sulphate (0.04 g) and concentrated sulfuric acid (20 mL) were added into the flask. The Kjeldahl flask was then heated until dense white fumes cleared from the bulb of the flask, with continuing heating for an additional 90 min to obtain a clear blue-green solution. After cooling down, 30 mL of water was added and the digested solution was transferred to a distillation tube for distillation (Gerhardt, Germany). Boric acid solution (4% w/v, 50 mL) with methyl red and methylene blue was added to an Erlenmeyer titration flask. The flask was placed under a condenser tip. NaOH solution (50% w/w, 75 mL) was added down along the sidewall of the sample tube with no agitation. The tube was heated for 300 s. Boric acid receiving solution was titrated with standard 0.1 M HCl solution.
2.4 Down scaled Kjeldahl digestion
A sample (0.01–0.08 g for solid sample or 0.1–0.3 g for liquid sample) was accurately weighed in a glass test tube with a screw cap (15 mL capacity), and it was then mixed with a catalyst of 0.125 g of copper(II) sulphate and 1.0 g of sodium sulphate and 3 mL of concentrated sulfuric acid. The tube was placed on a homemade 37 hole digestion block (Fig. S1a†) made of iron functioning as a digestion plate (3.6 cm × 17.8 cm i.d.). The dimension of each hole was 1.8 cm diameter and 2.8 cm depth. The block was put on the top of a hotplate (Fisher Scientific, USA) to heat and digest the sample for 90 min (Fig. S1b†). During the digestion process, it was placed in a fume hood. After 90 min of digestion, the digestion tube was cooled to room temperature. The digested solution was filtered through filter paper into a 25 mL volumetric flask and the volume was adjusted to the mark with water. Blanks were also treated in the same manner but without samples.
2.5 A newly designed sticker – plastic sheet platform and light control box
The newly designed sticker-plastic sheet (Fig. 1a) as an alternative platform for the conventional transparent 96-well microplate for serving a suitable microlitre scale volume in quantifying the total Kjeldahl nitrogen by colorimetry employing Nessler's reagent with a smartphone detection, instead of titration.
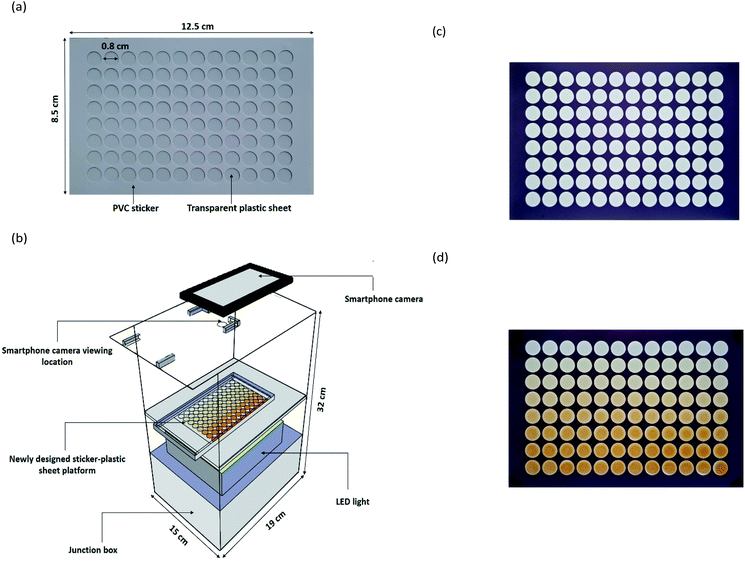 |
| Fig. 1 The newly designed sticker-plastic sheet platform: (a) photo of a ready to use platform, (b) its position in the light control box, (c) photo of the platform taken in the light control box, and (d) photo of the platform taken for the assay. | |
The sticker pattern was designed in a 96 well format using Adobe Illustrator software. Two sheets of the white PVC sticker (Kodak, 0.1 mm thickness) were stacked in 2 layers and then cut with a cutting plotter machine (Teneth, China). The obtained sticker sheet with a size of 8.5 cm × 12.5 cm × 0.2 mm with a 96 well format was stuck onto a transparent plastic sheet (PVC, 0.1 mm thickness). The dimension of each well is 0.8 cm diameter and 0.2 mm depth with a maximum capacity of 60 μL.
A laboratory-made light control box (Fig. 1b) was made from a white opaque acrylic box with an outer dimension of 19 × 32 × 15 cm. A tray was set at the middle of the box. The outside of the box was completely covered with a black PVC sticker to make sure that no light penetrated into the box. Internal illumination was provided by a LED video light with 300 high quality LED light beads of extra-large luminous chips (Yongnuo YN300 III, China). An ON/OFF switch was used to control the power supply (6.5–8.5 V, 3 A). The setup of LED light was positioned below the acrylic tray in the box used as a light diffuser and for supporting the newly designed sticker-plastic sheet platform (Fig. 1c). At the top side, a hole of a 1 cm diameter was made for photographing via a built-in camera of the smartphone (ASUS, Zenfone max plus, Taiwan).
2.6 The proposed digital image-based method for protein assay
The 96 well sticker-plastic sheet platform was placed on the acrylic tray. 30 μL of ammonium–nitrogen standard/sample solutions from down scaled Kjeldahl digestion were transferred into the well of 96 well sticker-plastic sheets with a micropipette. The working Nessler's reagent (30 μL) was then pipetted into the well using a 12 multi-channel micropipette (Nichiryo, Japan). The tray was moved into the light control box. After 3 minutes, a yellowish-brown colloidal product on each well was observed and photographed (Fig. 1d).
The captured images were analysed using ImageJ software. The values of the colour intensities in the detection zone were measured using an RGB (red, green, blue) approach (300 × 240 pixels, circle). A calibration graph plotted between the blue intensity difference (ΔI) and ammonium–nitrogen concentrations was used for examining the nitrogen content in a sample. Protein content was then evaluated by multiplying the percentage of nitrogen with a conversion factor48 and reported as % w/w protein in dry weight of sample.
3 Results and discussion
3.1 Colorimetric reaction, platform fabrication and digital image analysis
The potassium mercuric iodide in alkaline solution of this reagent can produce coloured colloidal products (λmax: 430–460 nm), yellow, orange or brown precipitates depending on the ammonium contents.7 A possible reaction for nesslerization is
With an increase of ammonia concentrations and a constant OH− concentration the composition tended towards NHHg2I2·H2O (deep brown) and finally NH2Hg2I3 (chocolate).
The use of the polymeric sticker film enables convenient fabrication of the newly designed platform as employing a cutting printer. The cut sticker sheet can be directly stuck onto the transparent plastic sheet.
This newly designed sticker-plastic sheet platform provides some advantages in terms of being simple, low-cost, re-usable, chemical resistance and an interesting alternative platform in comparison with other most popular materials. In addition, this newly designed platform is more suitable for detection of samples/products in the precipitated form. The relative standard deviation for detection of the yellowish-brown colloidal products obtained from 60 mg L−1 ammonium–nitrogen and the working Nessler's reagent using the newly-designed platform and commercial microwell plate was 5% (n = 96) and 17% (n = 96), respectively.
A series of standards and samples were treated in the wells of the newly-designed sticker-plastic sheet platform; a photo of the platform was taken and is shown in Fig. 1c. The photo was processed for imaging using ImageJ software. For quantitation purposes, the graph of RGB intensity difference (ΔI) (difference in colour intensity of the reagent blank zone and colour intensity of the standard/sample zone) was plotted against ammonium–nitrogen concentrations (Fig. S2†). It was found that the B colour intensity related to the ammonia–nitrogen concentration.
3.2 Optimization of operational parameters
3.2.1 Illumination device. To eliminate the need for a flash to capture images and to control the uniformity of light intensity during capture of images, a LED (Yongnuo YN300 III, China) was used as the illumination device. This device was set in the lower part in the light control box, below the middle of the sticker-plastic sheet tray. After that, with the captured images of the newly designed sticker-plastic sheet, the repeatability of RGB intensities was evaluated for the homogeneity of the light emitted by the LEDs. A relative standard deviation of less than 2% was obtained. This indicated good repeatability of measurements in a light control box.
3.2.2 Total volume of the reaction vessels on the newly designed sticker-plastic sheet platform. The volumes of the reaction vessels on the newly designed sticker-plastic sheet platform depend on the thickness of the sticker sheet and the diameter of the well. Due to the limitation of the cutting machine for the sticker sheet, it was found that the maximum possible thickness was 0.2 mm. Therefore, two sheets of the white PVC sticker (0.1 mm thickness) were stacked in 2 layers (as 0.2 mm thickness) and cut to a 96 well format. The well should be suitable for 60 μL operation.
3.3 Optimization of reaction conditions
In this work, the commercial ready to use Nessler's reagent was used, due to simplicity in reagent preparation. To reduce the reagent consumption, the effect of the dilution ratio of Nessler's reagent was studied: 1
:
0, 1
:
0.25, 1
:
0.5, 1
:
1, 1
:
2, and 1
:
3 (the purchased Nessler's reagent
:
water).
It was found that the dilution up to the ratio of 1
:
1 resulted in practically the same calibration graph but the higher dilution resulted in lower sensitivity. This could be due to the amount of the reagent as well as lowering the alkalinity. In further experiments, 20% w/v NaOH solution was used instead of water for the dilution to maintain the alkalinity of the reaction.49
The effect of reaction time on the colour development was investigated within the range 1–10 min. No significant difference in slopes of calibration graphs was observed for any reaction time in the study range. 3 min was chosen for further experiments.
It should be noted that the volume per test of 15 μL of the purchased commercially available Nessler's reagent was consumed. This significantly minimized hazardous waste.
3.4 Optimization for down scaled Kjeldahl digestion
In order to reduce the consumption of chemicals/reagents in the conventional Kjeldahl digestion,47 the down scaled Kjeldahl digestion procedure was developed using a homemade iron digestion plate as a block digester. Soybean powder and soy sauce were used as models for solid and liquid samples, respectively, in this study. L-Tryptophan standard was used for recovery study by spiking the standard into the studied models.
3.4.1 Mass of the solid sample. The mass of the sample (soybean powder model) was evaluated in a univariate mode by fixing the other variables at concentrated sulfuric acid 3 mL, copper(II) sulphate 0.125 g, sodium sulphate 1.0 g and digestion time 90 min. The mass of the sample utilized in digestion was varied from 0.013, 0.025, 0.050, 0.075 and 0.100 g. High percentage recoveries (>90%) as presented in Fig. S3a† were obtained for all sample masses studied, except for 0.100 g, for which incomplete digestion sample (from visual observation) was observed. Therefore, a sample mass in a range of 0.013–0.075 g was suitable for digestion under these conditions. The mass of the sample employed for protein analysis can be chosen according to their amount in the sample. A solid sample of 0.025 g was taken for down scaled Kjeldahl digestion.
3.4.2 Volume of sulfuric acid. Concentrated sulfuric acid has been used for matrix dissolution/mineralization of organic matters in protein-based samples without oxidizing the liberated ammonia to gaseous nitrogen.5 The amount of acid required for the decomposition of the sample will vary depending upon the size of the sample and relative amount of organic matters in the sample. The volumes of concentrated sulfuric acid were varied between 1 and 5 mL. High percentage recoveries were obtained from a volume in a range of 2–3 mL as shown in Fig. S3b.† However, an incomplete digestion sample (from visual observation) was obtained when less than 2 mL of concentrated sulfuric acid was used. The volume higher than 3 mL gave lower recoveries due to overflow of the digested solution from the digestion vessels during digestion. Thus, 3 mL of concentrated sulfuric acid was selected for further study.
3.4.3 Mass of copper(II) sulphate and sodium sulphate. Copper(II) sulphate was used as a catalyst to reduce time and increase the digestion efficiency of the Kjeldahl digestion procedure.5 In addition, sodium sulphate or potassium sulphate has been used to increase the boiling point of sulfuric acid and the oxidizing power for digestion.50 Copper(II) sulphate was studied in the range of 0.03–0.50 g. As shown in Fig. S3c,† it was found that quantitative recoveries were obtained when using copper(II) sulphate more than 0.05 g. For further work, 0.125 g of copper(II) sulphate was chosen.The amount of sodium sulphate was also investigated by varying it from 0.10 to 1.5 g as presented in Fig. S3d.† It was found that 1.0 g of sodium sulphate resulted in high percentage recovery and was selected for further experiments.
3.4.4 Digestion time. The influence of digestion time on the digestion efficiency was investigated by studying the range of 30 to 120 min. Practically quantitative recoveries were observed when using 90 min or above (Fig S3e†).
3.4.5 Mass of the liquid sample. The mass of soy sauce as a model for liquid sample was evaluated by varying 0.05, 0.1, 0.2, 0.3 and 0.5 g sample with employing the above optimized parameters of concentrated sulfuric acid 3.0 mL, copper(II) sulphate 0.125 g, sodium sulphate 1.0 g and digestion time 90 min. Practically quantitative recoveries were obtained (Fig. S3f†) for a sample mass in the range of 0.10–0.30 g. Therefore, a liquid sample mass in the range of 0.10–0.30 g was suitable for digestion under the conditions.
3.5 Analytical characteristics of the proposed method
The analytical characteristics of the proposed method were investigated. The matrix-matched calibration was employed. To eliminate the color matrix effects from the copper catalyst, when analyzed in the digested sample solutions, the aliquot of digestion blank was added to all concentrations of ammonium–nitrogen standard solutions.
Under the optimum conditions for digestion and determination of total Kjeldahl nitrogen as described above, a standard calibration curve of ammonium–nitrogen in the range of 5–60 mg L−1 was constructed by plotting the B intensity difference (ΔI) against concentrations. A linear calibration graph was obtained with the calibration equation y = 2.302X − 1.609, R2 = 0.994 as presented in Fig. 2. The limits of detection (LOD) (3σ/s) and quantification (LOQ) (10σ/s) (where σ is the standard deviation of the digestion blank (n = 9) and s is the slope of the calibration curve) were obtained to be LOD 2.8 mg L−1 and LOQ 7.6 mg L−1. The relative standard deviations for determinations of 20 mg L−1 ammonium–nitrogen were 6.7% for repeatability (n = 8) and 8.8% for reproducibility (n = 5).
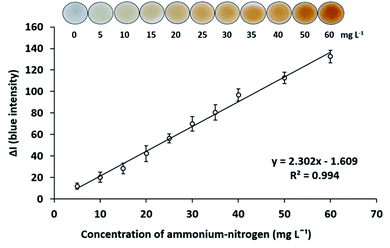 |
| Fig. 2 Calibration graph of ammonium–nitrogen with working Nessler's reagent in the concentration range of 5–60 mg L−1. | |
Accuracy of the proposed method was evaluated by employing the method for reference materials of milk powder and feeding stuffs. As presented in the results in Table 1, there is no evidence of significant difference between the protein contents obtained by the proposed method and the reference values at 95% confidence level (tcritical = 4.303, tcalculate = 1.407 and 0.866 for milk powder and feeding stuffs, respectively).
Table 1 Assays of reference materials and samples
Sample |
Proteina (g/100 g) |
Reference values (g/100 g) |
% Recoveryb |
Proposed method |
Conventional Kjeldahl method |
Protein = total nitrogen × nitrogen-to-protein conversion factor (6.25 for normal food; 6.38 for dairy products; 5.71 for soybean). Recovery (%) = (protein found with the proposed method/protein found with the conventional Kjeldahl method as the standard method) × 100. |
Milk powder |
18.5 ± 1.6 |
— |
17.2 ± 0.3 |
— |
Feeding stuffs |
43.1 ± 1.1 |
— |
42.55 ± 0.85 |
— |
Soybean powder |
40.0 ± 1.8 |
39.58 ± 0.56 |
— |
101 |
Soy sauce |
13.5 ± 1.1 |
12.63 ± 0.58 |
— |
107 |
Performance of the proposed method was tested for digestion and determination of protein contents in soybean powder (n = 10) and soy sauce samples (n = 10). The conventional Kjeldahl method47 was used as the reference method for comparison. According to t-test at 98% confidence limit, the results obtained from both methods were in good agreement (tcritical = 2.552, tcalculate = 0.705 and 2.212 for soybean powder and soy sauce, respectively) as summarized in Table 1.
The proposed method with the down scaled Kjeldahl digestion procedure and the newly designed sticker-plastic sheet platform and smartphone-based digital imaging could provide the analysis results for protein contents the same as the conventional Kjeldahl method (digestion, distillation and titration). The proposed method offers some advantages over the conventional Kjeldahl method, such as low costs, ease of operation, low chemical/reagent consumption, high sample throughput of more than a dozen samples that can be treated simultaneously and high sample measurement frequency (16 samples per 3 min or 320 samples per hour).
3.6 Application to real samples
The proposed down scaled Kjeldahl digestion prior to the smartphone-based digital image method using the newly designed sticker-plastic sheet platform was employed for determination of protein content in soy foods and protein-based food samples. The conventional Kjeldahl method was used as a reference method for comparison. The results from both methods presented in Table 2 were in good agreement at 95% confidence level (tcritical = 2.145, tcalculate = 0.084). To perform the recovery study, all samples were spiked with L-tryptophan standard. Satisfactory results were obtained with percentage recoveries of 92–108% and 91–107% for the proposed method and the conventional Kjeldahl method, respectively. The relative standard deviations (RSDs) of the recovery studies for the proposed method and for the conventional Kjeldahl method were less than 10% and less than 23%, respectively. The proposed method was demonstrated to have a good recovery and its effectiveness was confirmed in the quantification of protein content in commercial protein-based foods. The highest amount of protein content was obtained in whey protein; however, the cricket powder is one of the most high protein animal-based foods.
Table 2 Protein contents in some food samples
Sample |
Label protein (g/100 g) |
Proposed method |
Conventional Kjeldahl method |
% Labelc |
Proteina (g/100 g) |
% Recoveryb |
Proteina (g/100 g) |
% Recoveryb |
Protein = total nitrogen × nitrogen-to-protein conversion factor (6.25 for normal food; 6.38 for dairy products; 5.71 for soybean). Recovery (%) = [(Cspliked − Cunspilked)/Cadded] × 100. Label (%) = [Cproposed/Clabel] × 100. |
Soy milk |
— |
1.5 ± 0.3 |
107 |
1.55 ± 0.16 |
94 |
— |
Soy milk |
— |
2.6 ± 0.6 |
108 |
3.07 ± 0.12 |
99 |
— |
Soybean powder |
40 |
40.0 ± 1.8 |
95 |
39.58 ± 0.56 |
101 |
100 |
Soybean powder |
18 |
17.5 ± 1.3 |
99 |
18.47 ± 0.61 |
95 |
97 |
Seasoning soy sauce |
13.3 |
13.5 ± 1.1 |
97 |
12.63 ± 0.58 |
97 |
102 |
Thin soy sauce |
6.6 |
5.1 ± 0.6 |
99 |
6.08 ± 0.22 |
101 |
77 |
Soy peptide |
4 |
3.7 ± 0.5 |
106 |
3.83 ± 0.16 |
95 |
93 |
Essence of chicken |
— |
6.4 ± 0.5 |
106 |
6.13 ± 0.26 |
91 |
— |
Meal replacement |
15.9 |
17.0 ± 0.4 |
99 |
16.67 ± 0.52 |
107 |
107 |
Milk powder |
20 |
21.7 ± 0.7 |
99 |
20.75 ± 0.25 |
105 |
109 |
Whey milk |
8 |
7.7 ± 0.3 |
103 |
8.44 ± 0.24 |
91 |
96 |
Whey protein |
56 |
57.1 ± 1.2 |
108 |
56.04 ± 0.82 |
102 |
102 |
Whey protein |
88.6 |
74.9 ± 2.8 |
92 |
71.54 ± 0.71 |
91 |
85 |
Cricket powder |
67.8 |
62.5 ± 2.4 |
96 |
66.10 ± 1.30 |
93 |
92 |
Pickled fish powder |
— |
12.6 ± 0.8 |
103 |
13.40 ± 0.42 |
103 |
— |
It should be noted that the Kjeldahl method indirectly quantified the total protein content of foods by direct nitrogen measurement multiplication by a conversion factor. The presence of non-protein nitrogen compounds in foods (amino acids, ammonia, urea, and trimethylamine oxide) overestimates their true protein content.5 Therefore, the adulteration of some organic nitrogen compounds in food such as melamine in milk could not be analyzed by this method. Except, the separation of non-protein nitrogen from true protein nitrogen might be carried out before analysis. Although the non-protein nitrogen was not separated during analysis in this work, the analysis of protein contents in samples by the proposed method supports the label values (Table 2). These results indicated that the proposed method can be used as an alternative method for fast total protein analysis in food samples, although it cannot provide information regarding adulteration.
4 Conclusions
The proposed method based on a newly designed sticker-plastic sheet platform and smartphone-based digital imaging after down scaled Kjeldahl digestion was able to quantify protein contents in food samples with good accuracy and precision, which was in good agreement with the results from the conventional Kjeldahl method (digestion, distillation and titration). The proposed method significantly increases the performance of the protein analysis method in terms of speed, simplicity, low-cost, portability, low chemical consumption and low waste production (3 mL for digestion and 60 μL for detection) which means that it is also environmentally friendly. This method has been successfully applied for the determination of protein contents in soy foods and protein-based foods. The proposed method offers advantages over previous methods through reduced chemical/reagent consumption and use of inexpensive devices that are easy to implement in low resource areas and especially where budget is limited. In addition, this method has high potential as a good alternative method for quality assurance of protein contents in raw materials and agricultural products.
Author contributions
P. Didpinrum: investigation, methodology, validation, data curation, formal analysis, visualization, and writing – original draft. W. Siriangkhawut: conceptualization, resources, investigation, methodology, validation, data curation, formal analysis, writing – original draft, and writing – review & editing. K. Ponhong: conceptualization, resources, investigation, methodology, validation, data curation, and writing – review & editing. P. Chantiratikul: resources and methodology. K. Grudpan: conceptualization, resources, writing – review & editing, supervision, and funding acquisition. All authors have read and agreed to the published version of the manuscript.
Conflicts of interest
There are no conflicts to declare.
Acknowledgements
The authors gratefully acknowledge the Thailand Research Fund (TRF) through the Research and Researchers for Industries (RRi) (Grant No. PHD59I0057) for a scholarship (P. Didpinrum) and TRF Distinguished Research Professor Award Grant and Research Grant (DPG608002) (K. Grudpan) for financial support. Additional support was received from Faculty of Science, Mahasarakham University; the Center of Excellence for Innovation in Chemistry (PERCH-CIC), Ministry of Higher Education, Science, Research and Innovation; the Center of Excellence for Innovation in Analytical Science and Technology (I-ANALY-ST), Chiang Mai University; and Metrohm Siam Co., Ltd.
References
- V. Vaclavik and E. W. Christian, Essentials of Food Science, New York, USA, 4th edn, 2014 Search PubMed.
- G. Wu, Food Funct., 2016, 7, 1251–1265 RSC.
- K. I. Chen, M. H. Erh, N. W. Su, W. H. Liu, C. C. Chou and K. C. Cheng, Appl. Microbiol. Biotechnol., 2012, 96, 9–22 CrossRef CAS PubMed.
- S. K. Chang and Y. Zhang, Food Analysis, Springer, Cham, Switzerland, 2017, pp. 315–331 Search PubMed.
- P. Sáez-Plaza, T. Michałowski, M. J. Navas, A. G. Asuero and S. Wybraniec, Crit. Rev. Anal. Chem., 2013, 43, 178–223 CrossRef.
- Analysis of Proteins Online, https://people.%20umass.edu%20/%7Emcclemen/581Proteins.html, accessed September 2021 Search PubMed.
- P. Sáez-Plaza, M. J. Navas, S. Wybraniec, T. Michałowski and A. G. Asuero, Crit. Rev. Anal. Chem., 2013, 43, 224–272 CrossRef.
- T. Whitesell, J. L. Aalhus, I. L. Larsenand and M. Juarez, J. Food Compos. Anal., 2014, 33, 67–70 CrossRef CAS.
- P. Yanu and J. Jakmunee, Food Chem., 2017, 230, 572–577 CrossRef CAS PubMed.
- C. Domini, L. Vidal, G. Cravotto and A. Canals, Ultrason. Sonochem., 2009, 16, 564–569 CrossRef CAS PubMed.
- G. Drochioiu, C. I. Ciobanu, S. Bancila, L. Ion, B. A. Petre, C. Andries, R. V. Gradinaru and M. Murariu, Ultrason. Sonochem., 2016, 29, 93–103 CrossRef CAS PubMed.
- A. F. S. Silva and F. R. P. Rocha, Food Control, 2020, 115, 107299 CrossRef CAS.
- V. de L. M. Finete, M. M. Gouvêa, F. F. de C. Marques and A. D. P. Netto, Food Chem., 2013, 141, 3649–3655 CrossRef CAS PubMed.
- N. K. K. Kamizake, M. M. Gonçalves, C. T. B. V. Zaia and D. A. M. Zaia, J. Food Compos. Anal., 2003, 16, 507–516 CrossRef CAS.
- J. E. Jablonski, T. Fu and L. S. Jackson, J. AOAC Int., 2010, 93, 213–220 CAS.
- S. Nozawa, A. Hakoda, K. Sakaida, T. Suzuki and A. Yasui, Anal. Sci., 2005, 21, 1129–1132 CrossRef CAS PubMed.
- J. Junsomboon and J. Jakmunee, Anal. Chim. Acta, 2008, 627, 232–238 CrossRef CAS PubMed.
- J. Sun, C. Hou, J. Feng and X. Wang, J. Food Qual., 2008, 31, 250–263 CrossRef CAS.
- A. Sasongko, R. W. Nugroho and D. Mulyani, J. Sains Teknol., 2018, 7, 126–134 CrossRef.
- M. D. Krom, Analyst, 1980, 105, 305–316 RSC.
- T. Thongkam, R. Rungsirisakun and K. Hemavibool, Anal. Methods, 2020, 12, 4649–4656 RSC.
- L. N. Demutskaya and I. E. Kalinichenko, J. Water Chem. Technol., 2010, 32, 90–94 CrossRef.
- K. Kolacinska and R. Koncki, Anal. Sci., 2014, 30, 1019–1022 CrossRef CAS PubMed.
- D. G. Abernathy, G. Spedding and B. Starcher, J. Inst. Brew., 2009, 115, 121–127 CrossRef.
- H. M. Baker and K. F. Alzboon, Eur. J. Chem., 2015, 6, 135–140 CrossRef CAS.
- Y. Liang, C. Yan, Q. Guo, J. Xu and H. Hu, Anal.
Chem. Res., 2016, 10, 1–8 CrossRef CAS.
- Y. Zhu, D. X. Yuan, Y. M. Huang, J. Ma and S. C. Feng, Anal. Chim. Acta, 2013, 794, 47–54 CrossRef CAS PubMed.
- A. S. Amin and M. M. Zareh, Microchim. Acta, 1996, 124, 227–233 CrossRef CAS.
- M. B. Devani, C. J. Shishoo, S. A. Shah and B. N. Suhagia, J. - Assoc. Off. Anal. Chem., 1989, 72, 953–956 CrossRef CAS.
- A. F. S. Silva and F. R. P. Rocha, Food Control, 2020, 115, 107299 CrossRef CAS.
- S. Kucheryavskiy, A. Melenteva and A. Bogomolov, Talanta, 2014, 121, 144–152 CrossRef CAS PubMed.
- T. A. Lerma, J. M. Martinez and E. M. Combat, J. Sci. Technol. Appl., 2019, 6, 53–64 CrossRef.
- J. P. Sun, C. Y. Hou, J. Feng and X. Wang, J. Food Qual., 2008, 31, 250–263 CrossRef CAS.
- C. L. M. Morais, A. C. O. Neves, F. G. Menezes and K. M. G. Lima, Anal. Methods, 2016, 8, 6458–6462 RSC.
- K. A. Oliveira, T. M. G. Cardosa, H. F. Oliveira, M. C. S. Fioravanti and W. K. T. Coltro, J. Braz. Chem. Soc., 2021, 32, 311–319 CAS.
- N. Bang-iam, Y. Udnan and P. Masawat, Microchem. J., 2013, 106, 270–275 CrossRef CAS.
- N. S. Moreira, C. L. S. Chagas, K. A. Oliveira, G. F. Duarte-junior, F. R. De Souza, M. Santhiago, C. D. Garcia, L. T. Kubota and W. K. T. Coltro, Anal. Chim. Acta, 2020, 1119, 1–10 CrossRef CAS PubMed.
- P. B. M. Silva, K. A. Oliveira and W. K. T. Coltro, J. Braz. Chem. Soc., 2017, 28, 197–201 CAS.
- L. P. S. Benedetti, V. B. dos Santos, T. A. Silva, E. B. Filho, V. L. Martins and O. Fatibello-Filho, Anal. Methods, 2015, 7, 4138–4144 RSC.
- S. Soares, M. J. A. Lima and F. R. P. Rocha, Microchem. J., 2017, 133, 195–199 CrossRef CAS.
- B. M. Jayawardane, I. D. McKelvie and S. D. Kolev, Anal. Chem., 2015, 87, 4621–4626 CrossRef CAS PubMed.
- P. Phansi, S. Sumantakul, T. Wongpakdee, N. Fukana, N. Ratanawimarnwong, J. Sitanurak and D. Nacapricha, Anal. Chem., 2016, 88, 8749–8756 CrossRef CAS PubMed.
- T. Thongkam, R. Rungsirisakul and K. Hemaviboon, Anal. Methods, 2020, 12, 4649–4656 RSC.
- L. Bueno, G. N. Meloni, S. M. Reddy and T. R. L. C. Paixao, RSC Adv., 2015, 5, 20148–20154 RSC.
- J. S. Ng and M. Hashimoto, RSC Adv., 2020, 10, 29797–29807 RSC.
- X. Tong, L. Ga, R. Zhao and J. Ai, RSC Adv., 2021, 11, 8793–8820 RSC.
- Official Methods of Analysis of AOAC International, Official Method 984.13 and 991.20, AOAC International, Gaithersburg, Maryland, USA, 18 th edn, 2005 Search PubMed.
- D. B. Jones, Br. Food J., 1932, 34, 1–10 CrossRef.
- O. Folin and W. Denis, J. Biol. Chem., 1916, 26, 473–489 CrossRef CAS.
- N. Yoshikuni, Talanta, 1994, 41, 89–92 CrossRef CAS PubMed.
Footnote |
† Electronic supplementary information (ESI) available. See DOI: 10.1039/d1ra04321h |
|
This journal is © The Royal Society of Chemistry 2021 |