DOI:
10.1039/D1RA03677G
(Paper)
RSC Adv., 2021,
11, 22835-22841
A dual-mode visual detector for toxic hydrazine†
Received
11th May 2021
, Accepted 17th June 2021
First published on 28th June 2021
Abstract
Hydrazine (N2H4) is one of the commonly used chemical reagents in numerous industries and applications but its toxicity to humans poses a need to develop simple visual detection methods. Herein, we demonstrate a novel dual-mode system to detect and simultaneously consume hydrazine in vapour and solution by using a small photoresponsive molecule that has altered optical response (both colourimetric and fluorescent) after reacting with hydrazine.
1. Introduction
Hydrazine (N2H4) is classified as a frequently used industrial substrate with applications in sectors including the aerospace industry as rocket fuel propellants,1,2 the pharmaceutical industry,3 and chemical industries that produce textile dyes and pesticides.4,5 Despite hydrazine's growing demand for these and other industries, it is categorized as a likely carcinogen and is toxic for the liver, kidneys and the central nervous system of humans.5–7 Humans can be exposed to hydrazine by inhaling its vapours or ingesting water contaminated with it, which can lead to symptoms including irritation of eyes, nose, and throat, dizziness, and nausea.5 Hydrazine and its derivatives are also considered as genotoxic impurities that can form methyl adducts with nucleotide bases leading to DNA damage and gene mutations.8,9 All of these severe effects have resulted in the US Environmental Protection Agency's (EPA) suggesting a low threshold limit value (∼10 ppb).7,8 Even the residual fuel-based debris falling into the oceans after satellite launches has led to severe environmental concerns globally because of hydrazine.10 This fact and the steady increase in supply and demand of hydrazine makes it essential to develop simple, user-friendly, and cost-effective methods for detecting this toxic substance.11,12
Existing detection methods that employ potentiometry,13 ion-selective electrodes,14 capillary electrophoresis15 metal and metal oxide-based nanostructures16–18 rely on the fabrication of tools, technical processes and time-consuming detection. Chromatography-based techniques4,19–21 rely on the low volatility and highly polar nature of hydrazine.4 These methods also suffer from fundamental problems associated with sophisticated equipment and experiment design, long processing times and greater equipment costs, which limit their use in public places.
Small molecule-based probes can detect hydrazine due to the toxin's nucleophilic behavior by reacting with it to produce a visual change in colour making them potentially more cost-effective and user-friendly.6,22–36 While fluorescence-based methods have the advantage of being highly sensitive, an optical response based on a simple change in the colour of a material can be more suitable for ‘naked-eye’ detection. A method that combines both optical techniques would provide a heightened level of reliability.3,37,38
Photochromic molecular systems reversibly transform into two or more easily recognizable, differently coloured forms when exposed to different colours of light and have been successfully used as detectors for small analytes.39 Those based on the dithienylethene backbone are of specific interest due to their noteworthy optical and thermal properties, and fast response times.40–44 These small organic molecules can easily be integrated in bulk materials to act as surface coatings on construction materials and textiles, and would not require any additional electronic controls when making the user aware of the presence of target analytes.
The reported examples of dithienylethene-based photochromic detectors have targeted metal ions,45–47 anions,48,49 biomolecules50 and toxic gases.51–53 The example we describe in this report detects hydrazine. Our photoresponsive compound offers a straightforward, easy-to-read visual colour change when exposed to hydrazine. A ‘turn-on’ emission output signal complements the change in colour and provides an additional way to detect this toxic analyte.
Our molecular design was inspired by the reaction of molecular backbones containing nitrile functional groups with hydrazine and the photoresponsive behaviour of the dithienylethene architecture as shown in Scheme 1. Compound 1o contains a photoreactive hexatriene common to all dithienylethenes and undergoes a ring-closing reaction when exposed to UV light to produce isomer 1c. While the ring-open isomer (1o) is colourless, its ring-closed counterpart is coloured due to the extended π-conjugated system running along the backbone of the molecule. Visible light is absorbed by 1c and drives the equilibrium back to 1o resetting the system.
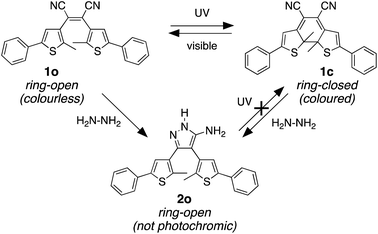 |
| Scheme 1 The reversible photoreactions of isomers 1o and 1c. The ring-open isomer (1o) is colourless due to the lack of electronic conjugation between the two heterocycles. The ring-closed counterpart (1c) is coloured because of the extended conjugated π-system running along the backbone. The reaction of either isomer with hydrazine produces 2o, which is not photoresponsive. | |
It is well-known that hydrazine readily reacts with substituted cyanoethylenes to produce aminopyrazoles.54–56 In our case, both isomers (1o and 1c) react with hydrazine to produce the same aminopyrazole (2o), which is predicted to be non-photoresponsive as already demonstrated for similar pyrazole containing dithienylethenes57 and, therefore, is colourless. While the ring-open isomer (1o) is also colourless, it has limited appeal as a visual detector of hydrazine, although as will be shown, it does provide a means of detection using changes in ‘turn-on’ emission. The ring-closed isomer offers a convenient way to detect hydrazine because the blue colour of 1c should disappear when it is converted to 2o. In this manuscript, we describe how both visual changes in colour and emission offer facile methods to detect an important toxin. An additional appeal is that our detector traps hydrazine and removes it from the environment.
2. Results and discussion
2.1 Synthesis of compounds 1o and 1c
2.1.1 Synthesis of ring-open isomer 1o. The photoresponsive dithienylethene (1o) can be synthesized in seven steps from commercially available 2-methylthiophene as described in the literature and as shown in Scheme 2.58,59
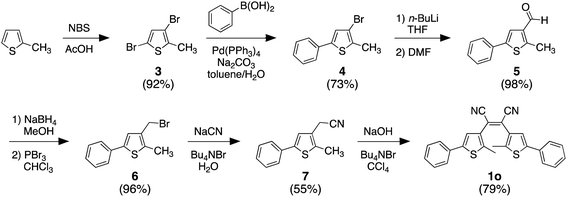 |
| Scheme 2 Synthesis of photoresponsive compound 1o. | |
2.1.2 Photochemistry of ring-open isomer 1o to prepare ring-closed isomer 1c. The photochromism of compound 1o can be visualized by exposing a CHCl3 solution of it (1.0 × 10−5 M) to a light source centred at 312 nm and measuring the UV-vis absorption spectra during the irradiation. As illustrated in Fig. 1, there is a decrease in the high-energy absorption bands (∼290 nm) and an appearance of a band centred at 580 nm, which accounts for the colour change from pale yellow to deep blue (Fig. 1 inset). At this concentration the system reaches an equilibrium after 2 minutes, at which point the changes in colour stop and the photostationary state is reached. The same photoreaction can also be monitored by 1H NMR spectroscopy using CDCl3. Naturally, the reaction is significantly slower at the higher concentrations needed for NMR spectroscopy (5 × 10−3 M) and is complete after 2 hours. The photostationary state contains 51% of the ring-closed isomer 1c as measured by comparing relative integrals for chemical shifts for each isomer (ESI†). The remaining 49% corresponds to the ring-open isomer 1o. The ring-closed isomer can be isolated by column chromatography using silica gel and 2
:
1 CH2Cl2/hexanes as the eluant and is stable as long as it is kept away from light.
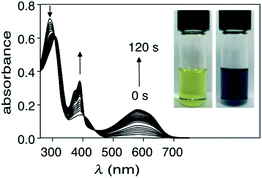 |
| Fig. 1 Changes in the UV-vis absorption spectra when a CHCl3 solution (1.0 × 10−5 M) of ring-open isomer 1o is exposed to 312 nm light for 2 minutes. The inset shows the colour change as a result of the photocyclization. | |
2.2 Treatment of 1c with hydrazine to produce 2o
When a solution of ring-closed isomer 1c in DMSO is treated with hydrazine monohydrate, there is a rapid change in the absorption spectrum and an obvious change in colour of the solution (Fig. 2). In the spectrum, the broad band centred at 580 nm characteristic for the ring-closed isomer disappears and the band at 290 nm increases. From the pseudo-first order plot of these changes, a rate constant of 5.5 × 10−3 s−1 for the disappearance of 1c can be estimated (see ESI† for details).
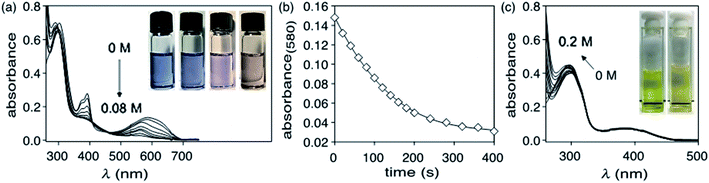 |
| Fig. 2 (a) Changes in the UV-vis absorption spectra when a DMSO solution of the isolated ring-closed isomer 1c (4.8 × 10−5 M) is treated with increments of 0.5 μL hydrazine monohydrate for a total added volume of 4 μL (equating to a total of 0.08 M N2H4), (b) the change in intensity of the absorption band at 580 nm when a DMSO solution (4.8 × 10−5 M) of the isolated ring-closed isomer 1c is treated with hydrazine monohydrate (0.08 M N2H4). (c) Changes in the UV-vis absorption spectra when a DMSO solution of the ring-open isomer 1o (9.6 × 10−6 M) is treated with increments of 0.5 μL hydrazine monohydrate for a total added volume of 10 μL (equating to a total of 0.20 M N2H4). The insets in ‘a’ and ‘c’ show the colour change for each experiment. | |
The product of the reaction of 1c with hydrazine can be isolated by column chromatography using silica gel and 15% EtOAc in hexanes as the eluant and confirmed as 2o using 1H and 13C NMR spectroscopy, and FT-IR spectroscopy (ESI†). The most characteristic change when 1c reacts with hydrazine is the disappearance of the band corresponding to C
N stretching at
= 2210 cm−1 in the FT-IR spectrum with the simultaneous appearance of a new set of bands corresponding to the amines (N–H stretching at
= 3319–3076 cm−1, N–H bending at
= 1625 cm−1, and C–N stretching at
= 1160 cm−1). In case of 1H NMR spectroscopy, the peak corresponding to the thiophene protons of 1c shift downfield from δ = 6.72 ppm to δ = 7.10 ppm supporting the restoration of aromaticity of the thiophene π-system as a result of ring-opening (for comparison, the thiophene proton in 1o appears at 6.91 ppm in the 1H NMR spectrum). The 13C NMR results show the disappearance of the peak at δ = 115.62 ppm corresponding to the carbon atoms of the nitrile groups and appearance of peaks at δ = 117.81 ppm for the bridging carbons of central pyrazole ring and δ = 153.24 ppm for carbon atom connected to the NH2 group. Tautomer 3o can be ruled out as the product based on density functional theory (DFT) computational methods based, which estimates that it is less stable than 2o (ESI†).
We propose the mechanism shown in Scheme 3 to explain the conversion of the ring-closed isomer (1c) to pyrazole 2o. Initially hydrazine acts as a nucleophile in an addition reaction opening the cyclohexadiene ring system. Elimination followed by cyclization produces the aminopyrazole as described in the literature. The conversion of ring-open 1o to pyrazole 2o follows what has already been reported for cyanoethene derivatives.54–56
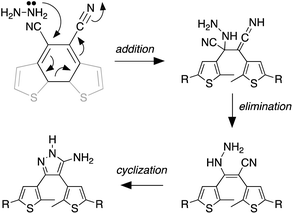 |
| Scheme 3 The mechanism that accounts for the ring-opening reaction in the presence of hydrazine. For the full mechanism, see ref. 54–56. | |
2.3 Limit of detection (LoD) of hydrazine in solution
The limit of detection (the lowest concentration of hydrazine that can be measured with reasonable certainty)60 using the ring-closed isomer (1c) can be estimated by treating a solution of 1c (4.8 × 10−5 M) in DMSO with a stock solution of hydrazine monohydrate (1.7 × 10−4 M) in DMSO in incremental amounts (5 μL) for a total of 110 μL (corresponding to a total concentration of 1.7 × 10−5 M N2H4), and monitoring the decrease in the absorbance at 580 nm (ESI†). The LoD of hydrazine obtained from linear regression is estimated to be 9.0 × 10−7 M (29 ppb) (ESI†). The value of limit of detection obtained for probe 1c is much lower than the permissible exposure limit (PEL) for hydrazine determined by the federal Occupational Safety and Health Administration (OSHA) (1 ppm, 8 h time-weighted average),61 and the non-disabling and transient acute exposure guideline level (AEGL-1) established by US EPA (0.1 ppm, 8 h time-weighted average).62 However, the value obtained remains higher than the TLV recommended by American Conference of Governmental Industrial Hygienists (ACGIH) (0.01 ppm, 8 h time-weighted average).62
2.4 Changes in emission
In addition to the conveniently observed change in colour, the photoisomerization process also causes changes in the electronic structure of the molecule that can, in turn, influence the luminescence of 1o and 1c.63 Unlike the majority of dithienylethenes, which are weakly emissive in both their ring-open and ring-closed forms, compound 1o is observably emissive due to a localized donor–acceptor intramolecular charge transfer from electron-rich thiophenes to strongly electron-poor nitrile groups (Fig. 3).64,65 On the other hand, ring-closed isomer 1c has π-electrons delocalized over the entire molecular backbone and is only weakly emissive.66,67 Treating DMSO solutions of either isomer with hydrazine monohydrate results in a substantial increase in emission (Fig. 3), which can also be used as an output signal for the detection.
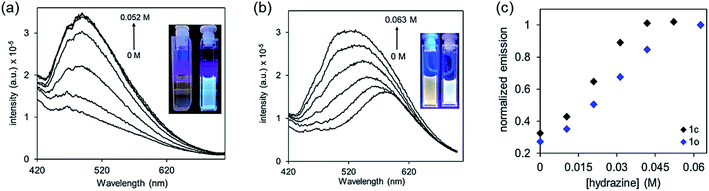 |
| Fig. 3 (a) Changes in the emission spectrum when a DMSO solution of the isolated ring-closed isomer 1c (4.8 × 10−5 M) is treated with increments of 1.0 μL hydrazine monohydrate for a total added volume of 4 μL (0.052 M N2H4), (b) a DMSO solution of the ring-open isomer 1o (9.6 × 10−6 M) is treated with increments of 1 μL hydrazine monohydrate for a total added volume of 6 μL (0.063 M N2H4), and (c) the normalized emission for each experiment. The insets show the change in emission for each experiment. | |
2.5 Detection of hydrazine vapours
Visual detection of hydrazine vapour can be readily demonstrated by exposing a piece of filter paper treated with a few drops of a solution of 1c (1 mg) dissolved in 0.1 mL of DMSO, drying the paper in a vacuum oven, placing it elevated in a vial containing 20 drops of hydrazine monohydrate and gently heating to generate hydrazine vapour. The originally blue and non-emissive paper loses its blue colour after a few minutes of exposure and becomes highly emissive under 365 nm light (Fig. 4). When exposed to light of wavelength 365 nm the filter paper remains pale yellow and emissive demonstrating that the non-photoresponsive 2o was generated.
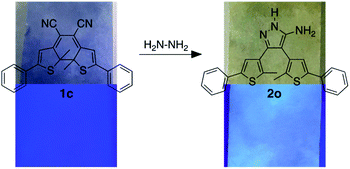 |
| Fig. 4 Changes in colour (top) and emission (bottom, λex = 365 nm) of a piece of filter paper soaked in compound 1c and exposed to vapours of hydrazine. | |
2.6 Competitive analytes
The selectivity of compound 1c to hydrazine over other possible interfering analytes such as cations (K+, Ca2+, Zn2+, Na+, Mg2+, NH4+), anions (I−, Br−, CO32−, OAc−, Cl−, SO42−), triethylamine (NEt3) and phenylhydrazine can be established by carrying out parallel UV-vis absorption experiments. When DMSO solutions of ring-closed isomer 1c (4.8 × 10−5 M) are treated with 20 molar equivalents of various analytes in ultrapure water (1.0 × 10−3 M) and incubated in dark at 24 °C for 30 min to ensure complete reaction, only the solution treated with hydrazine loses its deep blue colour and has a significant decrease in the intensity of the absorption band centred at 585 nm in the UV-vis absorption spectrum (Fig. 4). Only small changes are observed for the other analytes.
When the identical experiment is carried out with ring-open isomer 1o the solutions containing all the potentially interfering analytes retained their bright yellow colour while the solution containing hydrazine became fainter. When exposed to UV light (312 nm) for 60 s, all the solutions turned blue except for the one containing hydrazine, which demonstrates the selectivity of the detection system (Fig. 5).
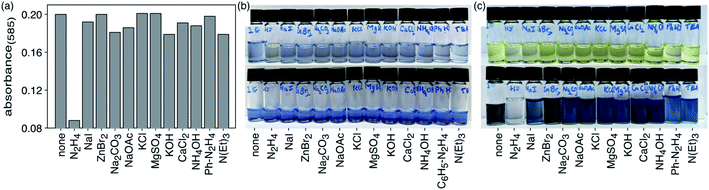 |
| Fig. 5 (a) Absorptivities of DMSO solutions of 1c (4.8 × 10−5 M) after addition of various analytes. (b) Changes in the colour of DMSO solutions of 1c before (top) and after (bottom) addition of various analytes. (c) Changes in the colour of DMSO solutions of before (top) and after (bottom) addition of various analytes and exposure to 312 nm light for 60 s. | |
3. Experimental
3.1 Materials and methods
3.1.1 General. All solvents and reagents used for synthesis, chromatography, UV-vis spectroscopy and photochemical studies were purchased from Aldrich, Anachemia, Caledon Labs, Fisher Scientific or Alfa Aesar, and used as received. Solvents used for metal–halogen exchange reactions were dried and degassed by passing them through steel columns containing activated alumina under nitrogen using an MBraun solvent purification system. Hydrazine monohydrate (64–65% hydrazine‡) was obtained from Aldrich and used as received. Solvents for NMR analysis were purchased from Cambridge Isotope Laboratories and used as received. Column chromatography was performed using silica gel 60 (230–400 mesh) from Silicycle Inc.1H and 13C NMR characterizations were performed on a Bruker Avance-400 instrument with a 5 mm inverse probe operating at 400.13 MHz for 1H NMR and 100.61 MHz for 13C NMR unless stated otherwise. Chemical shifts (δ) are reported in parts per million (ppm) relative to tetramethylsilane using the residual solvent peak as a reference, and splitting patterns are designated as s (singlet), d (doublet), dd (doublet of doubles), t (triplet) and m (multiplet). Coupling constants (J) are reported in Hertz. UV-vis absorption spectra were recorded on a Shimadzu UV-3600 Plus spectrophotometer. Fluorescence measurements were performed on a PTI Quantamaster spectrofluorometer. A JDS Uniphase 980 nm laser diode (device type L4-9897510-100M) coupled to a 105 μm (core) fibre was employed as the excitation source. The output of the diode laser was collimated and directed on the 110 samples using a Newport F-91-C1-T Multimode Fiber Coupler. The visible emissions were collected from the samples at π/2 from the incident beam in the plane of the spectrometer. All of the samples were held in a square quartz cuvette (path length of 1 cm). All spectra were corrected for instrument sensitivity. IR spectroscopy measurements were conducted on PerkinElmer Spectrum Two™ IR spectrometer equipped with universal ATR accessory with a 9-bounce diamond top-plate. High Resolution Mass Spectroscopy (HRMS) measurements were performed using an Agilent 6210 TOF LC/MS in ESI-(+) mode. Microanalysis (C, H, N) was carried out using a Carlo Erba EA 1110 CHN Elemental Analyser. Melting points were measured using a Gallenkamp melting point apparatus and are uncorrected.
3.1.2 Photochemistry. All the ring-closing reactions were carried out using the light source from a lamp used for visualizing TLC plates at 312 nm (Spectroline E series, 470 W cm−2). The ring-opening reactions were carried out using the light of a 150 W halogen photo-optic source passed through a 435 nm cut-off filter to eliminate higher energy light.
3.2 Synthetic methods
Only the final step in Scheme 2 is described below. All other compounds were prepared according to the literature without modifications.57,58 The analysis of all compounds matched those in the literature.
3.2.1 Synthesis of 2,3-bis(2-methyl-5-phenylthiophen-3-yl) maleonitrile (1o). A solution of 2-(2-methyl-5-phenylthiophen-3-yl) acetonitrile (7) (0.93 g, 2.2 mmol) in CCl4 (5 mL) was added to a stirring solution of 50% aqueous NaOH (5 mL) containing tetrabutylammonium bromide (35 mg, 0.11 mmol) at room temperature. The mixture was stirred at 50 °C for 1.5 h and the reaction progress was monitored by TLC (10
:
1 hexanes/EtOAc). Upon completion, the reaction mixture was poured into water and extracted with chloroform (2 × 10 mL), dried over MgSO4, filtered and concentrated under reduced pressure. Purification by column chromatography using silica gel (10
:
1 hexanes/EtOAc) afforded 735 mg (79%) of the product as a yellow solid.1H NMR (400 MHz, CDCl3): δ 7.40 (d, J = 8.1 Hz, 3H), 7.33 (d, J = 7.2 Hz, 2H), 7.29 (d, J = 7.1 Hz, 1H), 6.91 (s, 1H), 2.31 (s, 3H).
13C NMR (101 MHz, CDCl3): δ 142.4, 133.1, 131.8, 129.4, 128.6, 127.5, 126.0, 121.1, 116.4, 15.0.
FT-IR (diamond ATR):
(cm−1) 2924–2851 (C–H stretch, aromatic), 2210 (C
N stretch), 1445 (C–C stretch, aromatic), 755 (C–H bend, oop), 690 (C–S stretch, thiophene).
Melting point: 132–133 °C.
HRMS (ESI): m/z (M + H) calculated for C26H18N2S2: 423.098417, found: 423.097986.
3.2.2 Photochemical synthesis of ring-closed isomer 1c. A CDCl3 solution of compound 1o (5 × 10−3 M) in an NMR tube was irradiated with 312 nm light until no further changes were observed in the 1H NMR spectrum. After 120 min the photostationary state was reached at this concentration, which contained 51% of the ring-closed isomer 1c as measured by comparing relative integrals for chemical shifts for 1o and 1c. The ring-closed isomer was isolated by column chromatography using silica gel (2
:
1 CH2Cl2/hexanes).1H NMR (400 MHz, CDCl3): δ 7.58 (d, J = 6.8 Hz, 2H), 7.46 (d, J = 6.8 Hz, 2H), 7.44 (d, J = 6.7 Hz, 1H), 6.72 (s, 1H), 2.17 (s, 3H).
13C NMR (101 MHz, CDCl3): δ 170.72, 144.69, 130.82, 129.20, 127.25, 115.62, 108.90, 99.58, 57.19, 29.87.
FT-IR (diamond ATR):
(cm−1) 2924–2851 (C–H stretch, aromatic), 2210 (C
N stretch), 1445 (C–C stretch, aromatic), 755 (C–H bend, oop), 690 (C–S stretch, thiophene).
3.2.3 Preparation of compound 2o using hydrazine. A solution of 1c (5 mg, 0.012 mmol, 1 equivalent) in DMSO (20 mL) was treated with N2H4·H2O (2 mL, 21 mmol N2H4, excess). The reaction mixture was stirred at room temperature for 16 h and reaction progress was monitored by TLC (15% ethyl acetate in hexanes). Upon completion, the contents were washed with brine and extracted with ethyl acetate (2 × 20 mL) dried over MgSO4, filtered and concentrated under reduced pressure. Purification by column chromatography using silica gel (15% ethyl acetate in hexanes) afforded 4 mg (78% yield) of 2o as a yellow-brown solid.1H NMR (600 MHz, CDCl3): δ 7.68–7.64 (m, 2H), 7.42 (d, J = 9.0 MHz, 3H), 7.10 (s, 1H), 2.11 (s, 3H).
13C NMR (151 MHz, CDCl3): δ 153.24, 133.77, 132.27, 129.14, 129.07, 128.71, 128.62, 127.87, 125.75, 125.60, 117.81, 14.72.
FT-IR (diamond ATR):
(cm−1) 3319–3076 (N–H stretch), 2924 (C–H stretch, aromatic), 1625 (N–H bend), 1445 (C–C stretch, aromatic), 1160 (C–N stretch), 755 (C–H bend, oop), 690 (C–S stretch, thiophene).
Melting point: 110–113 °C.
Elemental analysis: C = 68.22%, H = 5.32%, N = 8.84%, S = 14.47%.
Conclusions
In this manuscript, we have described the use of a coloured photoresponsive molecule to conveniently detect toxic levels of hydrazine. The combination of the disappearance of colour and generation of bright emission provides two ways to ensure reliability. Our detector also consumes the hydrazine as it changes its optoelectronic properties, which can be considered as a hydrazine trap if applied to large surface area materials. The advantages of our system are based on a distinct colour change with excellent selectivity (see ESI† for a table of comparative systems).
Author contributions
B. K. conducted all the experimentation, characterization, prepared the original draft and worked on the revisions. R. R. worked on investigation and validation of the photochemistry experiments and preparation of the first draft. B. K. formulated the idea of the project and developed the methodology. N. R. B. worked on all drafts of the manuscript and supervised the overall project.
Conflicts of interest
There are no conflicts to declare.
Acknowledgements
This research was supported by the Natural Sciences and Engineering Research Council (NSERC) of Canada and the Canada Research Chairs Program. This work made use of 4D LABS shared facilities supported by the Canada Foundation for Innovation (CFI), British Columbia Knowledge Development Fund (BCKDF) and Simon Fraser University.
Notes and references
- R. Fletcher-Wood, Magnificent molecules: Hydrazine, https://edu.rsc.org/magnificent.molecules/hydrazine/2000023.article, 2016 Search PubMed.
- L. Cui, C. Ji, Z. Peng, L. Zhong, C. Zhou, L. Yan, S. Qu, S. Zhang, C. Huang, X. Qian and Y. Xu, Anal. Chem., 2014, 86, 4611–4617 CrossRef CAS PubMed.
- D. P. Elder, D. Snodin and A. Teasdale, J. Pharm. Biomed. Anal., 2011, 54, 900–910 CrossRef CAS PubMed.
- B. Roy and S. Bandyopadhyay, Anal. Methods, 2018, 10, 1117–1139 RSC.
- K. H. Nguyen, Y. Hao, W. Chen, Y. Zhang, M. Xu, M. Yang and Y. N. Liu, Luminescence, 2018, 33, 816–836 CrossRef.
- U.S.E.P. Agency, Hydrazine, 2016 Search PubMed.
- U.S. Department of Health and Human Services, Occupational Safety and Health Guideline for Hydrazine Potential Human Carcinogen, 1988 Search PubMed.
- S. Parodi, S. De Flora, M. Cavanna, A. Pino, L. Robbiano, C. Bennicelli and G. Brambilla, Cancer Res., 1981, 41, 1469–1482 CAS.
- M. J. Zeilmaker, M. J. Horsfall, J. B. van Helten, B. W. Glickman and G. R. Mohn, Mol. Carcinog., 1991, 4, 180–188 CrossRef CAS PubMed.
- M. Byers and C. Byers, Polar Rec., 2017, 53, 580–591 CrossRef.
- S. Satsangi, Hydrazine Market by Application (Pharmaceuticals, Water Treatment, Blowing Agents, Agrochemicals, and Others) – Global Opportunity Analysis and Industry Forecast, 2014–2022, www.alliedmarketresearch.com/hydrazine-market, accessed 13 April 2021 Search PubMed.
- Hydrazine Market Size, Share & Trends Analysis Report By Application, Regional Outlook, Competitive Strategies, And Segment Forecasts, 2019 to 2025, www.grandviewresearch.com/industry-analysis/hydrazine-market, accessed 13 April 2021 Search PubMed.
- J. R. Stetter, K. F. Blurton, A. M. Valentine and K. A. Tellefsen, J. Electrochem. Soc., 1978, 125, 1804–1807 CrossRef CAS.
- N. M. Ratcliffe, Anal. Chim. Acta, 1990, 239, 257–262 CrossRef CAS.
- J. Liu, W. Zhou, T. You, F. Li, E. Wang and S. Dong, Anal. Chem., 1996, 68, 3350–3353 CrossRef CAS.
- C. Batchelor-McAuley, C. E. Banks, A. O. Simm, T. G. J. Jones and R. G. Compton, Analyst, 2006, 131, 106–110 RSC.
- A. Umar, M. M. Rahman, S. H. Kim and Y. B. Hahn, Chem. Commun., 2008, 166–168 RSC.
- J. Liu, Y. Li, J. Jiang and X. Huang, Dalton Trans., 2010, 39, 8693–8697 RSC.
- M. Sun, L. Bai and D. Q. Liu, J. Pharm. Biomed. Anal., 2009, 49, 529–533 CrossRef CAS PubMed.
- H. Bhutani, S. Singh, S. Vir, K. K. Bhutani, R. Kumar, A. K. Chakraborti and K. C. Jindal, J. Pharm. Biomed. Anal., 2007, 43, 1213–1220 CrossRef CAS PubMed.
- T. Kean, J. H. M. B. Miller, G. G. Skellern and D. Snodin, Pharmeuropa Scientific Notes, 2006, pp. 23–33 Search PubMed.
- T. Miura, Y. Urano, K. Tanaka, T. Nagano, K. Ohkubo and S. Fukuzumi, J. Am. Chem. Soc., 2003, 125, 8666–8671 CrossRef CAS.
- X. Jiang, M. Shangguan, Z. Lu, S. Yi, X. Zeng, Y. Zhang and L. Hou, Tetrahedron, 2020, 76, 1–6 Search PubMed.
- X. Jin, C. Liu, X. Wang, H. Huang, X. Zhang and H. Zhu, Sens. Actuators, B, 2015, 216, 141–149 CrossRef CAS.
- S. W. Thomas and T. M. Swager, Adv. Mater., 2006, 18, 1047–1050 CrossRef CAS.
- X. Guo, S. Li, S. Mu, Y. Zhang, X. Liu and H. Zhang, Spectrochim. Acta, Part A, 2020, 226, 117625 CrossRef CAS PubMed.
- M. Li, H. Chen, X. Liu, Y. Wang, N. Zhang and K. Zheng, Tetrahedron Lett., 2019, 60, 151219 CrossRef CAS.
- Y. Li, X. Wu, S. Yang, S. Liang, H. Tian and B. Sun, Anal. Sci., 2020, 36, 323–327 CrossRef CAS PubMed.
- L. Xiao, J. Tu, S. Sun, Z. Pei, Y. Pei, Y. Pang and Y. Xu, RSC Adv., 2014, 4, 41807–41811 RSC.
- B. Roy, S. Halder, A. Guha and S. Bandyopadhyay, Anal. Chem., 2017, 89, 10625–10636 CrossRef CAS PubMed.
- J. Fan, W. Sun, M. Hu, J. Cao, G. Cheng, H. Dong, K. Song, Y. Liu, S. Sun and X. Peng, Chem. Commun., 2012, 48, 8117–8119 RSC.
- S. Goswami, S. Paul and A. Manna, RSC Adv., 2013, 3, 18872–18877 RSC.
- H. Xu, Z. Huang, Y. Li, B. Gu, Z. Zhou, R. Xie, X. Pang, H. Li and Y. Zhang, Analyst, 2018, 143, 4354–4358 RSC.
- J. Ma, J. Fan, H. Li, Q. Yao, J. Xia, J. Wang and X. Peng, Dyes Pigm., 2017, 138, 39–46 CrossRef CAS.
- W. Xu, X. Li, J. Yin, M. Han, W. Liu, Y. Yang and W. Li, J. Photochem. Photobiol., A, 2020, 390, 112262 CrossRef CAS.
- Y. Jung, I. G. Ju, Y. H. Choe, Y. Kim, S. Park, Y. M. Hyun, M. S. Oh and D. Kim, ACS Sens., 2019, 4, 441–449 CrossRef CAS PubMed.
- K. Vijay, C. Nandi and S. D. Samant, RSC Adv., 2014, 4, 30712–30717 RSC.
- J. Liu, T. Li, S. Wang, Q. Qi, H. Song, Z. Li, L. Yang and W. Huang, RSC Adv., 2020, 10, 5572–5578 RSC.
- M. Qin, Y. Huang, F. Li and Y. Song, J. Mater. Chem. C, 2015, 3, 9265–9275 RSC.
- M. Irie, Chem. Rev., 2000, 100, 1685–1716 CrossRef CAS PubMed.
- B. L. Feringa in Molecular Switches ed. B. Feringa, Wiley-VCH Verlag GmbH, Weinheim, 2001 Search PubMed.
- M. Irie in Organic Photochromic and Thermochromic Compounds Main Photochromic Families ed. J. C. Crano and R. J. Guglielmetti, Springer US, New York, 2002, pp. 207–222 Search PubMed.
- Z. Li, Y. Song, Y. Dai, Y. Pei, Z. Lu and H. Guo, Opt. Mater., 2019, 95, 109235 CrossRef CAS.
- Z. Li, Y. Pei, S. Hou, Y. Dai, D. Liu, J. Zhu, Y. P. Zhu and X. Liu, Dyes Pigm., 2020, 179, 108419 CrossRef CAS.
- Q. Zou, X. Li, J. Zhang, J. Zhou, B. Sun and H. Tian, Chem. Commun., 2012, 48, 2095–2097 RSC.
- Q. Zou, J. Jin, B. Xu, L. Ding and H. Tian, Tetrahedron, 2011, 67, 915–921 CrossRef CAS.
- S. Huang, Z. Li, S. Li, J. Yin and S. Liu, Dyes Pigm., 2012, 92, 961–966 CrossRef CAS.
- Z. Li, H. Zhang, H. Zhang, Y. Xie, H. Chen, C. He, Y. Wang, H. Ya and H. Guo, J. Chem. Res., 2018, 42, 305–308 CrossRef CAS.
- Z. Li, Y. Song, Y. Dai, Y. Pei, Z. Lu and H. Guo, Opt. Mater., 2019, 95, 109235 CrossRef CAS.
- K. Liu, Y. Wen, T. Shi, Y. Li, F. Li, Y. Zhao, C. Huang and T. Yi, Chem. Commun., 2014, 50, 9141–9144 RSC.
- F. Nourmohammadian, T. Wu and N. R. Branda, Chem. Commun., 2011, 47, 10954–10956 RSC.
- G. Vamvounis and N. Sandery, Aust. J. Chem., 2015, 68, 1723–1726 CrossRef CAS.
- V. Valderrey, A. Bonasera, S. Fredrich and S. Hecht, Angew. Chem., Int. Ed., 2017, 56, 1914–1918 CrossRef CAS.
- C. L. Dickinson, J. K. Williams and B. C. McKusic, J. Org. Chem., 1964, 29, 1915–1919 CrossRef CAS.
- S. M. Hecht, D. Werner, D. Traficante, M. Sundaralingam, P. Prusiner, T. Ito and T. Sakurai, J. Org. Chem., 1975, 40, 1815–1822 CrossRef CAS.
- A.-S. S. H. Elgazwy and M. R. M. Rafaee, Org. Chem.: Curr. Res., 2013, 2, 1–27 Search PubMed.
- M. M. Krayushkin, B. V. Lichitskii, A. P. Mikhalev, B. V. Nabatov, A. A. Dudinov and S. N. Ivanov, Russ. J. Org. Chem., 2006, 42, 860–864 CrossRef CAS.
- M. Ohsumi, T. Fukaminato and M. Irie, Chem. Commun., 2005, 3921–3923 RSC.
- B. Kaur, R. Raza, M. J. Stashick and N. R. Branda, Org. Chem. Front., 2019, 6, 1253–1256 RSC.
- A. D. McNaught and A. Wilkinson, IUPAC Compendium of Chemical Terminology (the ‘Gold Book’), Blackwell Scientific Publications, Oxford, 2nd edn, 1997 Search PubMed.
- U.S. Department of Health and Human Services, Occupational Safety and Health Guideline for Hydrazine Potential Human Carcinogen, 1988 Search PubMed.
- National Research Council in Acute Exposure Guideline Levels for Selected Airborne Chemicals, The National Academies Press, Washington, DC, 2010, vol. 8, DOI:10.17226/12770.
- T. B. Norsten and N. R. Branda, J. Am. Chem. Soc., 2001, 123, 1784–1785 CrossRef CAS PubMed.
- T. Kawai, T. Koshido, Y. Kaneuchi and K. Yoshino, Thin Solid Films, 1996, 273, 195–198 CrossRef CAS.
- M. Irie, T. Fukaminato, K. Matsuda and S. Kobatake, Chem. Rev., 2014, 114, 12174–12277 CrossRef CAS PubMed.
- K. Kasatani, S. Kambe and M. Irie, J. Photochem. Photobiol., A, 1999, 122, 11–15 CrossRef CAS.
- K. Matsuda and M. Irie, J. Photochem. Photobiol., C, 2004, 5, 169–182 CrossRef CAS.
Footnotes |
† Electronic supplementary information (ESI) available. See DOI: 10.1039/d1ra03677g |
‡ WARNING: Hydrazine vapour are toxic upon ingestion or inhalation. Extreme caution must be exercised while handling. |
|
This journal is © The Royal Society of Chemistry 2021 |
Click here to see how this site uses Cookies. View our privacy policy here.