DOI:
10.1039/D1RA03559B
(Paper)
RSC Adv., 2021,
11, 24232-24237
One pot synthesis of aryl nitriles from aromatic aldehydes in a water environment†
Received
7th May 2021
, Accepted 5th July 2021
First published on 9th July 2021
Abstract
In this study, we found a green method to obtain aryl nitriles from aromatic aldehyde in water. This simple process was modified from a conventional method. Compared with those approaches, we used water as the solvent instead of harmful chemical reagents. In this one-pot conversion, we got twenty-five aryl nitriles conveniently with pollution to the environment being minimized. Furthermore, we confirmed the reaction mechanism by capturing the intermediates, aldoximes.
Introduction
Aryl nitriles, which are widely used in medicine, pesticide, liquid crystal material and other fields, are one of the most important intermediates for organic synthesis in the chemical industry.1 Hence, the syntheses of aryl nitriles are fundamentally important in organic chemistry. The syntheses of aryl nitriles mainly include the ammoxidation of methyl aromatics,2,3 the cyanidation of halogenated aromatics,4,5 the dehydrating of arylamides,6 the dehydrating of arylaldoximes7–9 the Sandmeyer reaction of arylamine diazonium salts,10,11 etc. Generally, ammoxidation of methyl aromatics is suitable for the production of large tonnage of nitriles. The direct cyanidation of halogenated aromatics and the Sandmeyer reaction of arylamine diazonium salts require the use of highly toxic cyanides. Comparatively speaking, the preparation of aromatic nitriles by dehydrating aromatic amides and aldehyde oximes is of great significance in both the laboratory and industry.
The preparation of aryl nitriles from aromatic aldehydes by dehydration of aldoxime is mainly through reflux of aldehyde and hydroxylamine hydrochloride in formic acid, acetic acid, N,N-dimethylformamide (DMF), N-methylpyrrolidone (NMP) and other solvents to obtain nitrile directly.12–14 For example, Bose15 reported that aldehydes reacted with hydroxylamine hydrochloride, triethylamine and triphosgene in a pot of chloroform to prepare the corresponding nitriles. Dornan16 transformed aldehyde into nitrile using Cu/TEMPO as catalyst, ammonium hydroxide as solvent and dioxygen (from air) as terminal oxidant. In addition, other researchers also conducted this reaction in ionic liquids, acetohydroxamic acid and aqueous ammonium acetate using Bi(OTf)3, [BMIM(SO3H)][OTf], tetrabutylammonium tribromide, hypervalent iodine(III) and CuO nanoparticles as catalysts.17 Movassagh18 reported a simple and economical procedure, which catalyzed by KF/Al2O3 and treated with hydroxylamine hydrochloride in anhydrous DMF at 100 °C, for the direct transformation from aldehydes into nitriles. However, the yield of product is unsatisfactory in DMF, whose consumption is considerable. Sampath19 prepared various aromatic and aliphatic nitriles in high yields by heating corresponding aldehydes with hydroxylamine hydrochloride in N-methylpyrrolidone at 110–115 °C, but N-methylpyrrolidone is difficult to recycle due to its high boiling point thus the cost is relatively high. Moreover, those reactions are commonly not environmentally friendly. Enthaler20 displayed a zinc-catalyst for the transformation of aldehydes and hydroxylamine hydrochloride to the corresponding nitriles. However, the reaction temperature is up to 100 °C, and the solvent toluene is volatile and toxic. Therefore, a desired solution to synthesize nitriles in a more serviceable and mild way does need to be underway for organic chemistry.
In 1979, Olah reported that aromatic aldehydes reacted with hydroxylamine hydrochloride and sodium formate in anhydrous formic acid to form nitriles.21 The reaction condition is easy to control, the after-treatment is simple and the yield is high. However, formic acid is a corrosive reagent with an offensive odor. Later on, other researchers optimized the method and found that the reaction could also be performed in formic acid with a mass fraction of 88% to 77.5%.22,23 Inspired by this discovery, we proposed to use water instead of some formic acid as solvent, thus reducing the amount of formic acid for environmental protection. Herein we presented our independent findings that various aromatic aldehydes could be converted to aryl nitriles successfully in a one-pot process by heating corresponding aromatic aldehydes with hydroxylamine hydrochloride and sodium acetate in an optimized proportion of formic acid–H2O solution (60%
:
40%) at 80 °C.
Results and discussion
Base and solvent screening
Based on Olah's research, our study initiated with the screening of bases and solvents (Table 1). In order to identify the optimal reaction conditions, 4-chlorobenzaldehyde (2a) and 2, 6-dichlorobenzaldehyde (4a) were chosen as model substrates. During the course of the reactions, we found that the solubility of the raw materials (aldehydes and hydroxylamine hydrochloride) in formic acid was better than that in acetic acid, thereby the yields were high with formic acid as the solvent. Then, we also found the selection of sodium formate or sodium acetate as base indicated similar yields of nitriles after 6 h reaction. Relatively speaking, the effect of solvent on the yield of the reaction was greater than that of base. Given the yield, availability and cost, we chose formic acid as solvent and sodium acetate as base for further investigation.
Table 1 Base and solvent evaluation
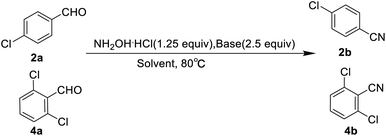
|
Compound |
Solvent |
Base |
Time (h) |
Yielda (%) |
Yield of crude products. |
2b |
HCOOH |
HCOONa |
6 |
70 |
CH3COONa |
6 |
71 |
CH3COOH |
HCOONa |
6 |
5 |
CH3COONa |
6 |
3 |
4b |
HCOOH |
HCOONa |
6 |
92 |
CH3COONa |
6 |
92 |
CH3COOH |
HCOONa |
6 |
68 |
CH3COONa |
6 |
61 |
Solvent optimization
In order to reduce the amount of formic acid, we attempted to replace it with water gradually and the results are shown in Table 2. Considering the solubility of the reactants and the high yields of the products, we selected 1a–5a, 8a, 20a, 21a and 25a as model substrates. All aldehydes could be converted into nitriles with high yields in aqueous formic acid solution (60% formic acid
:
40% water, v/v). To our delight, some aldehydes, such as 2a, 5a, 8a and 21a, could be converted into the corresponding nitriles efficiently even when the water increased to 50%. It was particularly noteworthy that 8a and 21a could be converted to the corresponding aryl nitriles with a total yield of 79% and 69%, respectively, in 40% formic acid
:
60% water solution. Thus, the generally applicable optimal condition here is: 1 equiv. aromatic aldehydes, 1.25 equiv. hydroxylamine hydrochloride, 2.5 equiv. sodium acetate, formic acid (60%) and H2O (40%) stirring at 80 °C and TLC tracking until the aromatic aldehydes disappeared. After the reaction, formic acid could be recycled from decompression spin steaming.
Table 2 The cyanidation of aromatic aldehydes with different proportions of water
Based on the above-optimized condition, we conducted the organic synthesis experiments of aryl nitriles from aromatic aldehydes and finally got twenty-five aryl nitriles 1b–25b. The chemical structures of 1b–25b are shown in Scheme 1 and their reaction time and yields are shown in Table 3.
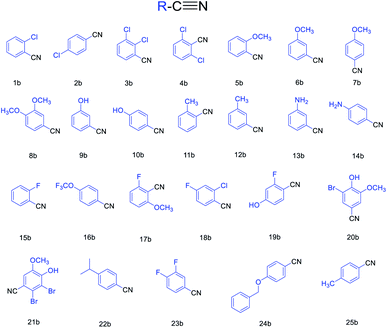 |
| Scheme 1 Substrate scope. | |
Table 3 The cyanidation of aromatic aldehydes in different reaction time

|
Compound |
Time (h) |
Yielda (%) |
Yield of crude products. |
1b |
6 |
56 |
2b |
6 |
78 |
3b |
6 |
78 |
4b |
6 |
91 |
5b |
6 |
77 |
6b |
12 |
68 |
7b |
12 |
65 |
8b |
6 |
86 |
9b |
12 |
89 |
10b |
12 |
52 |
11b |
12 |
91 |
12b |
12 |
74 |
13b |
12 |
65 |
14b |
12 |
70 |
15b |
12 |
74 |
16b |
12 |
63 |
17b |
12 |
65 |
18b |
12 |
68 |
19b |
12 |
45 |
20b |
6 |
70 |
21b |
6 |
82 |
22b |
24 |
43 |
23b |
24 |
54 |
24b |
12 |
80 |
25b |
6 |
56 |
Determination of reaction mechanism and capturing intermediate products
The possible reaction mechanism is shown in Scheme 2. Firstly, aldehydes react with hydroxylamine hydrochloride to afford aldoximes. Subsequently, aldoximes react with formic acid to form ((benzylideneamino)oxy)formaldehydes, which is dehydrated under heating condition to form nitriles. In order to determine the reaction mechanism, we designed different conditions (time, solvent) to capture the intermediates. As listed in Table 4, the shorter the reaction time, the more the intermediate A can be captured. Data shown in Table 5 indicate that the larger the proportion of water, the more intermediates A can be captured. Unfortunately, we failed to capture the intermediates B. In this reaction system, formic acid acted as both solvent and catalyst. According to the solubility differences between aldehydes in formic acid, we could chose different concentrations of formic acid solutions as the solvent of aromatic aldehydes cyanidation to reduce the amount of formic acid, save costs and protect the environment.
 |
| Scheme 2 The mechanism for the production of aryl nitriles from aromatic aldehydes and hydroxylamine hydrochloride by using formic acid aqueous solution as solvent. | |
Table 4 Intermediates captured at various time-points (solvent: 60% formic acid: 40% water, v/v)
Raw material |
Time (h) |
Products |
1a |
4 |
1A |
8 |
1A + 1b |
12 |
1A + 1b |
3a |
4 |
3A |
8 |
3A + 3b |
12 |
3A + 3b |
5a |
4 |
5A |
8 |
5A + 5b |
12 |
5A + 5b |
Table 5 Intermediates capture under various solvent conditions (reaction time: 12 h)
Raw material |
HCOOH/H2O (%) |
Products |
1a |
60 : 40 |
1A + 1b |
50 : 50 |
1A + 1b |
40 : 60 |
1A |
3a |
50 : 50 |
3A + 3b |
40 : 60 |
3A + 3b |
30 : 70 |
3A |
5a |
40 : 60 |
5A + 5b |
30 : 70 |
5A + 5b |
20 : 80 |
5A |
Experimental
General information and materials
Except for benzaldehyde purchased from Shanghai Shaoyuan Co., Ltd, all other chemicals (reagent grade) used were purchased from Nanjing Chemical Reagent Co. Ltd (Nanjing, China). All the 1H NMR spectra were recorded on a Bruker DPX 300 or DRX 500 spectrometer in CDCl3 or d6-DMSO. Chemical shifts (δ) for 1H NMR spectra were reported in ppm (δ). 13C NMR spectra were recorded on a Bruker DPX 600 spectrometer in CDCl3 or d6-DMSO. All the 19F NMR spectra were recorded on a Bruker DPX 400 spectrometer in CDCl3. Melting points (uncorrected) were measured on a XT4 MP Apparatus (Taike Corp., Beijing, China).
General procedure for preparation of compounds 1b–25b
A mixture of aromatic aldehydes (50 mmol) (1a–25a), hydroxylamine hydrochloride (62.5 mmol), sodium formate or sodium acetate (125 mmol) were dissolved in formic acid or acetic acid solution and stirred at 80 °C until TLC analysis indicated the disappearance of aromatic aldehydes. Then, after the reaction system cooled down to room temperature, it was immersed into water to obtain the target compounds. Some desired products dissolved in the formic acid or acetic acid solution could be obtained by salting out. Then, the solid target compounds were obtained by filtration and recrystallized by alcohol, and then dried under vacuum. However, some target compounds were oily. These oily compounds were obtained by extraction with ethyl acetate and the solvent was removed in vacuum to afford aryl nitriles (1b–25b).
Analytical data.
2-Chlorobenzonitrile (1b). Light yellow needle crystal, 56% yield. Mp: 43.6–45.5 °C (ref. mp: 43–45 °C, see ESI†). 1H NMR (500 MHz, CDCl3) δ 7.68 (d, J = 8.2 Hz, 1H), 7.58–7.49 (m, 2H), 7.41–7.34 (m, 1H). 13C NMR (151 MHz, CDCl3) δ 136.84, 134.02, 133.91, 130.05, 127.18, 115.99, 113.36.
4-Chlorobenzonitrile (2b). White flake crystals, 78% yield. Mp: 90.6–92.8 °C (ref. mp: 90–93 °C/92–94 °C/91.2–93.1 °C). 1H NMR (300 MHz, CDCl3) δ 7.60 (d, J = 8.4 Hz, 2H), 7.47 (d, J = 8.5 Hz, 2H). 13C NMR (151 MHz, CDCl3) δ 139.55, 133.40, 129.70, 118.00, 110.75.
2,3-Dichlorobenzonitrile (3b). White needle crystals, 78% yield. Mp: 61.5–62.4 °C (ref. mp: 60–61 °C/56–57 °C/51–53.5 °C). 1H NMR (300 MHz, CDCl3) δ 8.57 (s, 1H), 7.76 (dd, J = 7.9, 1.5 Hz, 1H), 7.49 (dd, J = 8.0, 1.6 Hz, 1H). 13C NMR (151 MHz, DMSO-d6) δ 145.61, 133.72, 133.27, 132.00, 131.11, 129.35, 126.36.
2,6-Dichlorobenzonitrile (4b). White needle crystals, 91% yield. Mp: 143.5–145.0 °C (ref. mp: 144–145 °C/140–143 °C/144–145 °C). 1H NMR (300 MHz, CDCl3) δ 7.52–7.46 (m, 1H), 7.44 (d, J = 4.9 Hz, 2H). 13C NMR (151 MHz, CDCl3) δ 138.49, 133.90, 128.17, 114.38, 113.37.
2-Methoxybenzonitrile (5b). White needle crystals, 77% yield. Mp: 24.5–25.5 °C (ref. mp: 59 °C/21.5–22 °C (ethanol)/24.5 °C). 1H NMR (500 MHz, CDCl3) δ 7.55 (t, J = 7.1 Hz, 2H), 7.04–6.95 (m, 2H), 3.93 (s, 3H). 13C NMR (151 MHz, CDCl3) δ 161.21, 134.53, 133.86, 120.80, 116.38, 111.70, 101.61, 56.03.
3-Methoxybenzonitrile (6b). Colorless transparent liquid, 68% yield. Mp: 20.0–22.0 °C (ref. mp: 24–25 °C/23 °C). 1H NMR (300 MHz, CDCl3) δ 7.42–7.29 (m, 1H), 7.24 (t, J = 7.3 Hz, 1H), 7.13 (s, 2H), 3.82 (t, J = 0.7 Hz, 3H). 13C NMR (151 MHz, CDCl3) δ 159.62, 130.35, 124.48, 119.31, 118.77, 116.83, 113.16, 55.54.
4-Methoxybenzonitrile (7b). White powder, 65% yield. Mp: 57.5–59.5 °C (ref. mp: 58–59 °C/55–57 °C/59.2–60.4 °C/56–58 °C/59–60 °C). 1H NMR (300 MHz, CDCl3) δ 7.58 (d, J = 8.8 Hz, 2H), 6.94 (d, J = 8.8 Hz, 2H), 3.85 (s, 3H). 13C NMR (151 MHz, CDCl3) δ 162.85, 133.99, 119.28, 114.76, 103.89, 55.57.
3,4-Dimethoxybenzonitrile (8b). White crystal, 79% yield. Mp: 67.5–70.5 °C (ref. mp: 68.6–70.5 °C/65–67 °C/66–67 °C/62–65 °C/65–66 °C/64.9–66.4 °C). 1H NMR (300 MHz, CDCl3) δ 7.26 (d, J = 8.3 Hz, 1H), 7.05 (s, 1H), 6.88 (d, J = 8.4 Hz, 1H), 3.91 (s, 3H), 3.88 (s, 3H). 13C NMR (151 MHz, CDCl3) δ 152.84, 149.16, 126.48, 119.26, 113.88, 111.23, 103.85, 56.14, 56.11.
3-Hydroxybenzonitrile (9b). Slightly yellow crystal, 89% yield. Mp: 78.5–80.5 °C (ref. mp: 74.8–77.2 °C/57–59.5 °C/78–80 °C/68 °C/76–78 °C). 1H NMR (300 MHz, CDCl3) δ 8.06 (s, 1H), 7.30 (dd, J = 15.6, 7.7 Hz, 1H), 7.19 (d, J = 7.6 Hz, 1H), 7.13 (d, J = 2.9 Hz, 1H), 6.63 (s, 1H). 13C NMR (151 MHz, CDCl3) δ 156.70, 130.65, 124.51, 121.11, 118.84, 118.72, 112.38.
4-Hydroxybenzonitrile (10b). White crystal, 52% yield. Mp: 110.5–113.0 °C (ref. mp: 110–113 °C/111–112 °C/109–111 °C/108.8–110.2 °C/100.7–103.2 °C/107–109 °C/111–112 °C). 1H NMR (300 MHz, CDCl3) δ 7.61–7.52 (m, 2H), 6.99–6.91 (m, 2H), 6.83 (s, 1H). 13C NMR (151 MHz, DMSO-d6) δ 162.61, 135.22, 120.53, 117.38, 101.96.
2-Methylbenzonitrile (11b). Colorless liquid, 91% yield. 1H NMR (300 MHz, CDCl3) δ 7.56 (d, J = 7.7 Hz, 1H), 7.46 (td, J = 7.6, 1.3 Hz, 1H), 7.34–7.16 (m, 2H), 2.52 (s, 3H). 13C NMR (151 MHz, CDCl3) δ 141.92, 132.66, 132.50, 130.24, 126.23, 118.17, 112.73, 20.47.
3-Methylbenzonitrile (12b). Colorless liquid, 74% yield. 1H NMR (300 MHz, CDCl3) δ 7.46–7.40 (m, 2H), 7.38 (s, 1H), 7.36–7.30 (m, 1H), 2.37 (s, 3H). 13C NMR (151 MHz, CDCl3) δ 139.23, 133.67, 132.48, 129.27, 129.00, 119.06, 112.20, 21.16.
3-Aminobenzonitrile (13b). Brown crystal, 65% yield. Mp: 53.5–54.5 °C (ref. mp: 52–54 °C/50–52 °C/50–54 °C). 1H NMR (300 MHz, CDCl3) δ 7.22 (t, J = 7.8 Hz, 1H), 7.01 (d, J = 7.6 Hz, 1H), 6.89 (t, J = 5.8, 1.7 Hz, 1H), 3.89 (s, 2H). 13C NMR (151 MHz, CDCl3) δ 146.98, 130.06, 121.92, 119.24, 119.21, 117.40, 112.84.
4-Aminobenzonitrile (14b). White crystal, 70% yield. Mp: 83.5–85.0 °C (ref. mp: 83–85 °C/83–86 °C/85–87 °C/85–86 °C). 1H NMR (300 MHz, CDCl3) δ 7.40 (dd, J = 8.8, 2.0 Hz, 2H), 6.64 (d, J = 8.7 Hz, 2H), 4.19 (s, 2H). 13C NMR (151 MHz, DMSO) δ 153.98, 134.43, 121.68, 114.42, 96.49.
2-Fluorobenzonitrile (15b). Colorless transparent liquid, 74% yield. 1H NMR (300 MHz, CDCl3) δ 7.57–7.66 (m, 2H), 7.31 (d, J = 3.0 Hz, 1H), 7.22 (dd, J = 18.0, 9.0 Hz, 1H). 13C NMR (151 MHz, CDCl3) δ 164.13, 162.17, 135.08, 133.57, 124.87, 116.58, 113.90, 101.27.
4-(Trifluoromethoxy)benzonitrile (16b). Colorless liquid, 63% yield. 1H NMR (300 MHz, CDCl3) δ 7.76–7.68 (m, 2H), 7.35–7.28 (m, 2H). 13C NMR (151 MHz, CDCl3) δ 152.36, 134.07, 121.19, 117.73, 110.84.
2-Fluoro-6-methoxybenzonitrile (17b). White crystal, 65% yield. Mp: 103.5–105.0 °C (ref. mp: 93–95 °C). 1H NMR (300 MHz, CDCl3) δ 7.52 (td, J = 8.5, 6.6 Hz, 1H), 6.79 (t, J = 8.1 Hz, 2H), 3.96 (s, 1H). 13C NMR (151 MHz, CDCl3) δ 164.84, 163.14, 162.39, 135.14, 111.63, 108.16, 106.97, 91.77, 56.70.
2-Chloro-4-fluorobenzonitrile (18b). White crystal, 68% yield. Mp: 64.5–66.0 °C (ref. mp: 63.0–65.0 °C). 1H NMR (300 MHz, chloroform-d) δ 7.70 (dd, J = 8.7, 5.6 Hz, 1H), 7.28 (dd, J = 8.0, 2.3 Hz, 1H), 7.12 (ddd, J = 8.7, 7.7, 2.5 Hz, 1H). 13C NMR (151 MHz, CDCl3) δ 165.61, 163.88, 138.76, 135.70, 118.08, 115.28, 115.21, 109.78.
2-Fluoro-4-hydroxybenzonitrile (19b). White crystal, 45% yield. Mp: 123.5–125.0 °C (ref. mp: 124–125 °C/123.5 °C/117–118 °C). 1H NMR (300 MHz, CDCl3) δ 7.55–7.44 (m, 1H), 6.87 (s, 1H), 6.74 (m, 2H). 13C NMR (151 MHz, DMSO-d6) δ 165.32, 164.36, 163.70, 135.22, 115.15, 113.42, 104.00, 90.25.
3-Bromo-4-hydroxy-5-methoxybenzonitrile (20b). White crystals, 60% yield. Mp: 143.0–146.0 °C (ref. mp: 159–160 °C/134–136 °C/144 °C). 1H NMR (300 MHz, DMSO-d6) δ 7.61 (d, J = 1.8 Hz, 1H), 7.38 (d, J = 1.8 Hz, 1H), 3.84 (s, 3H). 13C NMR (151 MHz, DMSO-d6) δ 149.16, 148.73, 129.50, 118.74, 114.90, 109.85, 102.53, 57.12.
2,3-Dibromo-4-hydroxy-5-methoxybenzonitrile (21b). Gray crystals, 80% yield. Mp: 212.0–215.0 °C (ref. mp: 179 °C). 1H NMR (300 MHz, CDCl3) δ 6.80 (s, 1H), 4.12 (s, 3H).
4-Isopropylbenzonitrile (22b). Colorless liquid, 43% yield. 1H NMR (300 MHz, CDCl3) δ 7.56 (d, J = 8.2 Hz, 2H), 7.31 (d, J = 8.2 Hz, 2H), 2.95 (p, J = 6.9 Hz, 1H), 1.25 (d, J = 6.9 Hz, 6H). 13C NMR (151 MHz, CDCl3) δ 154.47, 132.19, 127.25, 119.05, 109.55, 34.35, 23.27.
3,4-Difluorobenzonitrile (23b). White flake crystals, 54% yield. Mp: 52.0–54.0 °C (ref. mp: 51–53 °C). 1H NMR (300 MHz, CDCl3) δ 8.04–7.85 (m, 2H), 7.37–7.27 (m, 1H). 13C NMR (151 MHz, CDCl3) δ 170.66, 155.09, 153.34, 150.84, 149.33, 127.61, 126.10, 119.68, 117.48.
4-(Benzyloxy)benzonitrile (24b). White powder, 80% yield. Mp: 93.0–95.0 °C (ref. mp: 86 °C/91–93 °C/86 °C/86–88 °C/97–99 °C/93–94 °C/96–98 °C). 1H NMR (500 MHz, CDCl3) δ 7.53 (d, J = 8.6 Hz, 2H), 7.46–7.30 (m, 5H), 6.99 (d, J = 8.6 Hz, 2H), 5.10 (s, 2H). 13C NMR (151 MHz, DMSO-d6) δ 159.66, 159.35, 148.08, 144.65, 137.24, 132.76, 128.93, 128.31, 128.21, 126.31, 115.63, 114.89, 69.79.
4-Methylbenzonitrile (25b). Colorless crystal, 56% yield. Mp: 28.0–30.0 °C (ref. mp: 26–28 °C/26 °C/27–29 °C/28–29 °C/26–27 °C/27.1–27.7 °C). 1H NMR (500 MHz, CDCl3) δ 7.56 (d, J = 8.1 Hz, 2H), 7.29 (d, J = 7.9 Hz, 2H), 2.44 (s, 3H). 13C NMR (151 MHz, CDCl3) δ 143.75, 132.00, 129.87, 119.16, 109.25, 21.91.
Conclusion
In summary, we optimized the method that aromatic aldehydes react with hydroxylamine hydrochloride and sodium formate in anhydrous formic acid to form nitriles. We used water instead of some formic acid as solvent to reducing the amount of formic acid for environmental protection. We found that most aromatic aldehydes could be converted to aryl nitriles efficiently in an optimized proportion of formic acid–H2O solution (60%
:
40%). Some aromatic aldehydes can even react in formic acid–H2O solution (30%
:
70%) to form corresponding nitriles. Mechanically specking, formic acid acts as both catalyst and solvent in this reaction. Therefore, we can choose appropriate proportion of formic acid solution as solvent to obtain nitrile according to the characteristics of different aromatic aldehydes.
Conflicts of interest
There are no conflicts to declare.
Acknowledgements
This research was supported by the National Natural Science Foundation of China (NSFC) (No. 21702100, 21907051, U1903201), the Program for Changjiang Scholars and Innovative Research Team in University (No. IRT_14R27), and the Fundamental Research Funds for the Central Universities (No. 020814380140, 020814380151).
Notes and references
- Y. X. Zhang, X. Xiao and Z. H. Fu, et al. Difluorocarbene-Based Cyanation of Aryl Iodides., Synlett, 2020, 31(7), 713–717 CrossRef CAS.
- C. P. Kumar, K. R. Reddy and V. V. Rao, et al. Vapour phase ammoxidation of toluene over vanadium oxide supported on NB2O5–TiO2, Green Chem., 2002, 4(5), 513–516 RSC.
- S. Zhou, K. Junge and D. Addis, A general and convenient catalytic synthesis of nitriles from amides and silanes, Org. Lett., 2010, 40(42), 2461 Search PubMed.
- P. E. Maligres, M. S. Waters, F. Fleitz and D. Askin, A highly catalytic palladium catalyzed cyanation of aryl bromides, Tetrahedron Lett., 2000, 31(5), 8193–8195 Search PubMed.
- P. Anbarasan, T. Schareina and M. Beller, Recent developments and perspectives in palladium-catalyzed cyanation of aryl halides: synthesis of benzonitriles, Chem. Soc. Rev., 2011, 40, 5049–5067 RSC.
- K. J. Hwang, J. P. O'Neil, J. A. Katzenellenbogen and J. A. Katzenellenbogen, 5, 6, 11, 12-Tetrahydrochrysenes: Synthesis of Rigid Stilbene Systems Designed to be Fluorescent Ligands for the Estrogen Receptor, J. Org. Chem., 1992, 57(4), 1262–1271 CrossRef CAS.
- N. D. Kokare and D. B. Shinde, Efficient conversion of aldoximes to nitriles using phosphoric acid diethylester 2-phenylbenzimi-dazol-1-ylester, Monatsh. Chem., 2009, 140, 185–188 CrossRef CAS.
- P. Supsana, T. Liaskopoulos, P. Tsoungas and G. Varvounis, DMF-Catalysed Thermal Dehydration of Aldoximes: A Convenient Access to Functionalized Aliphatic and Aromatic Nitriles, Synlett, 2007, 17, 2671–2674 Search PubMed.
- D. Li, F. Shi, S. Guo and Y. Deng, Highly efficient Beckmann rearrangement and dehydration of oximes, Tetrahedron Lett., 2005, 46, 671–674 CrossRef CAS.
- M. A. K. Zarchi and S. Z. Mousavi, Diazotization-bromination of aromatic amines using polymer-supported bromide via Sandmeyer-type reaction, J. Polym. Res., 2014, 21, 330–338 CrossRef.
- F. Mo, D. Qiu, Y. Zhang and J. Wang, Renaissance of Sandmeyer-Type Reactions Conversion of Aromatic C-N Bonds into C-X Bonds (X = B, Sn, P, or CF), Acc. Chem. Res., 2018, 51(2), 496–506 CrossRef CAS PubMed.
- K. Yamaguchi, H. Fujiwara and Y. Ogasawara, et al. A tungsten-tinmixed hydroxide as an efficient heterogeneous catalyst for dehydration of aldoximes to nitriles, Angew Chem. Int. Ed. Engl., 2007, 46(21), 3922–3925 CrossRef CAS PubMed.
- S. T. Chill and R. C. Mebane, A facile one-pot conversion of aldehydes into nitriles, Synth. Commun., 2009, 39, 3601–3606 CrossRef CAS.
- J. L. Zhu, F. Y. Lee and J. D. Wu, et al. An efficient new procedure for the one-pot conversion of aldehydes into the corresponding nitriles, Synlett, 2007, 8, 1317–1319 CrossRef.
- D. S. Bose and P. R. Goud, Bis (trichloromethyl) carbonate, an efficient activator for the one-pot conversion of aldehydes into nitriles under mild conditions, Synth. Commun., 2002, 32, 3621–3624 CrossRef CAS.
- L. M. Dornan, Q. Cao and J. C. A. Flanagan, et al. Copper/TEMPO catalysed synthesis of nitriles from aldehydes or alcohols using aqueous ammonia and with air as the oxidant, Chem. Commun., 2013, 49(54), 6030–6032 RSC.
- G. C. Nandi and K. K. Laali, Schmidt reaction in ionic liquids: highly efficient and selective conversion of aromatic and heteroaromatic aldehydes to nitriles with [BMIM(SO3H)][OTf] as catalyst and [BMIM][PF6] as solvent, Tetrahedron Lett., 2013, 54(17), 2177–2179 CrossRef CAS.
- B. Movassagh and S. Shokri, An Efficient and Convenient KF/Al2O3-Mediated Synthesis of Nitriles from Aldehydes, Cheminform, 2006, 37(2), 6923–6925 Search PubMed.
- K. H. M. Sampath, R. B. V. Subba and R. P. Tirupathi, Efficient One-Pot Preparation of Nitriles from Aldehydes using N-Methyl-pyrrolidone, Cheminform, 1999, 4, 586–587 Search PubMed.
- S. Enthaler, M. Weidauer and F. Schröder, Straightforward zinc-catalyzed transformation of aldehydes and hydroxylamine hydrochloride to nitriles, Tetrahedron Lett., 2012, 53, 882–885 CrossRef CAS.
- G. A. Olah and T. Keumi, Improved one-step conversion of aldehydes into nitriles with hydroxylamine in formic acid solution, Synthesis, 1979, 2, 112–113 CrossRef.
- H. Ghafuri and M. Roshani, Aqueous formic acid: an efficient, inexpensive and environmentally friendly organocatalyst for three-component Strecker synthesis of α-aminonitriles and imines with excellent yields, RSC Adv., 2014, 4(102), 58280–58286 RSC.
- M. R. Winkle and R. C. Ronald, Regioselective metalation reactions of some substituted (methoxymethoxy) arenes, J. Org. Chem., 1982, 47(11), 2101–2108 CrossRef CAS.
Footnote |
† Electronic supplementary information (ESI) available. See DOI: 10.1039/d1ra03559b |
|
This journal is © The Royal Society of Chemistry 2021 |
Click here to see how this site uses Cookies. View our privacy policy here.