DOI:
10.1039/D1RA03379D
(Paper)
RSC Adv., 2021,
11, 20322-20325
Iron porphyrin-catalyzed N-trifluoroethylation of anilines with 2,2,2-trifluoroethylamine hydrochloride in aqueous solution†
Received
4th May 2021
, Accepted 27th May 2021
First published on 7th June 2021
Abstract
An iron porphyrin-catalyzed N-trifluoroethylation of anilines has been developed with 2,2,2-trifluoroethylamine hydrochloride as the fluorine source. This one-pot N–H insertion reaction is conducted via cascade diazotization/N-trifluoroethylation reactions. The developed transformation can afford a wide range of N-trifluoroethylated anilines in good yields using readily available primary amines and secondary anilines as starting materials.
Introduction
The existence of fluorine in organic molecules usually provides favorable properties for these molecules; therefore, the development of new synthetic methods for the introduction of fluorine and fluorous functional groups is an emerging field in synthetic organic chemistry.1–3 N-trifluoroethylated amines are widely used as platform chemicals in the fields of synthetic organic, medicinal and agrochemistry.4–6 To obtain N-trifluoroethylated amines, two approaches have been used: (1) using aryl halides via the reaction with 2,2,2-trifluoroethylamine8–10 and (2) using amines through the reaction with trifluoroethylated reagents such as trifluoroethyl chloride,11 hypervalent-iodine–CH2CF3 reagents,12 trifluoroacetic acid13 and fluoroalcohols.7 Trifluorodiazoethane (CF3CHN2) synthesized from trifluoroethylamine hydrochloride was widely used as an attractive CF3-containing synthon, largely owing to the pioneering work in 1943 from Gilman and Jones.14 Since then, trifluorodiazoethane have been expanded for the synthesis of various fluorinated compounds including N-trifluoroethylated amines.15 The first work on N-trifluoroethylation of anilines using CF3CHN2 as fluoroethylated agent was reported by Wang and co-workers,16 in which Ag(I) complex catalyzed anilines N–H insertion, yielded trifluoroethyl-substituted primary amines (Scheme 1a). In another attempt, Gouverneur and co-workers reported further extension of the insertion reactions of CF3CHN2 into heteroatom-H bonds including N–H bond catalyzed by Cu(I) catalyst (Scheme 1b).17 Despite significant progress in this field, the current methodologies could not afford trifluroethyl-substituted tertiary amines with secondary amines as substrates. Moreover, the available N–H insertion with trifluorodiazoethane as carbene precursor needs handle diazo compounds. The development of new strategies to access N-trifluroethylated amines from readily available primary and secondary anilines in one pot still remains highly desired.
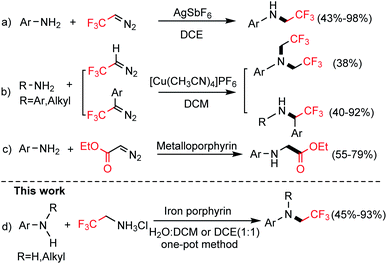 |
| Scheme 1 Transition-metal catalyzed N–H insertion of anilines. | |
The N–H insertions, which are typical in metal-carbene reactions, have been extensively explored with metalloporphyrins as catalysts. For example, metalloporphyrin have been used in N–H insertion with ethyl diazoacetate as carbene precursor (Scheme 1c).18 However, except for our patent,19 metalloporphyrin-based N–H insertion with trifluoroethylamine hydrochloride as the carbene precursor have not been explored to date. In view of the importance of N-trifluoroethylated anilines and also as part of our ongoing interest in metalloporphyrin-based biomimetic catalysis,20–24 herein, we report one-pot method for iron-porphyrin catalyzed N-trifluoroethylation of amines with trifluoroethylamine hydrochloride as the fluorine source in aqueous solution, as described in Scheme 1d.
Results and discussion
We initiated our studies with o-methyl aniline (1a) as the model substrate to screen the optimal reaction conditions and the results are summarized in Table 1. Among the investigated transition-metal catalysts, an iron–porphyrin complex tetraphenylporphyrinatoiron(III) chloride [Fe(TPP)Cl] performed well under the conditions show as note in Table 1, 2-methyl-N-(2,2,2-trifluoroethyl) aniline was afforded as product in 78% yield (Table 1, entry 1). The use of metalloporphyrin catalysts (MnTPPCl, CuTPPCl and CoTPP) as well as Vitamin B12 (VB12) gave trace of products (Table 1, entries 2–5). Iron salts such as FeCl3, FeCl2 were examed and had almost no catalytic activity (Table 1, entries 6 and 7). Two common metal salts used in N-trifluoroethylation reactions AgSbF6 and Cu[(CH3CN)4]PF6 afforded very poor yields (Table 1, entries 8 and 9). These results exhibit the importance of the iron porphyrin for the reaction. Next, it was found that the aqueous solvent plays a key role in the reaction and that H2O
:
CH2Cl2 = 1
:
1 stood out as the best one among the solvents examined (Table 1, entries 1, 10 and 11). In addition, a careful survey of acids revealed that organic acid was better than inorganic acid as catalyst in diazotization reaction, and acetic acid was the best one (Table 1, entries 12–14). It is worth note that the desired reaction could also occurred without any acids (Table 1, entry 15), owing to the participation in diazotization reaction of hydrochloric acid of trifluoroethylated reagents.
Table 1 Optimization of reaction conditionsa

|
Entry |
Catalyst |
Solvent |
Acid |
Temperature [°C] |
Yield [%] |
Reaction conditions: 1a (0.3 mmol, 1.0 equiv.), trifluoroethylamine hydrochloride (0.6 mmol, 2 equiv.), catalyst (0.9 mol%), NaNO2 (0.6 mmol, 2 equiv.), Acid (0.6 mmol, 2 equiv.), solvent (4 mL), air atmosphere, r. t., 12 h. |
1 |
FeTPPCl |
H2O : CH2Cl2 = 1 : 1 |
CH3COOH |
r.t |
78 |
2 |
MnTPPCl |
H2O : CH2Cl2 = 1 : 1 |
CH3COOH |
r.t |
Trace |
3 |
CuTPPCl |
H2O : CH2Cl2 = 1 : 1 |
CH3COOH |
r.t |
Trace |
4 |
CoTPPCl |
H2O : CH2Cl2 = 1 : 1 |
CH3COOH |
r.t |
Trace |
5 |
VB12 |
H2O : CH2Cl2 = 1 : 1 |
CH3COOH |
r.t |
Trace |
6 |
FeCl3 |
H2O : CH2Cl2 = 1 : 1 |
CH3COOH |
r.t |
Trace |
7 |
FeCl2 |
H2O : CH2Cl2 = 1 : 1 |
CH3COOH |
r.t |
Trace |
8 |
AgSbF6 |
H2O : CH2Cl2 = 1 : 1 |
CH3COOH |
r.t |
Trace |
9 |
Cu[(CH3CN)4]PF6 |
H2O : CH2Cl2 = 1 : 1 |
CH3COOH |
r.t |
Trace |
10 |
FeTPPCl |
H2O : toluene = 1 : 1 |
CH3COOH |
r.t |
50 |
11 |
FeTPPCl |
H2O : CH2ClCH2Cl = 1 : 1 |
CH3COOH |
r.t |
67 |
12 |
FeTPPCl |
H2O : CH2Cl2 = 1 : 1 |
HCOOH |
r.t |
70 |
13 |
FeTPPCl |
H2O : CH2Cl2 = 1 : 1 |
HCl |
r.t |
58 |
14 |
FeTPPCl |
H2O : CH2Cl2 = 1 : 1 |
H2SO4 |
r.t |
60 |
15 |
FeTPPCl |
H2O : CH2Cl2 = 1 : 1 |
|
r.t |
62 |
Encouraged by above results, we investigated the scope of the reaction (Table 2). A series of anilines bearing electron-rich (2a–2k) and electron-deficient (2m–2s) groups performed the N-trifluoroethylation reactions in moderate to high yields of 45–93%. Compared with anilines with electron-deficient groups on para position of the phenyl ring, anilines with electron-rich groups favored the N–H insertions (2a–2k), unlike the reaction reported by Wang's group.16 This might because that the carbene complex generated in situ become more prone to attack by the electron-rich amines. Addition two electron-rich substituents on phenyl ring could enhance the yields of products evidently (2h–2k), while no reaction was observed with anilines containing strong electron-withdrawing groups such as –NO2 or –CN. Interestingly, the position of substituents on the benzene ring had a slight effect on the reaction because o-, m- and p-substituted anilines gave similar yields of the desired products (2a–2c, 2d–2f, 2m–2o). Unfortunately, when we explored the 2-tert-butyl aniline as substrate, this reaction did not occur. It suggested that the steric hindrance effects could play an important part in the reactions.
Table 2 Substrate scope of primary anilinesa
Reaction conditions: 1 (0.3 mmol, 1.0 equiv.), trifluoroethylamine hydrochloride (0.6 mmol, 2 equiv.), FeTPPCl (0.9 mol%), NaNO2 (0.6 mmol, 2 equiv.), CH3COOH (0.6 mmol, 2 equiv.), H2O : CH2Cl2 = 1 : 1 (4 mL), air atmosphere, r. t., 12 h. |
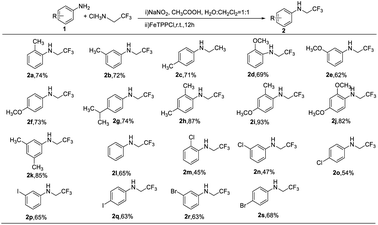 |
The relative broad scope of this method encouraged further studies on the N-trifluoroethylation of secondary anilines, which could not be applied in the works by Wang's and Gouverneur's groups.16,17 Firstly, we examined N-methylaniline as the model of secondary amine substrate under the standard condition. However, the yield of the desired product was only 30% (see the ESI, S2 entry 1†). When we increase the reaction temperature and use the higher boiling point solvent (DCE), the yield of desired products also increased (see the ESI, S2 entries 2–4†). The optimal temperature is 80 °C. Next, several N-methylanilines were expanded and the results are shown in Table 3. The N-trifluoroethylation reactions have shown excellent tolerance to both electron-withdrawing and electron-donating groups on the m- and p-position of phenyl rings (4b–4g) with good yields of 70–75%. Similarly, this reaction seems to be sensitive to steric effects, because no reaction was observed with 4-bromo-N-isopropylaniline and 2-methoxy-N-methylaniline as substrates. Regrettably, with N-ethylaniline as substrates, the yield of desired product reduced a lot, and even only trace of product was detected with N-isopropyl-aniline as substrate.
Table 3 Substrate scope of secondary anilinesa
Reaction conditions: 3 (0.3 mmol, 1.0 equiv.), trifluoroethylamine hydrochloride (0.6 mmol, 2 equiv.), FeTPPCl (0.9 mol%), NaNO2 (0.6 mmol, 2 equiv.), CH3COOH (0.6 mmol, 2 equiv.), H2O : CH2ClCH2Cl = 1 : 1 (4 mL), air atmosphere, 80 °C, 12 h. |
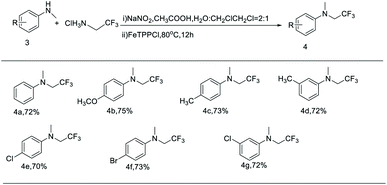 |
With the substrate scopes in hand, we further explore the catalytic mechanism of iron porphyrin-catalyzed N-trifluoroethylation reaction. According to Castro and Ford's reports,25,26 iron(III) porphyrins could be easily converted to a ferrous nitrosyl complex (FeTPP (NO)) in presence of nitrate and acid. We wonder if this ferrous nitrosyl intermediate also exists in our catalytic system, so we tried to synthesize and characterized it with ESR, shown in Fig. 1 and 2 (see the ESI S2†). Three g value of the ESR spectrum of FeTPP (NO) are observed at 2.09, 2.05, and 2.0 under 77 k test, and g value of the ESR spectrum of FeTPP (NO) are observed at 2.05 under room temperature test, which are similar with that reported in the literature.27,28 This result proves that FeTPP (NO) was indeed synthesized.
After that, we tried to utilize FeTPP (NO) instead of FeTPPCl to catalyze the N-trifluoroethylation of o-methyl aniline, shown in Scheme 2. 83% yield of 2-methyl-N-(2,2,2-trifluoroethyl) aniline was obtained. This result suggests the formation of FeTPP (NO) in this reaction.
 |
| Scheme 2 FeTPP (NO)-catalyzed N–H insertion of o-methyl aniline. | |
On the basis of the above-mentioned results and the literature,3,18,29 we proposed a similar reaction mechanism to that of iron porphyrin-catalyzed N-trifluoroethylation reaction (Scheme 3). Iron porphyrin reacts with sodium nitrite to produce Fe(III)TPP (NO2), which can react with reducing substances to produce ferrous nitrosyl complex Fe(II)TPP (NO) under weak acid conditions. Fe(II)TPP (NO) is particularly susceptible to the attack of trifluoromethyl diazomethane formed by the reaction of sodium nitrite and trifluoroethylamine hydrochloride. The ferrous nitrosyl intermediate then forms an iron-carbene intermediate, which is sensitive to nucleophilic attack by an aniline. Finally, N-trifluoroethylated amine is generated and the active catalyst is recycled.
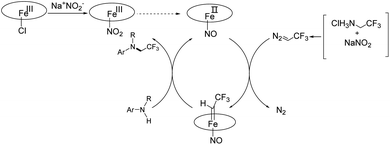 |
| Scheme 3 Potential routes for iron porphyrin-catalyzed N-trifluoroethylation in water. | |
Experimental
General procedure for N-trifluoroethylation
To an oven-dried Schlenk tube was added 2,2,2-trifluoroethylamine hydrochloride (81.3 mg, 0.6 mmol), acetic acid (36.0 mg, 0.6 mmol), 2 mL H2O and 1 mL dichloromethane under an air atmosphere at room temperature. NaNO2 (41.4 mg, 0.6 mmol) was added into the Schlenk tube. The mixed solution was stirred at room temperature for half an hour. After that, o-methyl aniline (32.2 mg, 0.3 mmol) and Fe(TPP)Cl (2 mg, 0.9% mol) was added into the vigorously stirred aqueous solution at room temperature. After 12 hours, the crude product was extracted three times with ethyl acetate and purified by column chromatography on silica gel to afford the product in order to analyze NMR.
Ferrous nitrosyl complex (FeTPP(NO)) was synthesized according to literature,28 and characterized by electron spin resonance spectroscopy (ESR).
Conclusions
In summary, we have developed a method of N-trifluoroethylation of anilines in the presence of an iron(III) porphyrin catalyst with 2,2,2-trifluoroethylamine hydrochloride as the fluorine source. The reaction exhibits good substrate scope (primary and secondary anilines) and functional group compatibility. This new useful protocol (absent inert atmosphere, aqueous solution) should find use in the preparation of N-trifluoroethylated amines under these reaction conditions.
Conflicts of interest
There are no conflicts to declare.
Acknowledgements
This work was financially supported by the National Natural Science Foundation of China (21572049), and Science and Technology Program of Changsha, China.
Notes and references
- J. Wang, M. Sánchez-Roselló, J. L. Aceña, C. del Pozo, A. E. Sorochinsky, S. Fustero, V. A. Soloshonok and H. Liu, Chem. Rev., 2014, 114, 2432 CrossRef CAS PubMed.
- C. Ni, M. Hu and J. Hu, Chem. Rev., 2015, 115, 765 CrossRef CAS PubMed.
- P. K. Mykhailiuk, Chem. Rev., 2021, 121(3), 1670 CrossRef CAS PubMed.
- V. G. Nenajdenko, V. M. Muzalevskiy and A. V. Shastin, Chem. Rev., 2015, 115, 973 CrossRef CAS PubMed.
- S. Purser, P. R. Moore, S. Swallow and V. Gouverneur, Chem. Soc. Rev., 2010, 39, 320 Search PubMed.
- H. Song, Q. Han, C. Zhao and C. Zhang, Green Chem., 2018, 20, 1662 RSC.
- F. Haghighi, F. Panahi, M. G. Haghighi and A. Khalafi-Nezhad, Chem. Commun., 2017, 53, 12650 RSC.
- E. B. Corcoran, M. T. Pirnot, S. Lin, S. D. Dreher and D. A. Dirocco, Aryl amination using ligand-free Ni (II) salts and photoredox catalysis, Science, 2016, 353, 279 CrossRef CAS PubMed.
- A. T. Brusoe and J. F. Hartwig, J. Am. Chem. Soc., 2015, 137, 8460 CrossRef CAS PubMed.
- Y. Kawamata, J. C. Vantourout, D. P. Hickey, P. Bai, L. Chen, Q. Hou, W. Qiao, K. Barman, M. A. Edwards, A. F. Garrido-Castro, J. N. de Gruyter, H. Nakamura, K. Knouse, C. Qin, K. J. Clay, D. Bao, C. Li, J. T. Starr, C. Garcia-Irizarry, N. Sach, H. S. White, M. Neurock, S. D. Minteer and P. S. Baran, J. Am. Chem. Soc., 2019, 141(15), 6392 CrossRef CAS PubMed.
- J. B. Dickey, E. B. Towne, M. S. Bloom, G. J. Taylor, H. M. Hill and R. A. Corbitt, Ind. Eng. Chem., 2002, 46, 2213 CrossRef.
- T. Umemoto and Y. Gotoh, J. Fluorine Chem., 1986, 31, 231 CrossRef CAS.
- K. G. Andrews, R. Faizova and R. M. Denton, Nat. Commun., 2017, 8, 15913 CrossRef PubMed.
- H. Gilman and R. G. Jones, J. Am. Chem. Soc., 1943, 65(8), 1458 CrossRef CAS.
- P. K. Mykhailiuk, Chem. Rev., 2020, 120(22), 12718 CrossRef CAS PubMed.
- H. Q. Luo, G. J. Wu, Y. Zhang and J. B. Wang, Angew. Chem., Int. Ed., 2015, 54, 14503 CrossRef CAS PubMed.
- S. Hyde, J. Veliks, B. Liégault, D. Grassi, M. Taillefer and V. Gouverneur, Angew. Chem., Int. Ed., 2016, 55, 3785 CrossRef CAS PubMed.
- C. Damiano, P. Sonzini and E. Gallo, Chem. Soc. Rev., 2020, 49, 4867 RSC.
- Q. Liu, G. Xu and C. Guo, Chinese Pat., CN108997144A, 2018.
- Q. Liu, D. D. Zhou, Z. X. Li, W. P. Luo and C. C. Guo, Chin. J. Chem., 2017, 35, 1063 CrossRef CAS.
- Q. Liu, X. H. Bai, G. X. Feng, Z. Tan, Q. Jiang and C. C. Guo, BioResources, 2016, 11, 10251 CAS.
- W. P. Luo, J. Sun, J. Ye, Y. Deng, Q. Liu and C. C. Guo, Ind. Eng. Chem., 2014, 20, 3061 CrossRef CAS.
- Q. Jiang, W. B. Sheng, M. Tian, J. Tang and C. C. Guo, Eur. J. Org. Chem., 2013, 10, 1861 CrossRef.
- Q. Liu and C. C. Guo, Sci. China: Chem., 2010, 55(10), 2036–2053 CrossRef.
- K. Stephen, W. Wen, S. Ruth, E. Charles and C. E. Castro, J. Organomet. Chem., 1996, 61(18), 6388 Search PubMed.
- K. Chosu, H. Julie and P. C. Ford, J. Am. Chem. Soc., 2008, 130(42), 13830 CrossRef PubMed.
- T. C. Berto, V. K. K. Praneeth, L. E. Goodrich and N. Lehnert, J. Am. Chem. Soc., 2009, 131(47), 17116 CrossRef CAS PubMed.
- B. B. Wayland and L. W. Olson, J. Am. Chem. Soc., 1974, 96(19), 6037 CrossRef CAS PubMed.
- K. Wu, C. Zhou and C. Che, Org. Lett., 2019, 21(1), 85 CrossRef CAS PubMed.
Footnote |
† Electronic supplementary information (ESI) available. See DOI: 10.1039/d1ra03379d |
|
This journal is © The Royal Society of Chemistry 2021 |
Click here to see how this site uses Cookies. View our privacy policy here.