DOI:
10.1039/D1RA03308E
(Paper)
RSC Adv., 2021,
11, 24985-24990
Microcystis aeruginosa removal by the combination of ultrasound and TiO2/biochar
Received
28th April 2021
, Accepted 7th July 2021
First published on 19th July 2021
Abstract
Harmful cyanobacteria blooms are increasing. They call for novel removal technology, since the required doses of algaecides may cause further environmental pollution or damage treatment facilities. Undesirable intracellular compounds can be released in the water when cyanobacterial cells are damaged. For the first time, ultrasound irradiation was combined with TiO2/biochar (TiO2/BC) at relatively low dosage and tested as an alternative for promoting the coagulation of Microcystis aeruginosa in water treatment. This pre-oxidation process removed 92% of cyanobacterial cells after coagulation. With the combination of ultrasound and TiO2/BC treatment, the dissolved organic carbon and microcystins levels did not increase significantly. The oxidative treatments enhanced the permeability of the cyanobacterial cell membranes, which may be due to the various active species generated from the ultrasound and TiO2/BC process. The results showed that the TiO2/BC hybrid catalyst could be a potential candidate for cyanobacterial cells removal in water.
1. Introduction
The shortage of water resources is a serious issue for modern society. Moreover, in recent years cyanobacteria blooms in lakes and reservoirs have been occurring more frequently. A large number of cyanobacteria cells in surface water increases the difficulty of drinking water treatment.1 In addition, algal organic matter (AOM) such as algal toxins, odorants and extracellular polymer substances may produce disinfection by-products which seriously threaten human health.2 That makes it very important to remove cyanobacteria effectively in water purification without releasing AOM to ensure the safety of drinking water. To counter this, chemical pre-oxidation is often used to enhance the coagulation efficiency for cyanobacteria removal.3,4 For example, hydroxyl radicals OH˙ generated from hydrogen peroxide can oxidize the surface of cyanobacteria cells.5,6 But at certain concentrations these oxidative agents can cause lysis of cyanobacteria cells with the release of toxins.7 The dosage of these chemicals applied should therefore be carefully considered to achieve a balance between efficient removal of cyanobacteria and drinking water safety.
For cyanobacteria removal in water, TiO2 has been considered as a photocatalyst, which could produce radicals for pre-oxidation to enhanced the coagulation efficiency, and thus the coagulant dose was reduced.8,9 But excessive TiO2 nanoparticles in water will have potential harm to the environment and human health. In this regard, as a substrate, carbon based materials could improve the catalytic performance of TiO2, leading to a higher oxidation efficiency, which may reduce the required dose of TiO2 to ensure its safety of environmental application. Alternatively, TiO2 can also be used as a sonosensitizer to enhance the formation of ˙OH radicals by ultrasonic irradiation.10–16 Moreover, Wu et al. reported that the integration of reduced graphene with TiO2 greatly enhanced the production of reactive oxygen species and suggested that the high conductivity of graphene is beneficial to the separation of electron hole pairs, which provides a strategy to enhance the sonocatalytic efficiency of TiO2 based sonosensitizer. On the other hand, biochar (BC) is derived from biomass (organic waste, crop residues, wood or bamboo materials), which is a kind of carbon based material with abundant resources, and can be used as a carbon based substrate. In fact, it has been reported that TiO2/biochar composites can improve the ultrasonic cavitation efficiency and degrade organic compounds since the composite has a large number of active centers, promoting the mass transfer of reactant molecules to the catalyst surface.10 However, it has not been reported whether the combination of ultrasound and TiO2/biochar can be used to promote cyanobacteria removal by coagulation–sedimentation.
Ultrasound is an alternative technology less affected by turbidity of water and it has been investigated for cyanobacteria cells removal via coagulation. In this work, Microcystis aeruginosa were selected as the model blooming cyanobacteria species, and the pre-oxidation effect of ultrasound and TiO2/BC on those cells was explored. The specific objectives were: (1) to synthesize TiO2 loaded BC nanocomposites and evaluate the effectiveness of ultrasound and TiO2/BC treatment in removing the cyanobacteria cells; (2) to evaluate the water quality after treatment, including the concentrations of any algal toxins and dissolved organic carbon; (3) to analyze the population of intact cyanobacteria after the treatments; and (4) to explore the potential of ultrasound and TiO2/BC as pre-oxidation processes in drinking water treatment.
2. Materials and methods
2.1 Microbes and reagents
Microcystis aeruginosa cells were purchased from the Culture Collection of Algae and Protozoa of the Scottish Society for Marine Science. They were cultured in BG11 medium at 25 °C under 12 hour diurnal illumination. Commercial bamboo biochar showing a black color, was purchased from Yangtai Power Co. Ltd. (Yangzhou, China). Butyl titanate, ethanol, acetic acid, hydrogen peroxide was purchased from Yangtai Chemical Reagent Yangzhou Co. Ltd. (China) in analytical grade. They were used without further purification. Distill water was purchased from Yangtai Chemical Reagent Yangzhou Co. Ltd. (China).
2.2 Preparation and characterization of TiO2 loaded BC nanoparticles
The TiO2 loaded BC nanocomposite was synthesized following previous reports.17 10 mL ethanol, 1 mL distilled water and 1 mL acetic acid were mixed and the mixture was stirred and dropwise added into 40 mL butyl titante/ethanol stock (volume ratio 1
:
3), and the mixture was kept away from light for 24 hours. 1 g of BC was dispersed in the obtained mixture. The suspension was stirred intensively and sonicated (40 kHz ultrasonic bath, 55 W) for 30 min at room temperature. Then distilled water drops were added into the mixture during the sonication. The obtained solid was dried in an oven at 105 °C for several hours to obtain black color solid lumps. The solid were then grinded into powder (100–200 mesh), and further fabricate in a tubular furnace under 400 °C with an atmosphere of nitrogen for 2 hours. Thereafter, the resulting sonocatalysts were cooled using nitrogen until reaction temperature drops to room temperature, and finished product was taken out. TiO2 alone was prepared using the same method without the addition of BC.
The morphology of the obtained samples was observed by a Transmission electron microscopy (TEM, JEM-200CX). X-ray diffraction (XRD) analysis was performed using a PRO diffractometer (Cu Kα, λ = 1.541 Å).
2.3 Ultrasound-assisted coagulation
Cells in the exponential growth phase were collected for the experiments and used at a concentration of approximately 1.3 × 107 cells per mL. Tests at that concentration were expected to demonstrate to what extent the treatments could control cyanobacterial growth and damage the cells under algal bloom conditions. For 600 kHz ultrasonic treatment (0.3 Watts per mL), 20 mL cyanobacterial suspension was irradiated for 0, 15, 30, 60 or 90 s. For ultrasound-TiO2/BC treatment, 20 mL samples were treated with different concentrations of catalysts and irradiated for 0, 15, 30, 60 or 90 s.
2.4 Coagulation and sedimentation experiments
After the ultrasound or ultrasound-TiO2/BC pre-oxidation, a coagulation process was performed on the resulting bacterial suspension using 0.5 mg L−1 alum (Al2(SO4)3·18H2O).18 After adding the coagulant, the mixtures were mixed at 300 rpm for 1 min, and then the mixing rate was changed to 42 rpm for 22 min. The suspensions were kept as stationary for 10 min. Water samples were then collected from 2 cm below the surface. The residual Microcystis aeruginosa still suspended were then tested for cell density, cell integrity, dissolved organic carbon (DOC) and microcystins concentration.
2.5 Cell integrity analysis
Approximately 50
000 cells were isolated using flow cytometry (BD FBCS Canto II, Becton Dickinson, Franklin Lakes, USA). An SYTOX Green dye (S-7020; Molecular Probes, Leiden, The Netherlands) was used to measure the percentages of damaged/dead (Syto-X stained) cells in a sample. Using an argon laser (excitation at 488 nm), green fluorescence was recorded from SYTO-X stained samples through a 550 nm band pass filter.
2.6 Water quality measurement
The DOC content was analyzed using a TOC-VCPN analyzer (Shimadzu, Japan). The concentrations of microcystins in the water were measured using enzyme-linked immunosorbent assay ELISA (UNIBIOTEST 96T microcystins ELISA kit, Youbo Biotechnology Co., Ltd, China). The detection limit of the ELISA kit was 0.1 μg L−1.19
2.7 Statistical analysis
Each treatment was conducted with three replications, and the means and standard deviations were computed.
3. Results and discussion
3.1 Characterization of the BC supported TiO2
The surface morphology of the TiO2/BC were demonstrated in Fig. 1. It could be obviously seen that TiO2 dispersed in the surface of flaky BC, with some TiO2 particles were loaded on the BC, indicating that TiO2 nanocomposites were successfully fabricated and anchored on the BC surface. Moreover, C, O and Ti elements could be found in the EDX spectrum of the fabricated nanoparticles. Among the element peaks, the strong peak of Ti confirmed the presence of TiO2 in BC. The mapping results further demonstrated that the Ti dispersed evenly in the TiO2/BC.
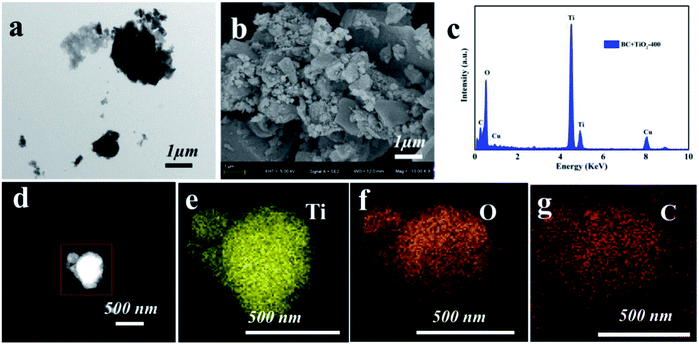 |
| Fig. 1 Characterization of TiO2/BC. (a) TEM image, (b) SEM image, (c) EDS spectrum of (d) with element mapping of Ti (e), O (f) and C (g), respectively. | |
XRD analyses were performed to confirm the crystal structure of TiO2/BC. For pure BC sample, the broad peaks at 2θ = 23° and 43° suggesting the graphitic characteristics of the fabricated BC nanocomposite. Compared with the XRD spectrum of TiO2 alone, there was no new peak or shift in that of TiO2/BC. Both TiO2 and TiO2/BC showed the peaks at 38° (200), 48° (105), 56° and 62° (211), agreeing well with the crystal planes of the anatase phase TiO2. XPS was also performed to investigate the surface chemical composition of the obtained TiO2/BC. The two peaks at 459.4 eV (Ti 2p3/2) and 465.1 eV (Ti 2p1/2) were corresponded with pure TiO2 (Fig. 2).
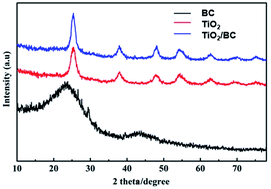 |
| Fig. 2 XRD patterns of BC, TiO2 and TiO2/BC. | |
Our experimental results show that TiO2 with the crystal planes of anatase phase has been successfully prepared. After the combination of TiO2 and BC, TiO2 adheres to the surface of porous BC, and its crystal form is not affected obviously. The advantage of this hybrid system is that TiO2 is an efficient sonocatalyst,10–16 meanwhile porous carbon materials provide a reliable support. Furthermore, the composted sonocatalyst with porous structure can absorb pollutants and improve the degradation rate. TiO2/BC has been used for environmental remediation due to the excellent sonocatalytic performance. But to best of our knowledge, the combination of ultrasound and TiO2/BC has not been investigated on its performance for cyanobacterial removal in water, which is a critical issue for water resource protection as well as drinking water safety.
3.2 M. aeruginosa removal by TiO2/BC sonocatalysis and coagulation process
Fig. 3 shows the removal of M. aeruginosa cells after TiO2/BC sonocatalysis and coagulation. The initial cell concentration in each experiment was approximately 1.3 × 107 cells per mL. With ultrasound irradiation alone, the concentration did not decrease after coagulation (data not shown). Without sonication, the cell concentration showed a slight reduction using 5 mg L−1 TiO2/BC. When the TiO2/BC dose was increased to 10 and 50 mg L−1, the decrease of cyanobacterial cell number was still not obvious. With 90 s ultrasonic irradiation and 5 mg L−1 TiO2/BC, the remaining cell number in water was 0.88 × 106 cells per mL. When the TiO2/BC concentration was 10 mg L−1, the concentration of cyanobacterial cells reduced to 0.42 × 106 cells per mL by 90 s of ultrasound treatment. With 50 mg L−1 of TiO2/BC and 90 s of ultrasonic irradiation, the cyanobacterial cell number further decreased to 0.8 × 105 cells per mL. These data show that the combination of ultrasound and TiO2/BC treatment can effectively enhance the coagulation to remove Microcystis aeruginosa cells.
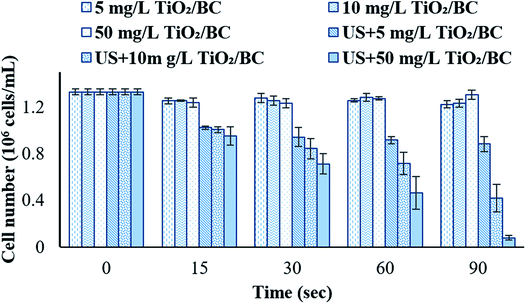 |
| Fig. 3 Coagulation removal of Microcystis aeruginosa cells using the assistance of ultrasound and TiO2/BC. | |
Although ultrasound alone did not significantly enhance the removal of the cyanobacterial cells, mild ultrasound is less harmful to humans, making it more suitable for disinfection on an industrial scale.20,21 In addition, there is less light scattering with ultrasound, making it more practical for water treatment. On the other hand, the removal of cyanobacteria was not promoted by the addition of sonocatalysts alone, which could be attributed to the low dosage (10–50 mg L−1) of the nanoparticles. Experiments were therefore performed to evaluating whether combining ultrasound and the TiO2/BC could promote the removal of cyanobacterial cells at the relatively low concentrations of TiO2/BC. In this work, the combination of ultrasound and sonocatalyst effectively promoted the efficiency of coagulation for algae removal. The combination of ultrasound and TiO2/BC has been proved to be an effective advanced oxidation reaction. It may work very similarly to other preoxidation methods such as Fenton reactions and Ozone treatment.3 It is suggested that ultrasound and the sonocatalyst TiO2/BC provided a moderate preoxidation to produce active species, which may change the extracellular polymer structure of Microcystis, leading to a considerable performance of coagulation for algae removal.22,23 Our results showed that even using low concentration of sonocatalyst, active species produced by ultrasound and TiO2/BC can effectively remove cyanobacterial cells via sedimentation.
3.3 The change in DOC and microcystins levels by ultrasound and TiO2/BC and coagulation process
In order to observe the effect of the combination of ultrasound and TiO2/BC pre-oxidation on water quality, we detected the change of dissolved organic carbon (DOC) in supernatant, which is shown in Fig. 4. In a short period of time, low-intensity ultrasound alone did not strongly release the intracellular organic matter of cyanobacteria, so the DOC level in water did not change significantly. For the combination of ultrasound and TiO2/BC, the pre-oxidation reduced DOC slightly, suggesting the moderate preoxidation did not strongly stimulate the cyanobacterial cells, so the cells did not release a large amount of organic matters. To further explore the pre-oxidation effects on water safety, the average concentrations of microcystins after coagulation are also shown in Fig. 4. The level of microcystin decreased with increasing of TiO2/BC dosage (from 5 to 50 mg L−1), suggesting that the TiO2/BC is helpful to control the release of microcystins. The mechanism of this control may involve the degradation of algal toxins by oxidation reaction, and/or the adsorption of algal toxins by TiO2/BC. Generally, the preoxidation and coagulation could remove cyanobacterial cells effectively and did not obviously increase the DOC and microcystins levels in the water, which is preliminarily proved that the method is safe.
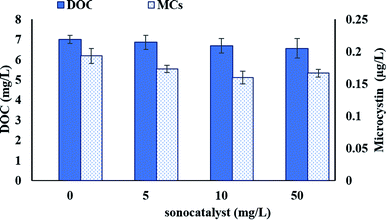 |
| Fig. 4 Changes in DOC and microcystins levels after ultrasound and TiO2/BC. The ultrasound irradiation time was 90 seconds. | |
The permeability of cyanobacterial cells is closely related to the DOC value and the level of algal toxins in water.7 Therefore, the permeability of cyanobacterial cells in water was detected using flow cytometry. Without irradiation, treatment with 50 mg L−1 of TiO2/BC left most cells intact in water. Fig. 5 shows that ultrasonic irradiation enhanced the cyanobacterial cell permeability, and the population of intact cells decreased significantly under the combination of ultrasound and 50 mg L−1 of TiO2/BC. The enhanced fluorescence signal of propidium iodide shows that, with ultrasound and TiO2/BC treatment, the permeability of cyanobacterial cells was changed, which could be explained that their cell membranes were damaged via oxidative reactions.5 Considering DOC and microcystins levels in water did not significantly increase, our results suggested that moderate pre-oxidation changes the cells' surface conditions, leading to a controllable increasing of cell permeability. Although we found that the permeability of cyanobacterial cells remained in water increased, the concentrations of DOC and microcystins in the supernatant did not increase significantly. This may be because most of the cyanobacterial cells are removed by pre-oxidation and coagulation, which did not have a negative impact on water quality.23,24 Thus, the pre-oxidation could effectively promote the coagulation and removal of cyanobacterial without secondary pollution. It could be a suitable method for handling sudden cyanobacterial blooms. Note, however, that the final microcystins levels in these experiments were above 0.1 μg L−1 for all of the treatments, so further treatment such as granular biochar adsorption should be applied for microcystins removal.
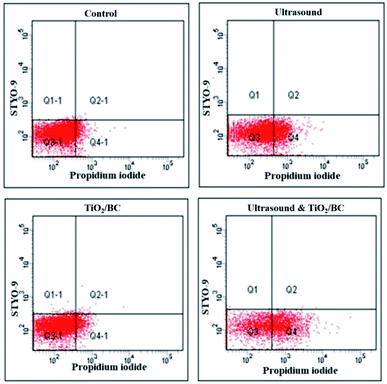 |
| Fig. 5 The change of the cell permeability of Microcystis aeruginosa cells using ultrasound, TiO2/BC, ultrasound and TiO2/BC. The ultrasound irradiation time was 90 seconds. | |
3.4 Mechanism investigation
Free radicals quenching experiments were performed to explore the active species involved in the reactions based on previous reports.25,26 Active species scavengers such as benzoquinone (BQ), and tert-butyl alcohol (TBA) were introduced to identify the roles of the main ROS. These chemicals could react with various radicals with different rates. Accordingly, BQ was considered as superoxide anion ˙O2− radicals' scavenger, and TBA was applied as the scavenger of ˙OH radicals. Because these scavengers themselves can cause the aggregation of cyanobacterial cells, it is impossible to get accurate results from the inhibition experiments of cyanobacterial removal. Alternatively, we selected rhodamine B (RhB) as the model organic chemical. All the scavengers obviously inhibited the removal efficiency, while TBA showed relatively higher inhibiting ability, suggesting ˙OH radicals are most responsible for the oxidation in the ultrasound/TiO2–BC process. The degradation of rhodamine B was also inhibited by BQ, indicating that h+ and ˙O2− may be also generated in the system (Fig. 6).
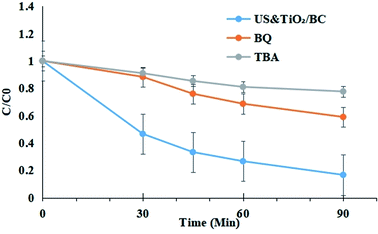 |
| Fig. 6 The degradation of RhB by ultrasound and TiO2/BC in the presence of various scavengers. | |
According to the experimental results and previous reports, we herein present the mechanism of TiO2–BC combined with ultrasound irradiation for antibacterial removal in water as below:
(i) In the presence of sonication, TiO2 could generate active species.26,27 Active species such as H˙ and ˙OH were generated by water molecules splitting that occurs via hot spots at the interface of the bubble–liquid as (eqn (1)). And the electron–hole pairs could be generated by TiO2 as semiconductor, the electrons can interact with oxygen molecules and generate ˙O2− via eqn (2):
(ii) The free radicals produced from the sonocatalytic reactions can react with the membrane of cyanobacterial cells, which is an essential factor for the electrostatic attraction between cyanobacterial cells and coagulants. Several researches have proved that the active radicals produced by sonocatalysis can moderately oxidize the cell membrane28 and/or improve the efficiency of coagulation of algae removal.29,30 Following reactions may occur (eqn (3) and (4)):
|
OH˙ + cell → cell** + AOM
| (3) |
|
˙O2− + cell → cell** + AOM
| (4) |
(iii) Moderate preoxidation only destroys the surface of cyanobacterial cells, without obvious cell rupture. This is supported by the evidence that after treatment, the concentration of organic matter and algal toxin in water did not increase significantly. Alternatively, there is another possibility that the active species generated from the oxidation system can degrade a certain concentration of algal organic matters, including cyanobacterial toxins. However, due to the low concentration sonocatalyst (50 mg L−1) and the ultrasonic radiation time is controlled within 90 seconds, the generation of free radicals is limited. Thus, it is suggested that ultrasound and TiO2/BC provides a moderate pre-oxidation system which can effectively remove cyanobacterial cells.
Ultrasonic pre-oxidation is of great significance to ensure the safety of drinking water. In the process of typical Fenton pre-oxidation, the low mass transfer efficiency of free radicals makes them unable to react with the target pollutants efficiently, resulting in self-quenching of free radicals or ineffective oxidation reactions (such as reaction with water molecules). At the same time, excessive oxidant may damage cyanobacterial cells, lead to the release of intracellular substances, and increase the risk of producing potentially carcinogenic disinfection by-products.31,32 Thus, it is still a big challenge to realize an efficient and safe moderate preoxidation, which encourages researchers to develop effective strategies to improve the mass transfer efficiency of free radicals at the cyanobacteria cell/catalyst interface. The usage efficiency of free radicals could then be achieved, leading to a moderate preoxidation of cyanobacteria cells.
In this work, ultrasound and TiO2/BC treatment effectively enhances the coagulation and removal of Microcystis aeruginosa cells. The permeability of some cyanobacterial cells was changed, suggesting that their cell membranes were damaged via oxidative reactions.5 The cyanobacterial cells are surrounded by negative-charged extracellular organic matter (EOM), hindering the electrostatic attraction between cyanobacteria cells and coagulants.28 Moderate treatment can release EOM to enhance the coagulation efficiency for cyanobacterial removal via adhesion bridging.28 Although the mechanism of ultrasound and TiO2/BC pre-treatment on cyanobacterial removal should be further explored, this work has confirmed that using mild ultrasound and TiO2/BC pre-oxidation promotes better coagulation. Meanwhile, the organic matters and microcystins levels in the water did not significantly increase. Moderate pre-oxidation changes the cells' surface conditions without causing large-scale cell lysis, probably due to the weak oxidative ability of ultrasound and the low TiO2/BC doses used. In this regard, ultrasound/TiO2/BC could reduce the required amount of chemicals, which should be a benefit of this integrated treatment.
4. Conclusions
TiO2/BC combined with ultrasound irradiation showed a moderate oxidising effect on these cyanobacteria. The cell number was reduced significantly after pre-oxidation and coagulation, and the concentrations of DOC and microcystins did not significantly increase. The free radicals quenching results proved that the TiO2/BC nanocomposites could be active by ultrasound, and active species such as ˙OH and ˙O2− were generated. Therefore, these findings obtained in this work may inform the fabricated TiO2/BC combined with ultrasound may provide a green and safe strategy for efficiently removing cyanobacteria for drinking water treatment.
Conflicts of interest
There are no conflicts to declare.
Acknowledgements
This work was supported by Natural Science Foundation of Jiangsu Province (BK20180938 and BK20160468).
References
- B. Liu, F. Qu, W. Chen, H. Liang, T. Y. Wang, X. X. Cheng, H. R. Yu and G. B. Li, Water Res., 2017, 125, 72–80 CrossRef CAS PubMed.
- M. Ma, R. P. Liu, H. J. Liu and J. H. Qu, J. Hazard. Mater., 2012, 217, 279–285 CrossRef PubMed.
- P. C. Xie, Y. Q. Chen, J. Ma, X. Zhang, J. Zou and Z. P. Wang, Chemosphere, 2016, 155, 550–563 CrossRef CAS PubMed.
- R. Henderson, S. A. Parsons and B. Jefferson, Water Res., 2008, 42, 1827–1845 CrossRef CAS PubMed.
- L. Gao, X. Pan, D. Zhang, S. Mu, D. J. Lee and U. Halik, Water Res., 2015, 69, 51–58 CrossRef CAS PubMed.
- X. C. Huo, D. W. Chang, J. H. Tseng, M. D. Burch and T. F. Lin, Environ. Sci. Technol., 2015, 49, 5502–5510 CrossRef CAS PubMed.
- J. Fan, L. Ho, P. Hobson and J. Brookes, Water Res., 2013, 47, 5153–5164 CrossRef CAS PubMed.
- C. W. Chang, X. C. Huo and T. F. Lin, Water Res., 2018, 141, 217–226 CrossRef CAS PubMed.
- M. Sendra, I. Moreno-Garrido, M. P. Yeste, J. M. Gatica and J. Blasco, Environ. Pollut., 2017, 227, 39–48 CrossRef CAS PubMed.
- Y. Pang, S. Lim and R. Lee, Environ. Sci. Pollut. Res., 2020, 27, 34638–34652 CrossRef CAS PubMed.
- D. Chen, S. Zhang, L. Zhuang, W. Rong and C. Yu, ACS Nano, 2017, 11, 9467–9480 CrossRef PubMed.
- Y. Wang, Y. Sun, S. Liu, L. Zhi and X. Wang, Ultrason. Sonochem., 2020, 63, 104968 CrossRef CAS PubMed.
- G. Li, L. Yi, J. Wang and Y. Song, Ultrason. Sonochem., 2020, 60, 104806 CrossRef CAS PubMed.
- A. Mehrizad, M. A. Behnajady, P. Gharbani and S. Sabbagh, J. Cleaner Prod., 2019, 215, 1341–1350 CrossRef CAS.
- L. Parizot, T. Chave, M. Galvez, H. Dutilleul and P. Da Costa, Appl. Catal., B, 2018, 241, 570–577 CrossRef.
- G. Lee, K. Chu, Y. Al-Hamadani, C. Park, M. Jang, J. Heo, N. Her, D. Kim and Y. Yoon, Chmosphere, 2018, 212, 723–733 CrossRef CAS PubMed.
- J. Colmenares, P. Lisowski and L. Dariusz, RSC Adv., 2013, 3, 20186–20192 RSC.
- J. L. Lin, L. C. Hua, Y. T. Wu and C. P. Huang, Water Res., 2016, 89, 261–269 CrossRef CAS PubMed.
- X. Wu, J. Liu and J. Zhu, Ultrason. Sonochem., 2018, 53, 68–76 CrossRef PubMed.
- S. Tian, S. Huang, Y. Zhu, G. Zhang, J. Lian, Z. Liu, L. Zhang and X. Qin, Ultrason. Sonochem., 2021, 73, 105527 CrossRef CAS PubMed.
- S. Babu, M. Ashokkumar and B. Neppolian, Top. Curr. Chem., 2016, 374, 1–32 CrossRef CAS PubMed.
- P. Jia, Y. Zhou, X. Zhang, Y. Zhang and R. Dai, Water Res., 2017, 131, 122–130 CrossRef PubMed.
- H. S. Ou, N. Y. Gao, Y. Deng, H. Wang and H. X. Zhang, Chmosphere, 2011, 85, 1192–1198 CrossRef CAS PubMed.
- J. A. Park, B. R. Yang, J. H. Kim, J. W. Choi, H. D. Park and S. H. Lee, Chem. Eng. J., 2018, 348, 125–134 CrossRef CAS.
- J. Qi, H. Lan, R. Liu, H. Liu and J. Qu, Water Res., 2020, 171, 115448 CrossRef CAS PubMed.
- Y. Zhao, Y. Chi, C. Tian, Y. Liu and A. Wang, Water Res., 2020, 177, 115789 CrossRef CAS PubMed.
- L. Lu, R. Shan, Y. Shi, S. Wang and H. Yang, Chemosphere, 2019, 222, 391–398 CrossRef CAS PubMed.
- Y. Wang, D. Zhao, W. Ma, C. Chen and J. Zhao, Environ. Sci. Technol., 2008, 42, 6173–6178 CrossRef CAS PubMed.
- X. G. Wu, G. F. Xu and J. J. Zhu, Ultrason. Sonochem., 2019, 58, 104634 CrossRef CAS PubMed.
- X. G. Wu, G. F. Xu and J. Wang, RSC Adv., 2020, 10, 13525–13531 RSC.
- Y. Li, X. Shi, Z. Zhang and Y. Peng, Ultrason. Sonochem., 2019, 55, 232–242 CrossRef CAS PubMed.
- Y. R. Huang, L. Li, X. M. Wei, H. Z. Li and R. Kuang, Ultrason. Sonochem., 2020, 69, 105278 CrossRef CAS PubMed.
|
This journal is © The Royal Society of Chemistry 2021 |
Click here to see how this site uses Cookies. View our privacy policy here.