DOI:
10.1039/D1RA03282H
(Paper)
RSC Adv., 2021,
11, 23113-23121
Recovery, reusability and stability studies of beta cyclodextrin used for cholesterol removal from shrimp lipid
Received
27th April 2021
, Accepted 22nd June 2021
First published on 30th June 2021
1 Introduction
Cyclodextrins (CDs) belong to the class of cyclic oligosaccharides composed of seven glucopyranose units. CDs mainly consist of α-cyclodextrin (α-CD), β-cyclodextrin (β-CD), and γ-cyclodextrin (γ-CD).1,2 The salient features of CDs are the structural complex with an inner hydrophobic and outer hydrophilic nature.1,3 Among the three CDs, β-CD is mostly used due to its lowest price and wide range of applications.4 β-CD is produced from starch using Bacillus macerans via amylase degradation.5 A β-CD inclusion complex is formed with hydrophobic compounds and further used in food applications such as encapsulation, oxidative protection and removal of undesirable taste.4 Although other CDs also consist of inner hydrophobic and outer hydrophilic parts, β-CD has an inner hydrophobic cavity diameter which is more suitable for the inclusion of aromatics, especially cholesterol.4 Since the presence of cholesterol in food is considered to be detrimental for health, it has been removed or reduced from the foods.6 The cholesterol entrapment capability of β-CD is considered to be vital in food applications for improvement of the nutrition of processed food product. β-CD was used for cholesterol removal from milk, pork lard, egg and ghee.7–9 The entrapment of target molecules was made by several techniques such as co-precipitation, slurry complexation, paste complexation, damp mixing and heating.4 During the complex formation by β-CD, several treatments such as homogenizing, heating and grinding have been employed to ensure the proper and effective entrapment of target molecule.4
Based on our previous study, β-CD: shrimp lipid ratio at 10
:
1 was used, followed by homogenization at high speed for 10 min, in which 97% of cholesterol from shrimp lipid was removed.10 This process required high amount of β-CD to remove cholesterol at a maximum level. Since β-CD has the tendency to complex with other components such as phospholipids, large amount of β-CD was employed for cholesterol removal. Although cholesterol was removed effectively from shrimp lipid, the huge amount of β-CD (10-fold of oil) was used.10 Therefore, high cost associated with β-CD would increase the operational cost for industries. This can further lead to the elevated market price of commercialized cholesterol removed shrimp lipid. Additionally, the used β-CD can cause environmental pollution, when its management is not appropriate. Hence, the reuse of the used β-CD would aid to alleviate such a drawback. Li et al. (2017) reported that β-CD after the cholesterol removal of duck egg powder can be activated for reuse purpose with the aid of ultrasonication.11 Cholesterol inclusion method used by Li et al. (2017) was slurry method using a blender.11 Generally, with mechanical homogenizer, the larger particles tend to breakdown into smaller size. This may disrupt the structure and physical properties of β-CD. The process used could therefore affect the efficacy of β-CD. However, the reuse or recycling of β-CD is necessary, in which β-CD can be fully exploited. Also operation cost for cholesterol removal could be reduced. No information of appropriate treatment of used β-CD to obtain the β-CD with quality equivalent to pure β-CD exists. This study was aimed to recycle the used β-CD after cholesterol removal process and to elucidate physical and structural properties of the obtained recycled β-CD.
2 Materials and methods
2.1 Chemicals
All the chemicals used in the experiment were analytical grade and purchased from Merck (Darmstadt, Germany). Pacific white shrimp (Litopenaeus vannamei) cephalothorax (shrimp head) were gifted from Sea Wealth Frozen Food Co., Ltd., Songkhla province, Thailand.
2.2 Cholesterol inclusion with β-CD in shrimp lipid
Shrimp lipid was firstly extracted by solvent extraction method.12 The extracted shrimp lipids were mixed with ethanol, in which polar and non-polar fractions were separated.13 Around 10 g of shrimp lipid was mixed with 50 mL of ethanol and allowed to crystallized at −20 °C for 2 h. The crystallized lipid was centrifuged at 3600 × g at 4 °C for 15 min (Hitachi Koki Co., Ltd, Tokyo, Japan). The above mentioned process was repeated twice for the complete separation of polar lipids from non-polar counterparts. The supernatant containing polar lipids such as phospholipids, cholesterol and pigments were collected. The solvent in polar lipid fraction was further evaporated using a rotary evaporator (EYELAN-1000, Tokyo Rikakikai, Co., Ltd., Tokyo, Japan).
The obtained polar lipid after ethanol removal (5 g) was mixed with 50 g of β-CD. The mixture was dispersed in 100 mL of ethyl acetate and 100 mL of water.10 The mixture was homogenized using a homogenizer (IKA Labortechnik, Selangor, Malaysia) for 10 min and centrifuged at 8000 × g for 20 min at 15 °C. The upper layer consisting of un-trapped compounds (ethyl acetate fraction with lowered cholesterol) was collected and subjected to solvent evaporation at 40 °C using a rotary evaporator. The aforementioned fraction was determined for cholesterol and fatty acid contents. β-CD bound with cholesterol situated in the pellet termed as ‘β-CD–CL’ was used for recycling study.
2.3 Recycling of used β-CD
β-CD recycling was performed using the modified method of Li et al. (2017).11 About 10 g of β-CD–CL was mixed with 150 mL of ethanol followed by the pH adjustment to 7.0 using 1.0 M KOH. The prepared suspension was poured in an airtight container and subjected to sonication using sonication bath (Elmasonic S 70H, Singen, Germany) for 40 min at 55 °C and then filtered using a Whatman filter paper no. 1 (Whatman plc, Madison, UK). The retentate was washed with 75 mL of ethanol to remove the cholesterol residues in β-CD–CL. Finally, the recycled β-CD (R-β-CD) was air-dried to remove the solvent and stored under vacuum (Fig. 1). To test efficiency for reuse of recycled β-CD, cholesterol removal of shrimp lipid was conducted using the inclusion complex formation as mentioned in section 2.2. Lipid treated with recycled β-CD was characterized in comparison with lipid treated with the pure β-CD (P-β-CD).
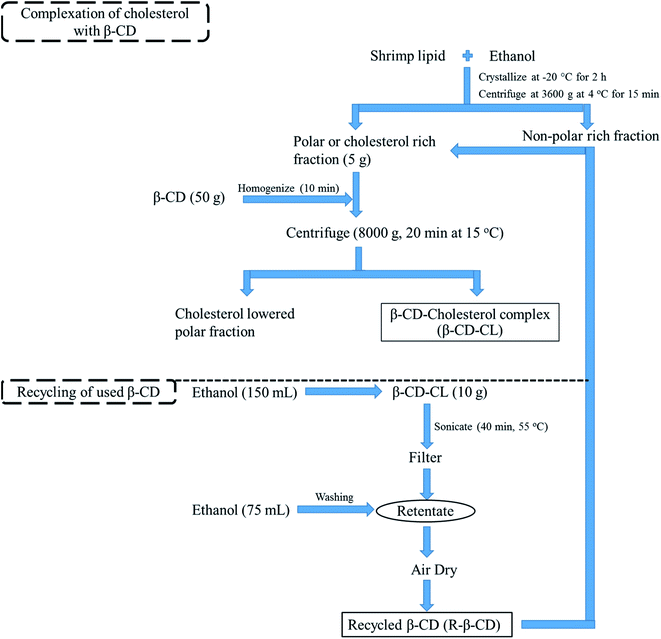 |
| Fig. 1 Flowchart of recycling of β-CD after cholesterol removal process. | |
2.4 Cholesterol and fatty acid contents in shrimp lipid treated with P-β-CD and R-β-CD
Shrimp lipids subjected to cholesterol removal using P-β-CD and R-β-CD was analyzed.
2.4.1 Determination of cholesterol content. The cholesterol contents in shrimp polar lipid before and after treatment with P-β-CD and R-β-CD were determined by the method of Raju and Benjakul, (2020).13 To determine cholesterol, high pressure liquid chromatography system (Waters 2695 series, Milford, MA, USA) equipped with photo diode-array detector was used. The peak separation was done using Diamonsil TM-C18 column (5 μm; 250 mm × 4.6 mm) with methanol–acetonitrile (50
:
50) mixture as mobile phase. The cholesterol peak was observed in 201 nm at 11 min. The peaks were identified and confirmed with cholesterol standards and the removal of cholesterol was expressed in percentage, relative to that found in initial shrimp lipid (untreated).
2.4.2 Residual cholesterol content in R-β-CD. Cholesterol removal from β-CD–CL complex was determined using spectrophotometric method as tailored by Luzia et al. (2003) by comparing the amount of retained cholesterol in β-CD–CL and R-β-CD.14 Around 100 mg of sample was added to 6 mL of saturated solution of ferrous sulphate prepared in glacial acetic acid. The mixture was further added with 2 mL of concentrated sulfuric acid (H2SO4). The absorbance of resulting solution was read at 490 nm using a spectrophotometer (Shimadzu UV-1800, Kyoto, Japan). Cholesterol standards from Sigma were used for the preparation of standard curve. Cholesterol residues were calculated for R-β-CD in comparison with that of β-CD–CL.
2.4.3 Fatty acid analysis. Fatty acid profile was analyzed by the method of Raju and Benjakul (2020).13 The lipid samples (0.1 g) were esterified with 200 μL of 2 M methanolic sodium hydroxide and neutralized with 200 μL of 2 M methanolic hydrochloric acid. Fatty acid methyl esters obtained by above mentioned process were analyzed by a gas chromatography (Agilent GC 7890B; Santa Clara, CA, USA). The GC conditions maintained for the analysis were 250 °C of injection temperature followed by the initial column temperature of 80 °C, which was programmed as follows: 4 °C min−1 ramp for 40 min to 220 °C and further increased to 240 °C. The separated compounds were detected at 270 °C detector (FID) temperature. Authentic standards (Supelco FAME mix, Bellefonte, PA, USA) were used for the identification of peak and expressed as g/100 g.
2.5 Characterization of R-β-CD in comparison with P-β-CD
2.5.1 Attenuated total reflection (ATR)-Fourier transform infrared (FTIR). FTIR spectra of both samples were analyzed by Bruker Model Equinox 55 FTIR spectrometer (Bruker Co., Esslingen, Germany) following the method of Singh et al., (2020).15 An empty cell was used for normalization at 25 °C with the wavenumber range of 4000–400 cm−1. OPUS 8.5 data collection software (Bruker Co. Billerica, MA, USA) was used.
2.5.2 Differential scanning calorimetry (DSC). Thermal properties of both samples were measured using a differential scanning calorimetric device (Perkin Elmer, Model DSC7, Norwalk, CA, USA) following the method of Mura et al. (2003).16 The samples were heated at the rate of 10 °C min−1 from 30 to 300 °C using an empty pan as the reference.
2.5.3 Hydrogen nuclear magnetic resonance (1H NMR). 1H NMR Cryoprobe prodigy mm BBO 500 MHz (Bruker Co., Switzerland) was used for the determination of proton shift of both samples. DMSO and D2O were used as solvents.17
2.6 Statistical analysis
Completely Randomized Design (CRD) was used for the whole study. Samples were analyzed in triplicates. Statistical analysis was performed using one-way analysis of variance (ANOVA) using SPSS 11.0 for windows (SPSS Inc., Chicago, IL, USA). t-test was used for pair comparison.
3 Results and discussion
3.1 Cholesterol removal from β-CD-cholesterol inclusion complex (β-CD–CL)
Firstly, β-CD–CL complex including cholesterol was subjected to ultrasonication in the presence of ethanol. Via this process, 97% of cholesterol was removed from β-CD cavity. The appropriate concentration of ethanol resulted in the diffusion of cholesterol and enhanced its mass transfer and solubilize cholesterol from the complex (β-CD–CL). When ultrasound was introduced, the migration of solvent into β-CD cavity via cavitational effect was enhanced and the interactions between ethanol and cholesterol were augmented.11 Li et al. (2017) used β-CD to remove cholesterol from duck egg and studied the β-CD recovery or recycling from β-CD-cholesterol complex.11 The optimum condition proposed by Li et al. (2017) was 10 mL g−1 of ethanol/β-CD–CL followed by sonication at 56 °C for 36 min.11 Increasing cholesterol removal was attained with increasing ethanol volume as well as ultrasonication power. Since shrimp lipids contained high amount of phospholipids and other hydrocarbons,18 the method developed in the present study was modified to increase the efficiency of cholesterol removal from the complex by extending the time to 40 min and the ethanol volume to 15 mL g−1 of β-CD–CL. Increasing volume of solvent could favor the augmented release of guest molecules bound to β-CD.11 Yamamoto et al. (2005) used foam separation method to dissociate cholesterol (44%) from β-CD–CL complex under elevated temperature and time.19 The cholesterol removal was increased with increasing temperature (from 298 K to 353 K) and time (from 0 to 180 min). The synergistic effect of bubbling and temperature aided the cholesterol to dissociate from its complex with β-CD.19 Tahir et al. (2013) used chemically modified β-CD to conjugate on the surface of glass beads for the cholesterol removal in milk. The modified β-CD aided to remove 73.6% of cholesterol with reusability of β-CD for multiple times.20
Previously, the cholesterol removal was done in shrimp lipids with β-CD using two different methods. First method was done by direct addition of β-CD in shrimp lipid.12 However, loss of some fatty acids was noticed for this method.12 Another method of cholesterol removal was developed to conquer the drawback of the aforementioned method. For this method, ethanolic fraction of shrimp polar lipid was separated first and β-CD was added. This method provided the improved cholesterol removal with augmented astaxanthin and lowered loss in fatty acids.10 Nevertheless, an excessive amount of β-CD was used for the second method to favor the additional binding of phospholipids and other sterols present in shrimp polar lipid.10 For the second process, the β-CD–CL was considered as byproduct and remained underutilized. The exploitation of β-CD–CL could be achieved by recovering β-CD. As a consequence, the recycled β-CD could be used for cholesterol removal in shrimp lipid.
3.2 Reusability of R-β-CD
There was no major change in cholesterol removal ability of R-β-CD when compared with P-β-CD (Fig. 2). Approximately, 95% (Fig. 2b) and 94% (Fig. 2c) of cholesterol was removed from shrimp polar lipid using P-β-CD and R-β-CD, respectively. Alternation in R-β-CD structure might occur to some degree during the recycling process. Additionally, the presence of 3% retained cholesterol in β-CD might bring about slightly lower efficiency of R-β-CD, compared to P-β-CD. Based on efficiency in cholesterol removal of R-β-CD, it can be deduced that β-CD could be reused for the entrapment of aromatic molecules such as cholesterol. The stability of β-CD could be due to the presence of low number of functional groups and mono layer chain, thus providing high β-CD stability and capacity of recycling for cholesterol removal.20 The reusability of β-CD could be a promising means with cost effectiveness via reducing the usage of fresh β-CD in cholesterol removal process.
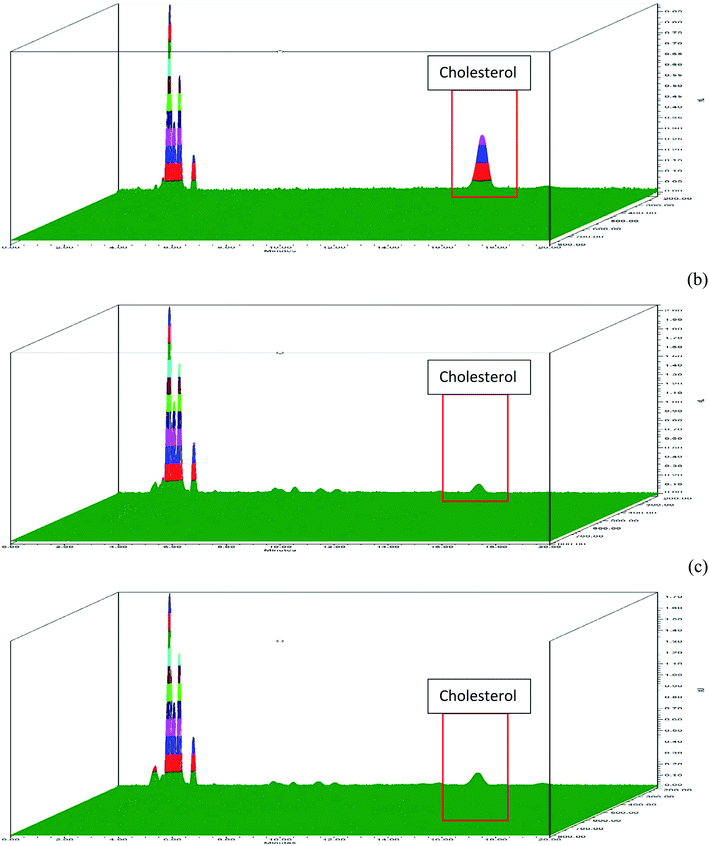 |
| Fig. 2 HPLC 3D PDA chromatograms of shrimp polar lipid (a), cholesterol removed shrimp polar lipid using P-β-CD (b) and cholesterol removed shrimp polar lipid using R-β-CD (c), (P-β-CD: pure β-CD and R-β-CD: recycled β-CD). | |
3.3 Thermal stability of P-β-CD and R-β-CD
Thermal stability of P-β-CD and R-β-CD was analyzed based on DSC thermogram (Fig. 3). P-β-CD showed melting peak at the onset temperature of 77.22 °C with the end at 123.57 °C. For R-β-CD, onset temperature was noted at 64 °C and ended at 112.84 °C. The phase transition of P-β-CD and R-β-CD was found at 107.1 °C and 99.83 °C, respectively. The phase transition of compounds provide the information about vaporization, sublimation and adsorption of the tested compounds.1
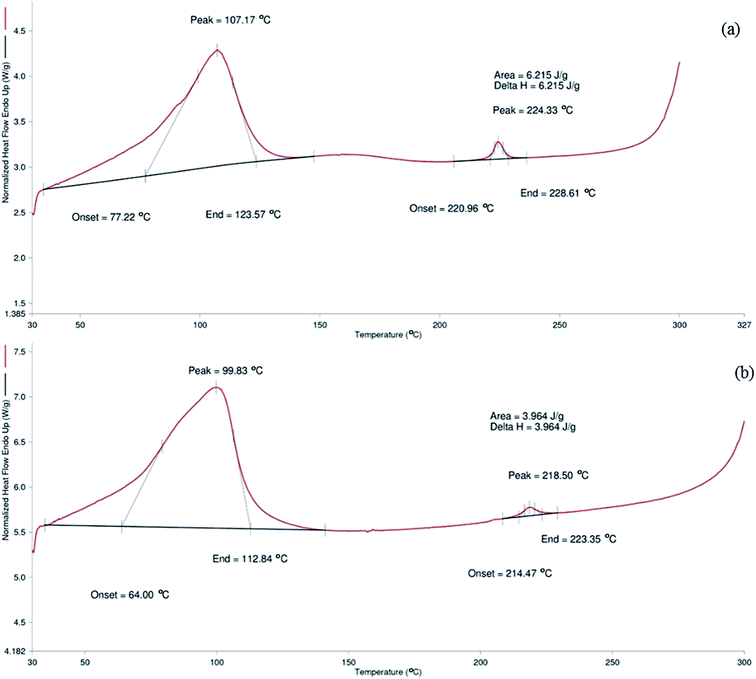 |
| Fig. 3 DSC thermogram of P-β-CD (a) and R-β-CD (b), (P-β-CD: pure β-CD and R-β-CD: recycled β-CD). | |
Thermal studies of β-CD with aceclofenac inclusion complex supports the present study that β-CD exhibited a melting peak at 109 °C.21 Although both the peaks were found in the same range, slight reduction was noted in the R-β-CD. The temperature difference indicated that β-CD might undergo slight modification after cholesterol removal and during recycling process. β-CD encountered harsh process such as high speed homogenization in the presence of ethyl acetate and water during inclusion of cholesterol. The peak shifting might be associated with the hydration of β-CD taken place during the cholesterol removal process from inclusion complex process22 It also resembled the initial stage of β-CD degradation. Bothara and Singh, (2012) reported that difference in thermal property occurred in natural polysaccharides was due to the degradation.23 However, Hădărugă et al. (2006) reported that β-CD showed the melting temperature with the range of 70–115 °C,24 which was less than that of P-β-CD in the current study but was similar to that of R-β-CD. These results suggested that the difference in melting point obtained in R-β-CD could be due to alternation in the hydrophilic and hydrophobic moieties during the cholesterol removal and β-CD recovery or recycling process to some extent. However, those changes in R-β-CD were minimal. Hence, R-β-CD was considered to be reusable for the cholesterol removal, although slight alterations in thermal stability of β-CD occurred.
3.4 FTIR spectra of P-β-CD and R-β-CD
FTIR spectra revealed the functional groups of P-β-CD and R-β-CD (Fig. 4). The spectra of both the samples were similar and no additional peaks were observed in R-β-CD. The absence of additional peaks in R-β-CD revealed that cholesterol and phospholipids in the inclusion complex were effectively removed during recovery or recycling process. The dominant peak in both samples were observed at 1025 cm−1, representing the OH bending vibration and OH symmetric, while asymmetric stretching was noted at 3300–3400 cm−1.25 The C–O–C vibration was noted at 1153 cm−1 and C–H vibration was also found at 1153 and 1029 cm−1.26 These functional groups were found to be similar between P-β-CD and R-β-CD. For asymmetric vibrations of C–H groups, which were found at 2880 cm−1, slight increase in wavenumber was found for R-β-CD. This peak represents the methyl group of cholesterol, suggesting the presence of trace amount of cholesterol in R-β-CD. This finding reconfirmed 97% cholesterol removal from β-CD–CL complex and the remaining cholesterol at 3%. Overall, FTIR spectra revealed that R-β-CD was mostly similar to P-β-CD. No changes in functional groups took place and the reusability of β-CD was confirmed.
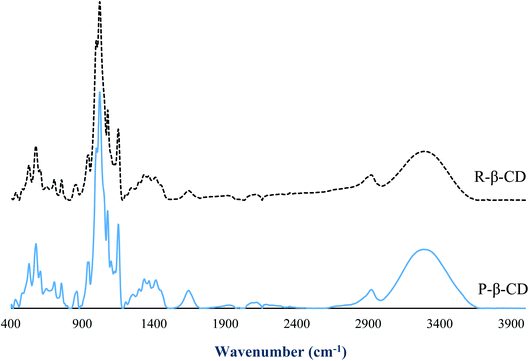 |
| Fig. 4 FTIR spectra comparison of P-β-CD and R-β-CD, (P-β-CD: pure β-CD and R-β-CD: recycled β-CD). | |
3.5 1H NMR of P-β-CD and R-β-CD
The structural modification of P-β-CD and R-β-CD was observed by NMR. Nowadays, NMR has become a vital tool for structural interpretation of several organic compounds. Fig. 5 represents the NMR spectra of P-β-CD and R-β-CD with different solvents including D2O and DMSO. The intramolecular exchange between water and β-CD occurs faster in NMR, leading to the restricted observations of OH groups.27 To detect OH group, DMSO was used as solvent and the OH 2, 3 and 6 were found in NMR spectra (Fig. 5). β-CD generally binds with the guest molecules with inner hydrophobic cavity. The protons of β-CD (shown inside Fig. 5) locating in the inner hydrophobic cavity are H3, H5 and H6,25 whereas the other two are situated at the outer ring of the β-CD. Based on spectra (Fig. 5) using D2O and DMSO as solvents, the identical spectra were observed between P-β-CD and R-β-CD spectra.
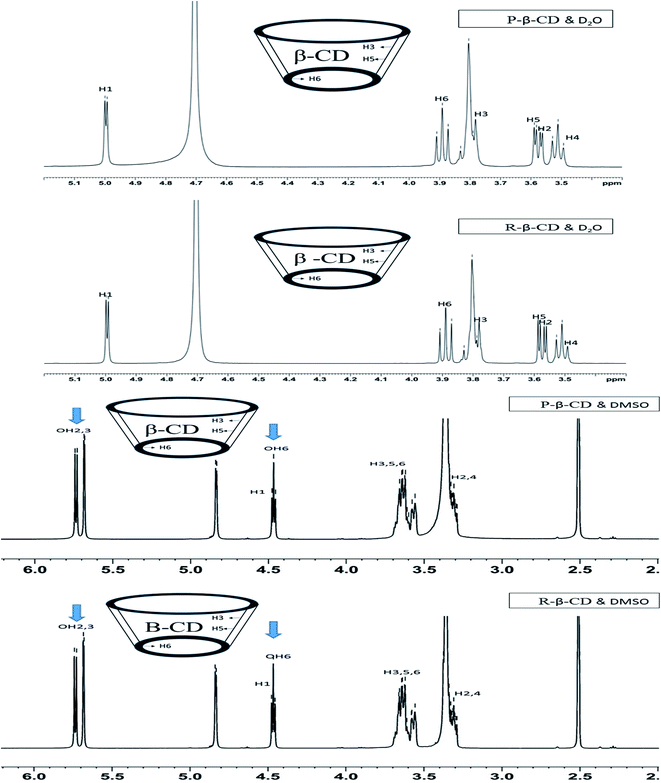 |
| Fig. 5 NMR spectra of P-β-CD and R-β-CD using D2O and DMSO as solvents, (arrow sign indicates OH), (P-β-CD: pure β-CD and R-β-CD: recycled β-CD). | |
Chemical shifts (Δδ) (Table 1) occurring in protons define the modifications of the recovered β-CD. Chemical shift variations were known to occur in protons present in free and bound states.28 The chemical shifts of DMSO soluble protons showed a minute difference in H2 and H4, whereas the inner cavity hydrophobic protons were unaltered (Table 1). However, water soluble protons showed a slight chemical shift in both inner H3, H5 and H6 (0.001, 0.002 and 0.002 ppm, respectively) and outer protons H1, H2 and H4 (0.002, 0.002 and 0.002, respectively) (Table 1). The chemical shift found in the water molecule could be due to the presence of some guest molecules that bound to β-CD in both inner and outer structure. Shan et al. (2019) prepared β-CD inclusion complex and analyzed for NMR spectra using DMSO as a solvent.26 The spectra of β-CD before and after inclusion suggested the chemical shift for H3 (0.011 ppm), H5 (0.006 ppm) and H6 (0.009 ppm), more likely due to the complex formation with β-CD. Inclusion complex between β-CD and phenylphosphonicdiamide showed the shift of H3, H5 and H6 protons around 0.002, 0.002 and 0.001 ppm, respectively.26 DMSO and water exhibited different chemical shifts to the protons. It confirmed that the recovered or recycled β-CD (R-β-CD) was mostly free of the guest molecule. Nevertheless, trace amount of molecules bound to β-CD in random manner was still detected. Overall, NMR spectra indicated that P-β-CD and R-β-CD were identical with minimum chemical changes. Thus it was deduced that R-β-CD had the potential for reusability.
Table 1 Chemical shift (Δδ) of P-β-CD and R-β-CD using different solvent (a) D2O and (b) DMSOa
Samples |
H1 (ppm) |
H2 (ppm) |
H3 (ppm) |
H4 (ppm) |
H5 (ppm) |
H6 (ppm) |
P-β-CD: pure β-CD and R-β-CD: recycled β-CD. |
(a) D2O |
P-β-CD |
4.998 |
3.567 |
3.78 |
3.491 |
3.587 |
3.889 |
R-β-CD |
5.000 |
3.569 |
3.781 |
3.493 |
3.589 |
3.891 |
Δδ |
0.002 |
0.002 |
0.001 |
0.002 |
0.002 |
0.002 |
![[thin space (1/6-em)]](https://www.rsc.org/images/entities/char_2009.gif) |
(b) DMSO |
P-β-CD |
4.831 |
3.295 |
3.62 |
3.328a |
3.639 |
3.657 |
R-β-CD |
4.831 |
3.296 |
3.62 |
3.329 |
3.639 |
3.657 |
Δδ |
0 |
0.001 |
0 |
0.001 |
0 |
0 |
3.6 Fatty acid profile of shrimp polar lipid treated with P-β-CD and R-β-CD for cholesterol removal
Fatty acid profile of shrimp polar lipid after the cholesterol removal with the aid of P-β-CD and R-β-CD are shown in Table 2. During cholesterol removal, some of the phospholipids could bind with outer portion of β-CD due to the hydrophilic nature. Raju and Benjakul (2019) reported that phospholipid was reduced after cholesterol removal process in shrimp lipid using β-CD.12 In addition, phospholipids were found to be conjugated with fatty acids, regardless of saturated, monounsaturated and polyunsaturated forms via their hydrophobic tails.18 Moreover, the cavity size of β-CD was appropriate for the heterocyclic structure rather than linear structure such as fatty acids.4 Fatty acid profile comparison revealed slightly higher fatty acid content in lipid treated with R-β-CD. This result showed that the binding between phospholipid and R-β-CD was lessened. Phospholipids have been reported to be rich in polyunsaturated fatty acids (PUFA).18 During cholesterol removal process, β-CD was used in the presence of water and ethyl acetate. Through this process, β-CD underwent interaction with water, in which it was hydrated to high degree. The hydration effect during the initial cholesterol removal and β-CD recovery or recycling process could lower the binding capacity of phospholipids, which had the hydrophobic tail. This led to the increased fatty acid profile of resulting treated shrimp polar lipid. Phospholipids could bind to the hydrophilic portion of β-CD due to its amphiphilic nature. Since ethyl acetate and water are immiscible in nature, the hydrated β-CD could not bind phospholipids effectively and favored the movement of phospholipids towards the ethyl acetate phase. The fatty acid profiling confirmed that the R-β-CD could be reused for the cholesterol removal. However, the multiple usage could reduce the efficiency in binding ability with phospholipids due to continuous hydration of β-CD. To overcome the hydration, β-CD should be dried under elevated temperature before further use.
Table 2 Fatty acid profile of cholesterol removed shrimp polar lipid treated with P-β-CD and R-β-CDa
Fatty acids (g/100 g) |
P-β-CD |
R-β-CD |
P-β-CD: pure β-CD and R-β-CD: recycled β-CD. |
C14:0 |
1.17 ± 0.01a |
1.25 ± 0.02a |
C15:0 |
0.58 ± 0.02a |
0.64 ± 0.01a |
C16:0 |
6.88 ± 0.10a |
6.99 ± 0.04a |
C16:1 |
0.86 ± 0.03a |
0.87 ± 0.01a |
C17:0 |
0.82 ± 0.03a |
0.86 ± 0.04a |
C18:0 |
3.22 ± 0.06a |
3.25 ± 0.03a |
C18:1n9c |
4.30 ± 0.06a |
4.34 ± 0.03a |
C18:2n3c |
3.56 ± 0.07a |
3.94 ± 0.02a |
C20:1 |
0.63 ± 0.02a |
0.65 ± 0.00a |
C18:3n3c |
0.60 ± 0.00a |
0.64 ± 0.00a |
C20:2n6c |
0.76 ± 0.02a |
0.85 ± 0.02a |
C23:0 |
1.83 ± 0.02a |
2.16 ± 0.03b |
C20:5n3c |
3.00 ± 0.06a |
3.92 ± 0.06b |
C22:6n3c |
2.93 ± 0.01a |
3.06 ± 0.04a |
4 Conclusion
Cholesterol was removed using β-CD from shrimp lipid. β-CD incorporated with cholesterol (β-CD–CL) was further processed using ethanol with the aid of ultrasound to obtain cholesterol free β-CD (R-β-CD). R-β-CD could remove 94% of cholesterol from shrimp polar lipid, which was comparable to P-β-CD (95% removal). DSC result showed a slight decrease in melting point of R-β-CD. FTIR and NMR results suggested that R-β-CD functional group and proton spectrum remained unaltered. Slight increase in fatty acid content of shrimp polar lipid was noticed when treated with R-β-CD. Overall results confirmed the reusability of β-CD after appropriate treatment. However, increased fatty acid profile with decreased cholesterol removal suggested that β-CD was slightly modified during recycling process. Although R-β-CD can be reused, continuous usage for cholesterol removal could have the lower efficiency.
Abbreviations
α-CD | α-Cyclodextrin |
γ-CD | γ-Cyclodextrin |
β-CD | β Cyclodextrin |
β-CD–CL | β Cyclodextrin cholesterol complex |
P-β-CD | Pure β cyclodextrin |
R-β-CD | Recycled β cyclodextrin |
ATR | Attenuated total reflection |
DSC | Differential scanning colorimeter |
DHA | Docosahexaenoic acid |
EPA | Eicosapentaenoic acid |
FTIR | Fourier transform infrared |
1H NMR | 1Hydrogen nuclear magnetic resonance |
PUFA | Polyunsaturated fatty acid |
PLF | Polar lipid fraction |
Author contributions
All authors contributed to this work. Navaneethan Raju, Avtar singh and Soottawat Benjakul conceptualized the idea, Navaneethan Raju conducted experiment, collect, interpret the data and wrote the manuscript draft. Avtar Singh provided the technical support. Soottawat Benjakul edited this article. The final version was approved by all authors.
Conflicts of interest
The authors declare no conflict of interest.
Acknowledgements
This work was supported by a Thailand's Education Hub for Southern Region of ASEAN Countries (TEH-AC, 2018) scholarship. Chair Professor grant (Grant No. P-20-52297) supported by National Science and Technology Development Agency (NSTDA), National Research Council of Thailand and Prince of Songkla University (Prachayacharn Grant No. AGR6402088N) were also acknowledged.
References
- G. Crini, S. Fourmentinn and E. Lichtfouse, Cyclodextrin Fundamentals, Reactivity and Analysis, Springer International Publishing, Cham, 2018, vol. 16 Search PubMed.
- A. Abou-Okeil, M. Rehan, S. M. El-Sawy, M. K. El-bisi, O. A. Ahmed-Farid and F. A. Abdel-Mohdy, Eur. Polym. J., 2018, 108, 304–310 CrossRef CAS.
- P. Jansook, N. Ogawa and T. Loftsson, Int. J. Pharm., 2018, 535, 272–284 CrossRef CAS PubMed.
- E. M. M. Del Valle, Process Biochem., 2004, 39, 1033–1046 CrossRef CAS.
- A. Munin and F. Edwards-Lévy, Pharmaceutics, 2011, 3, 793–829 CrossRef CAS PubMed.
- A. J. Lusis, Nature, 2000, 407, 233–241 CrossRef CAS PubMed.
- L. Alonso, P. Cuesta, J. Fontecha, M. Juarez and S. E. Gilliland, J. Dairy Sci., 2009, 92, 863–869 CrossRef CAS PubMed.
- S. H. Kim, H. Y. Kim and H. S. Kwak, Asian-Australas. J. Anim. Sci., 2007, 20, 1468–1472 CrossRef CAS.
- P. Bhatia, V. Sharma, S. Arora and P. S. Rao, Int. J. Food Prop., 2019, 22, 1–8 CrossRef CAS.
- N. Raju, T. Sae-leaw, K. Osako and S. Benjakul, J. Food Sci. Technol. Search PubMed , under revision..
- Y. Li, Y. Chen and H. Li, Ultrason. Sonochem., 2017, 34, 281–288 CrossRef CAS PubMed.
- N. Raju and S. Benjakul, Eur. J. Lipid Sci. Technol., 2019, 122, 1900242 CrossRef.
- N. Raju and S. Benjakul, Eur. J. Lipid Sci. Technol., 2020, 122, 2000078 CrossRef CAS.
- L. A. Luzia, G. R. Sampaio, C. M. . Castellucci and E. A. F. . Torres, Food Chem., 2003, 83, 93–97 CrossRef CAS.
- A. Singh, A. T. Idowu, S. Benjakul, H. Kishimura, R. E. Aluko and Y. Kumagai, Food Chem., 2020, 321, 126686 CrossRef CAS PubMed.
- P. Mura, F. Maestrelli, M. Cirri, S. Furlanetto and S. Pinzauti, J. Therm. Anal. Calorim., 2003, 73, 635–646 CrossRef CAS.
- A. M. L. Denadai, M. M. Santoro, L. H. D. Silva, A. T. Viana, R. A. S. Santos and R. D. Sinisterra, J. Inclusion Phenom., 2006, 55, 41–49 CrossRef CAS.
- S. Gulzar, N. Raju, R. Chandragiri Nagarajarao and S. Benjakul, Trends Food Sci. Technol., 2020, 100, 307–319 CrossRef CAS.
- S. Yamamoto, H. Kurihara, T. Mutoh, X. Xing and H. Unno, Biochem. Eng. J., 2005, 22, 197–205 CrossRef CAS.
- M. N. Tahir, C. Kwon, D. Jeong, E. Cho, S. R. Paik and S. Jung, J. Dairy Sci., 2013, 96, 4191–4196 CrossRef CAS PubMed.
- J. Shaikh, S. V. Deshmane, R. N. Purohit and K. R. Biyani, Indian Drugs, 2015, 52, 19–23 Search PubMed.
- R. O. Williams, V. Mahaguna and M. Sriwongjanya, Eur. J. Pharm. Biopharm., 1998, 46, 355–360 CrossRef CAS PubMed.
- S. B. Bothara and S. Singh, Asian Pac. J. Trop. Biomed., 2012, 2, S1031–S1035 CrossRef.
- N. G. Hădărugă, D. I. Hădărugă, V. Păunescu, C. Tatu, V. L. Ordodi, G. Bandur and A. X. Lupea, Food
Chem., 2006, 99, 500–508 CrossRef.
- K. P. Sambasevam, S. Mohamad, N. M. Sarih and N. A. Ismail, Int. J. Mol. Sci., 2013, 14, 3671–3682 CrossRef CAS PubMed.
- X. Shan, K. Jiang, J. Li, Y. Song, J. Han and Y. Hu, Polymers, 2019, 11, 71 CrossRef PubMed.
- H. J. Schneider, F. Hacket, V. Rüdiger and H. Ikeda, Chem. Rev., 1998, 98, 1755–1785 CrossRef CAS PubMed.
- T. Do Thi, K. Nauwelaerts, M. Froeyen, L. Baudemprez, M. Van Speybroeck, P. Augustijns, P. Annaert, J. Martens, J. Van Humbeeck and G. Van Den Mooter, J. Pharm. Sci., 2010, 99, 3863–3873 CrossRef PubMed.
|
This journal is © The Royal Society of Chemistry 2021 |
Click here to see how this site uses Cookies. View our privacy policy here.