DOI:
10.1039/D1RA03142B
(Paper)
RSC Adv., 2021,
11, 20788-20793
Direct synthesis of amides and imines by dehydrogenative homo or cross-coupling of amines and alcohols catalyzed by Cu-MOF†
Received
22nd April 2021
, Accepted 5th June 2021
First published on 10th June 2021
Abstract
Oxidative dehydrogenative homo-coupling of amines to imines and cross-coupling of amines with alcohols to amides was achieved with high to moderate yields at room temperature in THF using Cu-MOF as an efficient and recyclable heterogeneous catalyst under mild conditions. Different primary benzyl amines and alcohols could be utilized for the synthesis of a wide variety of amides and imines. The Cu-MOF catalyst could be recycled and reused four times without loss of catalytic activity.
Introduction
Imines are of great importance for the synthesis of chemical and biologically active compounds such as amines, chiral amines, amides, pyrrolines, hydroxyamines, and oxaziridines.1–3 Typically for the synthesis of imines, a mixture of an aldehyde or ketone with an amine in the presence of a catalyst is required. On the other hand, many useful oxidation methods for the synthesis of imines have been developed4 which include dimerization of primary amines5–10 oxidation of secondary amines11–14 and other methodologies.15–23
The formation of amide bonds is one of the most commonly used organic reactions due to the widespread presence of this functional group in natural products, pharmaceutical compounds, and synthetic polymers.24 Usually, amides are synthesized by the reaction of an amine and carboxylic acid, which needs a coupling reagent25 or conversion into reactive derivatives.26 These methods have several drawbacks, such as the use of hazardous and expensive reagents, generating stoichiometric amounts of waste which lead to environmental problems. To solve these problems, there is great interest in the development of atom economic and environmentally friendly routes for the synthesis of amides such as named reactions like the Beckmann rearrangement,27 the Schmidt reaction28 and Ugi29 reaction. A more recent method has considered the use of direct oxidative amidation from alcohols and aldehydes.30–41 However, these methods require the use of expensive transition metals as catalyst and in some cases the reactions require hazardous, expensive, dual catalyst and in most cases is a problem and the catalyst is not recyclable.42 Therefore, the search of a green, low cost and heterogeneous catalyst system remains a challenge. Recently, MOFs have attracted much more attention due to their structural and chemical diversities and have become very popular in the diverse research areas such as catalysis,43 drug delivery44 gas adsorption and storage45 and etc. As part of our ongoing work to develop catalytic activity of metal–organic frameworks (MOFs) as efficient heterogeneous, green and recyclable catalyst in organic synthesis46 we report herein an improved oxidative homo-coupling of amines to imines and oxidative cross-coupling of amine with alcohols to amides, using inexpensive and readily available Cu2(BDC)2(DABCO) as the recyclable catalysts and TBHP as oxidant (Scheme 1).
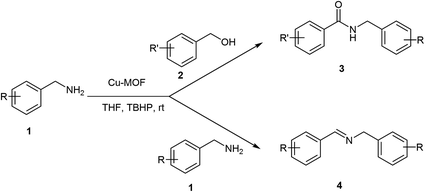 |
| Scheme 1 Oxidative homo and cross-coupling of amine with alcohols catalyzed by Cu-MOF. | |
Experimental section
Materials and methods
All the chemicals were purchased from commercial sources and used without further purification. Cu2(BDC)2(DABCO) was synthesized according to the our previously reported procedure.46a All reactions were monitored by thin layer chromatography (TLC) using plates coated with Merck 60 HF254 silica under UV light. Melting points were measured on an Electrothermal 9100 apparatus. 1H-NMR spectra were recorded with BRUKER DRX 400 and 500-AVANCE FT-NMR instrument (CDCl3 solution) at 400 MHz and 500 MHz, respectively. Scanning electron microscope (SEM) images were captured with a ZEISS scanning electron microscope at 30 kV with gold coating. X-ray powder diffraction (XRD) measurements were performed using an X'pert MPD. Philips diffractometer with Cu radiation source (λ = 1.54050 Å) at 40 kV voltage and 40 mA current.
Synthesis of amides via dehydrogenative cross-coupling of amines and alcohols catalyzed by Cu2(BDC)2(DABCO)
To a solution of amines (1 mmol) and alcohols (1 mmol) in THF (5 ml) was added Cu2(BDC)2DABCO (10% mol) and TBHP 70% (2 mmol) and the reaction mixture was stirred at room temperature for 24 h. The reaction progress was monitored by TLC. After the reaction was completed, the catalyst was filtered and the filtrate was evaporated under reduced pressure, and the residue was purified using silicagel column chromatography (hexane/ethyl acetate (3
:
1)).
Selected spectral data
N-Benzylbenzamide (3a), white solid; yield: 78%; 1H NMR (500 MHz, CDCl3): δ 7.81 (d, J = 7.1 Hz, 1H, CH of Ar), 7.43–7.45 (m, 3H, CH of Ar), 7.36–7.37 (m, 4H, CH of Ar), 7.29 (d, J = 7.1 Hz, 2H), 6.45 (s, 1H, NH), 4.85 (s, 2H, benzylic CH2).
Synthesis of imines via dehydrogenative homo-coupling of amines catalyzed by Cu2(BDC)2(DABCO)
To a solution of amines (2 mmol) in THF (5 ml) was added Cu2(BDC)2DABCO (10% mol) and TBHP 70% (4 mmol) and the reaction temperature was stirred at room temperature for 24 h. The reaction progress was monitored by TLC. After reaction completion, catalyst filtered and filtrate was evaporated under reduced pressure, and the residue was purified using silicagel column chromatography (hexane/ethyl acetate (3
:
1)).
N-(Benzylidene)benzylamine (4a).47 1H NMR (400 MHz, CDCl3): δ 8.44 (s, 1H, CH), 7.97–7.76 (m, 2H, CH of Ar), 7.57–7.42 (m, 3H, CH of Ar), 7.40 (d, J = 4.4 Hz, 4H, CH of Ar), 7.34–7.27 (m, 1H, CH of Ar), 4.88 (s, 2H, benzylic CH2).
Result and discussion
Cu2(BDC)2DABCO (Cu-MOF) catalysts were prepared and characterized according to our previous work.46a The synthetic Cu2(BDC)2DABCO was employed as a catalyst in the dehydrogenative homo-coupling of amines to imines and cross-coupling of amines with alcohols to amides. At the first, for getting to the optimum reaction conditions, various parameters such as solvent, amount of the catalyst and oxidant were examined for the model reactions (Table 1). Three solvent including THF, DMF and CH3CN were investigated for this homo-coupling reaction in presence of Cu-MOF as catalyst and TBHP as oxidant. The results are summarized in the Table 1 and can be seen that the maximum yield was obtained in the THF as solvent (Table 1, entries 4–6). For the study of the amount of catalyst in this reaction, 5, 10 and 20 mol% of the catalyst was used and as shown in Table 1, 10 mol% of Cu-MOF was the best for the synthesis of imines and amides (Table 1, entries 5, 7) and the higher amounts of the catalyst did not significantly effect on the reaction yield. TBHP was used as oxidant because of our previous experience in oxidative coupling reactions.43 Control experiments revealed that in the absence of Cu-MOF as catalyst (Table 1, entry 10) and TBHP as oxidant (Table 1, entry 11) the product 3a and 4a were not formed.
Table 1 Optimization of reaction conditions for synthesis of imine and amide from amines and alcoholsa
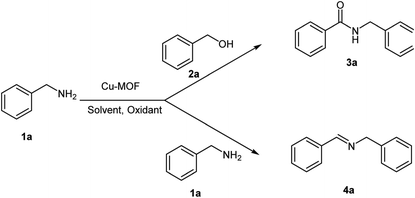
|
Entry |
Catalyst Cu-MOF (mol%) |
Oxidant |
Solvent |
Yield 3a (%) |
Yield 4a (%) |
Reaction condition: benzylamine 1a (1.0 mmol), benzyl amine 1a or benzyl alcohol 2a (1.0 mmol), TBHP (2.0 mmol), solvent (5 ml), time: 24 h, rt. |
1 |
5 |
TBHP |
CH3CN |
52 |
35 |
2 |
10 |
TBHP |
CH3CN |
60 |
40 |
3 |
20 |
TBHP |
CH3CN |
65 |
50 |
4 |
5 |
TBHP |
THF |
75 |
60 |
5 |
10 |
TBHP |
THF |
86 |
79 |
6 |
20 |
TBHP |
THF |
81 |
74 |
7 |
5 |
TBHP |
DMF |
10 |
25 |
8 |
10 |
TBHP |
DMF |
25 |
35 |
9 |
20 |
TBHP |
DMF |
25 |
35 |
10 |
— |
TBHP |
THF |
— |
— |
11 |
10 |
— |
THF |
— |
— |
After optimization of the model reaction in hand (Table 1, entry 5), to know the substrate scope a range of benzylic amine and benzylic alcohols for the synthesis of amides (Table 2) were used under the optimized reaction conditions. Benzylic alcohols and amines with different substituent provided the corresponding amides (3a–k) in excellent yields. Propyl amine was favored substrate, provided good yields of amide products.
Table 2 Cu-MOF catalyzed dehydrogenative cross-coupling of amines and alcohols to amidesa
For the investigation of substrate scope of oxidative dehydrogenative homo-coupling of benzyl amines to imines, benzyl amines with electron-donating group at ortho and para position were used and imines were formed in good yields (Table 3, entries 1–7) but benzyl amines with electron-withdrawing group at para position led to formation of a trace of product (Table 3, entry 8).
Table 3 Cu-MOF catalyzed dehydrogenative homo-coupling of amines to iminesa
A possible mechanism for these type of reactions is shown in Scheme 2. Cu(II) oxidized TBHP in such a way that TBHP transformed into tert-butylproxy radical. tert-Butylproxy radical captured a hydrogen from benzylamine or benzyl alcohol, thereby converting the benzylamine and benzyl alcohol into 5a and 7a proxy intermediates. Elimination of TBHP from this two intermediates led to imine 6a and benzaldehyde. Addition of benzyl amine to imine 6a and elimination of ammonia resulted imine 3a. Also, aminal 8a was obtained by addition of benzyl amine to benzaldehyde and continued oxidative dehydrogenation of aminal 8a by Cu(II) and TBHP led to amide 4a. The latter mechanism was confirmed by reaction between benzaldehyde and benzylamine under the same conditions and led to exclusive formation of the corresponding amide. This indicates that the reaction proceeds through an aldehyde. On the other hand, as shown in Table 1 products 3 and 4 were not formed in the absence of Cu-MOF and TBHP as catalyst and oxidant and all of these evidence supports our proposed mechanism.
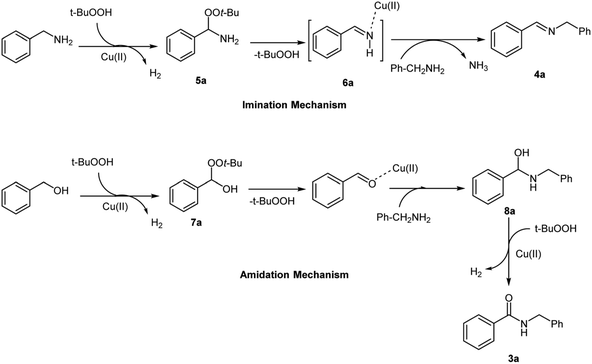 |
| Scheme 2 Proposed mechanism for the synthesis of amides and imines catalyzed by Cu-MOF. | |
The Cu-MOF catalyst also shown good recyclability and stability. The catalyst was recovered by simple filtration and washed with methanol and dried in oven and reused for 4 times (Table 4). The catalyst could be stored for a long time under air atmosphere without significant loss of catalytic activity. Also, the XRD pattern of Cu-MOF shows that the crystalline structure of Cu2(BDC)2DABCO is maintained after four run (Fig. S1†).46a
Table 4 Recovery and reuse of Cu-MOF in the dehydrogenative coupling of amines and alcohols
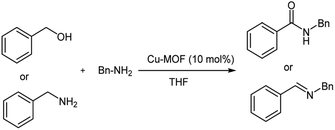
|
Run |
1 |
2 |
3 |
4 |
Yield (%) |
75 |
75 |
74 |
72 |
A comparison with other catalytic systems in the dehydrogenative homo- or cross-coupling of amines and alcohols demonstrated that our present Cu-MOF catalyst system exhibited a higher conversion and yield under milder conditions (Table 5).
Table 5 Comparison of activity for different catalytic systems in the coupling of benzyl alcohol and benzyl amine
Entry |
Catalyst |
Reaction condition |
Yield (compound 3a or 4a) |
Ref. no. |
1 |
Au6Pd/resin |
NaOH (1.1 eq.), O2 balloon, H2O, r.t., 2 h |
51% (3a) |
48 |
2 |
Ru(COD)Cl2/PCyp3.HBF4 |
KOtBu, toluene, 110 °C, 24 h |
78% (3a) |
49 |
3 |
Cu2O/CQDs |
CH3CN, O2, white cold LED λ > 400 nm, 24 h |
95% (4a) |
50 |
4 |
Co2(CO)8/trioctylphosphine oxide (TOPO) |
Mesitylene, 164 °C, 24 h |
79% (4a) |
51 |
5 |
Silicagel supported salicylic acid |
O2 (0.1 MPa), toluene, 90 °C, 24 h |
81% (4a) |
52 |
6 |
Manganese pincer complex |
KOtBu, toluene, 110 °C, 48 h |
88% (3a) |
53 |
7 |
Cu-MOF |
TBHP, THF, r.t |
86% (3a), 75% (4a) |
Present work |
Conclusion
In conclusion, we have identified Cu-MOF as a green and recyclable heterogeneous catalyst for the efficient dehydrogenative coupling of alcohols with amines for the synthesis of amides and imines. A different range of amines and alcohols are applicable in this two reactions. Furthermore, this cost-effective reaction provides practical alternatives for the synthesis of amides and imines under the mild conditions. Further studies on the catalytic application MOFs are in progress and would be presented in the future.
Conflicts of interest
There are no conflicts to declare.
References
- R. A. Volkmann, Nucleophilic addition to imines and imine derivatives, in Comprehensive Organic Synthesis, ed. B. M. Trost and I. Fleming, Pergamon Press, Oxford, 1st edn, 1991, pp. 355−396 Search PubMed.
- E. F. Kleinman and R. A. Volkmann, Reaction of allyl and propargyl/allenic organometallics with imines and iminium ions, in Comprehensive Organic Synthesis, ed. B. M. Trost and I. Fleming, Pergamon Press, Oxford, 1st edn, 1991, pp. 975−993 Search PubMed.
- V. N. G. Lindsay and A. B. Charette, Nucleophilic addition of nonstabilized carbanions to imines and imine derivatives, in Comprehensive Organic Synthesis, ed. P. Knochel and G. A. Molander, Academic Press, Oxford, 2nd edn, 2014, pp. 365−394 Search PubMed.
- G. M. Robertson, Imines and their N-substituted derivatives: NH, NR and N-haloimines, in Comprehensive Organic Functional Group Transformations, ed. A. R. Katritzky, O. Meth-Cohn and C. W. Rees, Pergamon Press,U.K., 1st edn, 1995, pp. 404−423 Search PubMed.
- S. Furukawa, Y. Ohno, T. Shishido, K. Teramura and T. Tanaka, ACS Catal., 2011, 1, 1150–1153 CrossRef CAS.
- L. Al-Hmoud and C. W. Jones, J. Catal., 2013, 301, 116–124 CrossRef CAS.
- A. E. Wendlandt and S. S. Stahl, Org. Lett., 2012, 14, 2850–2853 CrossRef CAS PubMed.
- M. Largeron and M. B. Fleury, Angew. Chem., Int. Ed., 2012, 51, 5409–5412 CrossRef CAS PubMed.
- L. Liu, S. Zhang, X. Fu and C.-H. Yan, Chem. Commun., 2011, 47, 10148–10150 RSC.
- C.-P. Dong, Y. Higashiura, K. Marui, S. Kumazawa, A. Nomoto, M. Ueshima and A. Ogawa, ACS Omega, 2016, 1, 799–807 CrossRef CAS PubMed.
- H. Yuan, W.-J. Yoo, H. Miyamura and S. Kobayashi, J. Am. Chem. Soc., 2012, 134, 13970–13973 CrossRef CAS PubMed.
- T. Sonobe, K. Oisaki and M. Kanai, Chem. Sci., 2012, 3, 3249–3255 RSC.
- L. Liu, Z. Wang, X. Fu and C.-H. Yan, Org. Lett., 2012, 14, 5692–5695 CrossRef CAS PubMed.
- B. Zhu, M. Lazar, B. G. Trewyn and R. J. Angelici, J. Catal., 2008, 260, 1–6 CrossRef CAS.
- J. Masdemont, J. A. Luque-Urrutia, M. Gimferrer, D. Milstein and A. Poater, ACS Catal., 2019, 9, 1662–1669 CrossRef CAS.
- M. Mastalir, M. Glatz, N. Gorgas, B. Stçger, E. Pittenauer, G. Allmaier, L. F. Veiros and K. Kirchner, Chem. –Eur. J., 2016, 22, 1–6 CrossRef PubMed.
- M. Rueping, C. Vila, A. Szadkowska, R. M. Koenigs and J. Fronert, ACS Catal., 2012, 2, 2810–2815 CrossRef CAS.
- J. Huang, L. Yu, L. He, Y.-M. Liu, Y. Cao and K.-N. Fan, Green Chem., 2011, 13, 2672–2677 RSC.
- Y. Xiang, Q. Meng, X. Li and J. Wang, Chem. Commun., 2010, 46, 5918–5920 RSC.
- G. Pelletier, W. S. Bechara and A. B. Charette, J. Am. Chem. Soc., 2010, 132, 12817–12819 CrossRef CAS PubMed.
- X. Cui, F. Shi and Y. Deng, Chem. Commun., 2012, 48, 7586–7588 RSC.
- S.-I. Naya, K. Kimura and H. Tada, ACS Catal., 2012, 3, 10–13 CrossRef.
- F. Bottaro, A. Takallou, A. Chehaiber and R. Madsen, Eur. J. Org. Chem., 2019, 2019, 7164–7168 CrossRef CAS.
- J. M. Humphrey and A. R. Chamberlin, Chem. Rev., 1997, 97, 2243 CrossRef CAS PubMed.
- C. A. G. N. Montalbetti and V. Falque, Tetrahedron, 2005, 61, 10827 CrossRef CAS.
-
(a) Y.-J. Kang, H.-A. Chung, J.-J. Kim and Y.-J. Yoon, Synthesis, 2002, 733 CrossRef CAS;
(b) I. Azumaya, T. Okamoto, F. Imabeppu and H. Takayanagi, Tetrahedron Lett., 2003, 59, 2325 CrossRef CAS;
(c) A. Teichert, K. Jantos, K. Harms and A. Studer, Org. Lett., 2004, 6, 3477 CrossRef CAS PubMed;
(d) D. M. Shendage, R. Froehlich and G. Haufe, Org. Lett., 2004, 6, 3675 CrossRef CAS PubMed;
(e) D. A. Black and B. A. Arndtsen, Org. Lett., 2006, 8, 1991 CrossRef CAS PubMed;
(f) A. R. Katritzky, C. Cai and S. K. Singh, J. Org. Chem., 2006, 71, 3375 CrossRef CAS PubMed;
(g) S. D. Roughley and A. M. Jordan, J. Med. Chem., 2011, 54, 3451 CrossRef CAS PubMed.
-
(a) E. Beckmann, Ber. Dtsch. Chem. Ges., 1886, 89, 988 CrossRef;
(b) N. A. Owston, A. J. Parker and J. M. J. Williams, Org. Lett., 2007, 9, 3599 CrossRef CAS PubMed.
-
(a) L. Yao and J. Aubé, J. Am. Chem. Soc., 2007, 129, 2766 CrossRef CAS PubMed;
(b) R. F. Schmidt, Ber. Dtsch. Chem. Ges., 1924, 57, 704 CrossRef.
-
(a) I. Ugi, Angew. Chem., Int. Ed., 1962, 74, 9 CrossRef CAS;
(b) I. Ugi, Angew. Chem., Int. Ed. Engl., 1962, 1, 8 CrossRef.
- A. Kumar, N. A. Espinosa-Jalapa, G. Leitus, Y. Diskin-Posner, L. Avram and D. Milstein, Angew. Chem., Int. Ed., 2017, 56, 14992–14996 CrossRef CAS PubMed.
- J. Gu, Z. Fang, Y. Yang, Z. Yang, L. Wan, X. Li, P. Wei and K. Guo, RSC Adv., 2016, 6, 89413 RSC.
- L. U. Nordstrøm, H. Vogt and R. Madsen, J. Am. Chem. Soc., 2008, 130, 17672–17673 CrossRef PubMed.
-
(a) K. Nakagawa, H. Inoue and K. Minami, Chem. Comm., 1966, 17, 17–18 RSC;
(b) K. Nakagawa, S. Mineo, S. Kawamura, M. Horikawa, T. Tokumoto and O. Mori, Synth. Commun., 1979, 9, 529 CrossRef CAS.
- J. Shie and J. Fang, J. Org. Chem., 2003, 68, 1158 CrossRef CAS PubMed.
-
(a) E. I. Marks and A. Mekhalfia, Tetrahedron Lett., 1990, 31, 7237 CrossRef;
(b) L. Wang, H. Fu, Y. Jiang and Y. Zhao, Chem. –Eur. J., 2008, 14, 10722 CrossRef CAS PubMed.
- N. W. Gilman, J. Chem. Soc. D, 1971, 733 RSC.
- S. D. Sarkar and A. Studer, Org. Lett., 2010, 12, 1992 CrossRef PubMed.
-
(a) K. Ekoue-Kovi and C. Wolf, Org. Lett., 2007, 9, 3429 CrossRef CAS PubMed;
(b) K. Ekoue-Kovi and C. Wolf, Chem. –Eur. J., 2008, 14, 6302 CrossRef CAS PubMed.
-
(a) H. U. Vora and T. Rovis, J. Am. Chem. Soc., 2007, 129, 13796 CrossRef CAS PubMed;
(b) J. W. Bode and S. S. Sohn, J. Am. Chem. Soc., 2007, 129, 13798 CrossRef CAS PubMed.
- W.-J. Yoo and C.-J. Li, J. Am. Chem. Soc., 2006, 128, 13064 CrossRef CAS PubMed.
- S. C. Ghosh, J. S. Y. Ngiam, C. L. L. Chai, A. M. Seayad, D. T. Tuan and A. Chen, Adv. Synth. Catal., 2012, 354, 1407 CrossRef CAS.
-
(a) S. C. Ghosh, J. S. Y. Ngiam, A. M. Seayad, D. T. Tuan, C. L. L. Chai and A. Chen, J. Org. Chem., 2012, 77, 8007–8015 CrossRef CAS PubMed;
(b) R. Cadoni, A. Porcheddu, G. Giacomelli and L. D. Luca, Org. Lett., 2012, 14, 5014–5017 CrossRef CAS PubMed;
(c) J. Gu, Z. Fang, Y. Yang, Z. Yang, L. Wan, X. Li, P. Wei and K. Guo, RSC Adv., 2016, 6, 89413–89416 RSC.
- V. Pascanu, G. G. Miera, A. K. Inge and B. Martín-Matute, J. Am. Chem. Soc., 2019, 141, 7223–7234 CrossRef CAS PubMed.
- Y. Sun, L. Zheng and Y. Yang, et al., Nano-Micro Lett., 2020, 12, 103 CrossRef CAS.
-
(a) H. Zhang, L. M. Yang, H. Pan and E. Ganz, Phys. Chem. Chem. Phys., 2020, 22, 24614–24623 RSC;
(b) H. Zhang, X. Zheng, L. M. Yang and E. Ganz, Inorg. Chem., 2021, 60(4), 2656–2662 CrossRef CAS PubMed;
(c) H. Zhang, L. M. Yang and E. Ganz, Langmuir, 2020, 36(46), 14104–14112 CrossRef CAS PubMed;
(d) H. Zhang, C. Shang, L. M. Yang and E. Ganz, Inorg. Chem., 2020, 59(22), 16665–16671 CrossRef CAS PubMed;
(e) H. Zhang, L. M. Yang, H. Pan and E. Ganz, Cryst. Growth Des., 2020, 20(10), 6337–6345 CrossRef CAS;
(f) H. Zhang, L. M. Yang and E. Ganz, ACS Appl. Mater. Interfaces, 2020, 12(16), 18533–18540 CrossRef CAS PubMed;
(g) H. Zhang, L. M. Yang and E. Ganz, ACS Sustainable Chem. Eng., 2020, 8(38), 14616–14626 CrossRef CAS;
(h) X. Zheng, H. Zhang, L. M. Yang and E. Ganz, Cryst. Growth Des., 2021, 21, 2474–2480 CrossRef CAS.
-
(a) L. Panahi, M. R. Naimi-Jamal, J. Mokhtari and A. Morsali, Microporous Mesoporous Mater., 2016, 244, 208–217 CrossRef;
(b) S. Akbari, J. Mokhtari and Z. Mirjafary, RSC Adv., 2017, 7, 40881–40886 RSC;
(c) A. Khosravi, J. Mokhtari, M. R. Naimi-Jamal, Sh. Tahmasebi and L. Panahi, RSC Adv., 2017, 7, 46022–46027 RSC;
(d) S. Tahmasebi, A. Khosravi, J. Mokhtari, M. R. Naimi-Jamal and L. Panahi, J. Organomet. Chem., 2017, 853, 35–41 CrossRef CAS;
(e) J. Mokhtari and B. A. Hassani, Inorg. Chim. Acta., 2018, 482, 726–731 CrossRef CAS;
(f) Z. Ahmadzadeh, J. Mokhtari and M. Rouhani, RSC Adv., 2018, 8, 24203–24208 RSC;
(g) S. Jamalifard, J. Mokhtari and Z. Mirjafary, RSC Adv., 2019, 9, 22749–22754 RSC.
- A. E. Wendlandt and S. S. Stahl, Chemoselective organocatalytic aerobic
oxidation of primary amines to secondary imines, Org. Lett., 2012, 14, 2850–2853 CrossRef CAS PubMed.
- L. Zhang, W. Wang, A. Wang, Y. Cui, X. Yang, Y. Huang, X. Liu, W. Liu, J. Y. Son, H. Ojic and T. Zhang, Green Chem., 2013, 15, 2680–2684 RSC.
- L. U. Nordstrøm, H. Vogt and R. Madsen, J. Am. Chem. Soc., 2008, 130, 17672–17673 CrossRef PubMed.
- A. Kumar, A. Hamdi, Y. Coffinier, A. Addad, P. Roussel, R. Boukherroub and S. Jain, J. Photochem. Photobiol. A, 2018, 356, 457–463 CrossRef CAS.
- F. Bottaro, A. Takallou, A. Chehaiber and R. Madsen, Eur. J. Org. Chem., 2019, 2019, 7164–7168 CrossRef CAS.
- C. P. Dong, Y. Higashiura, K. Marui, S. Kumazawa, A. Nomoto, M. Ueshima and A. Ogawa, ACS Omega, 2016, 1, 799–807 CrossRef CAS PubMed.
- A. Kumar, N. A. Espinosa-Jalapa, G. Leitus, Y. Diskin-Posner, L. Avram and D. Milstein, Angew. Chem., Int. Ed., 2017, 56, 14992–14996 CrossRef CAS PubMed.
Footnote |
† Electronic supplementary information (ESI) available. See DOI: 10.1039/d1ra03142b |
|
This journal is © The Royal Society of Chemistry 2021 |
Click here to see how this site uses Cookies. View our privacy policy here.