DOI:
10.1039/D1RA02494A
(Paper)
RSC Adv., 2021,
11, 21375-21383
One-step-immunoassay of procalcitonin enables rapid and accurate diagnosis of bacterial infection†
Received
30th March 2021
, Accepted 9th June 2021
First published on 17th June 2021
Abstract
Procalcitonin (PCT) (i.e. a precursor of calcitonin) attracts much attention as a reliable biomarker of bacterial infections because its concentration increases rapidly in the blood when bacterial infections occur in the body. Sepsis may occur due to indiscriminate and vigorous proliferation of infectious bacteria, and accordingly early diagnosis and treatment of bacterial infection are of crucial importance. However, current diagnostic methods for sepsis suffer from long assay time, multiple and complex assay steps, inaccuracy, and requirement of analytical equipments. The goal of this study is to develop an advanced one-step-immunoassay that enables quick and accurate diagnosis of sepsis through measuring the PCT concentration in patient sera, which is based on self-enhancement of optical detection signals from large gold particles (i.e. clusters of gold nanoparticles) that are formed on the agglomerates of PCT-bound 3-dimensional (3D) probes. The 3D probe is constructed through attaching polyclonal anti-PCT antibodies (IgGs) to the surface of a modified hepatitis B virus (HBV) capsid, where both tandem repeats of the B domain of Staphylococcal protein A (SPAB) and the hexa-histidine tag are inserted into each HBV core protein (i.e. subunit of HBV capsid). That is, anti-PCT IgGs are attached via strong interaction between the Fc region and surface-exposed SPAB. Furthermore, hook effect-free and PCT concentration-dependent optical signals were consistently generated by adding both bovine serum albumin (BSA) and nickel ions to patient sera and also by optimally adjusting the 3D probe concentration. Compared to conventional chemiluminescent microparticle immunoassay (CMIA) showing poor linearity of detection signals, this novel immunoassay accurately detected PCT with good linearity between PCT concentrations and optical signals in a wide range of PCT concentrations (0.05–200 ng mL−1) and also showed a sufficiently low limit of detection, resulting in 100% sensitivity and 100% specificity when tested with 30 sepsis patients and 30 healthy individuals.
Introduction
Bacterial infection can happen in any part of the body and often causes serious diseases such as pneumonia, meningitis, etc.1 If the infection is not appropriately treated at an early stage, sepsis may occur due to spreading of the bacteria to the bloodstream,2 which induces a dysregulated host response and causes life-threatening organ dysfunction.2,3 The patient mortality of severe sepsis is 25 to 30% and increases up to 40 to 70% for septic shock,3–5 indicating that rapid and accurate diagnosis and timely treatment of sepsis is essential to enhancing survival rate.6–8 However, ‘gold standard’ of sepsis diagnosis that enables both high speed and high accuracy is not currently available.9,10
Because septic symptoms including fever, tachycardia, tachypnea, and leukocytosis4,7 are not sepsis-specific, or overlap with those of other noninfectious systemic inflammation,4,11 either blood culture or detection of sepsis-specific biomarkers has been used as diagnostic assay method.9–12 Blood culture is a direct method for confirming bacterial infection13–16 but it takes 1 to 3 days until the assay is completed and therefore is not suitable for rapid diagnosis. Furthermore, this labor-intensive method suffers from low sensitivity and may miss slow-growing or fastidious bacteria.16,17 Polymerase chain reaction (PCR)-based assay can detect bacterial cells within several hours but is not sufficiently sensitive for the blood samples containing bacteria at low concentration such as approximately 1 to 100 colony forming units. Therefore, this PCR method often requires a culture-enrichment step, leading to delayed assay and increased cost.18–20
Recently, PCT has attracted much attention as a reliable biomarker for sepsis. PCT (116 amino acids, 13 kDa) is a precursor of calcitonin produced in C-cells of thyroid gland21,22 and closely associated with host responses to bacterial infection.22–25 Under normal conditions, mature calcitonin is secreted into blood after pro-sequence of PCT is removed in the cells,12 and accordingly serum concentration of PCT in healthy individuals are very low.22 However, in case of bacterial infection PCT production is induced by bacterial endotoxin and inflammatory mediators such as tumor necrosis factor and interleukin-6, followed by rapid increase of serum concentration of PCT.23 Reportedly, PCT concentration correlates with severity of sepsis.26–28 Although well-known inflammatory indicator C-reactive protein (CRP) has been also considered as a biomarker for bacterial infection, it is currently accepted that PCT is more reliable than CRP in the accurate diagnosis of sepsis,29 because compared to CRP, PCT concentration increases at earlier stage of bacterial infection and finally increases by several thousand fold more,29,30 providing a better negative predictive value.12,31,32 Reportedly,33 less than 0.1 ng mL−1 of PCT has been detected in healthy individuals, whereas PCT concentrations at early stage of infection is higher than 0.5 ng mL−1. Patients with severe sepsis have PCT concentrations over 2 ng mL−1, and patients under septic shock have PCT concentrations over 10 ng mL−1.33
As PCT assay methods, lateral flow assay (LFA) such as BRAHMS PCT-Q (ThermoFisher Scientific, Waltham, MA, USA) and immunoluminometric assay such as LUMItest have been reported.12 BRAHMS PCT-Q can rapidly detect serum PCT levels in 30 min but is only semi-quantitative and not sensitive enough to detect moderately elevated PCT concentrations.12,34 Luminometer-dependent LUMItest using two different monoclonal antibodies with one being luminescence-labeled is useful for diagnosis of severe sepsis, but it is not sensitive enough to detect mildly or moderately elevated PCT levels.12,35–38 These indicate that both BRAHMS PCT-Q and LUMItest are not adequate for early diagnosis of sepsis that requires high sensitivity and quantitative accuracy.
In this study, we accurately and rapidly measured PCT using an enhanced one-step-immunoassay that is performed through the following events that occur simultaneously and rapidly in assay solution: PCT-dependent clustering of gold ion-adsorbed 3D probes, gold ion reduction, and rapid formation of large gold particles, which enables self-enhancement of optical signals. To avoid undesirable hook effect, the amount of anti-PCT 3D probes was optimally adjusted, and for quantitatively consistent and accurate assay, bovine serum albumin (BSA) and nickel ions (Ni2+) were added to patient sera prior to the immunoassay. We successfully detected PCT over a broad range of concentrations (0.05–200 ng mL−1), and assay sensitivity and specificity were all 100% in the diagnosis of 30 sepsis patients and 30 healthy individuals, indicating that this one-step-immunoassay is suitable for accurate diagnosis of early-stage sepsis as well as severe sepsis and septic shock.
Methods
Synthesis of anti-PCT 3D probes
To detect PCT in patient sera for diagnosing sepsis, anti-PCT 3D probes were prepared as described in Fig. 1. Through assembly PCR with appropriate primers, we prepared two clones derived from hepatitis B virus (HBV) core protein (HBVcAg) gene, which code for NH2-NdeI-hexahistidine(H6)-HBVcAg(1–78)-G4SG4T-XhoI-COOH and NH2-BamHI-G4SG4S-HBVcAg(81–149)-HindIII-COOH. To replace P79A80 of HBVcAg with tandem repeat of B domain (209–271) of Staphylococcal protein A (SPAB), we prepared two additional clones, NH2-XhoI-SPAB-EcoRI-COOH and NH2-EcoRI-SPAB-BamHI-COOH. Sequential ligation of these four clones was performed to construct the expression vector pT7-HBVC-HSPA, which encodes NH2–H6-HBVcAg(1–78)-G4SG4T-(SPAB)2-G4SG4-HBVcAg(81–149)-COOH (i.e., H6-SPAB-capsid). After complete DNA sequencing of the gel-purified expression plasmid vector, Escherichia coli strain BL21(DE3) [F−ompThsdSB(rB−mB−)] was transformed with pT7-HBVC-HSPA and ampicillin-resistant transformants were selected. The experimental procedures for gene expression, purification, and transmission electron microscope (TEM) image analysis of H6-SPAB-capsid are described in our previous report.39 Expression level and solubility of H6-SPAB-capsid were analyzed using a densitometer (GS-800, BIO-RAD Inc., Hercules, CA, USA) and image analysis software (Quantity One, BIO-RAD Inc., Hercules, CA, USA) (ESI Fig. S1†). Sheep anti-PCT polyclonal IgG antibody (cat. no. PA1-75361, Invitrogen, Carlsbad, CA, USA) was diluted to 5 (or 50) μg mL−1 in a final volume of 200 μL PBS consisting of 2.7 mM KCl, 137 mM NaCl, 2 mM KH2PO4, and 10 mM Na2HPO4 at pH 7.2 and was subsequently mixed with H6-SPAB-capsid at the concentration of 6.25 (or 62.5) μg mL−1 in 800 μL PBS buffer (pH 7.4). The mixture was then incubated with mild stirring at 4 °C for 12–16 h to prepare the pre-assay solution.
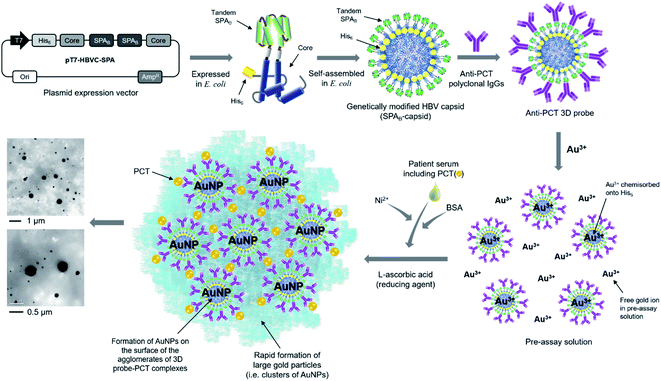 |
| Fig. 1 Schematic illustration of advanced one-step-immunoassay of PCT in patient serum. | |
One-step-immunoassay for detecting PCT
Preparation of standard PCT serum solution. We tested sera of actual sepsis patients and also prepared standard PCT serum solutions containing recombinant human PCT (rPCT) to evaluate quantitative performance of one-step-immunoassay: 2 μL of rPCT (cat. no. RP-75698; Invitrogen) at 0.1 mg mL−1 was added to 998 μL of healthy donor sera (Seoul National University Hospital Biomedical Research Institute, Seoul, Republic of Korea), followed by serial dilution with healthy sera, resulting in the standard serum solutions of rPCT at the concentrations of 0.01–200 ng mL−1. All procedures of using patient and healthy sera were performed in accordance with the Guidelines for Care and Use of human biological materials of Korea university and approved by the Institutional Review Board of Korea University. And the consent for use of all human sera was obtained from the Seoul National University Hospital Biomedical Research Institute. To prevent free gold ions (Au3+) from binding to serum proteins, nickel ion (Ni2+) solution containing 25% (w/v) NiCl2 (cat. No. 451193; Sigma Aldrich, St. Louis, MO, U.S.A.) in PBS was mixed with the sera at 1
:
49 ratio (v/v) prior to immunoassays.
Bovine serum albumin (BSA) preparation. To prevent free Au ions from binding to BSA, 0.18 g of NiCl2 was added to a BSA solution containing 15% (w/v) BSA (cat. no. BAC65; Equitech-Bio Inc., Kerrville, TX, U.S.A.) in 30 mL of PBS (pH 7.4), followed by mild stirring at 4 °C.
One-step-immunoassay of PCT. To prepare pre-assay solution, Au3+ ion solution containing 0.5% (w/v) HAuCl4 (cat. no. 254169; Sigma Aldrich) in 500 μL of PBS (pH 7.4) was mixed with a solution of anti-PCT 3D probes. The mixture was then incubated with mild stirring at 4 °C for 12–16 h. To perform the one-step-immunoassay for sepsis diagnosis, 24 μL of the pre-assay solution, 6 μL of BSA solution, 2 μL of patient or healthy donor serum, and 8 μL of L-ascorbic acid (LAA) (cat. no. A7506; Sigma Aldrich) [0.05 M in PBS (pH 7.4)] were added to each well of a 384-well plate (cat. 3680; Corning Inc., Corning, NY, U.S.A.). Absorbance at 564 nm (O.D.564 nm) was measured immediately and every 5 minutes using an Infinite M200 Pro microplate reader (TECAN, Männedorf, Switzerland) at room temperature.
Chemiluminescent microparticle immunoassay (CMIA) using standard PCT serum solutions
Standard PCT serum solutions containing rPCT at the concentrations of 0.01–200 ng mL−1 that were prepared as described above were analyzed using ARCHITECT B·R·A·H·M·S PCT CMIA (Abbott, Chicago, IL, U.S.A.) that was available at Green Cross Laboratory (Yong-In, Republic of Korea).
TEM, dynamic light scattering (DLS), and energy dispersive X-ray (EDX) analyses of assay solutions
For TEM analysis 7 μL of assay solution was dropped onto 200 square mesh copper grids with carbon film (Electron Microscopy Science, Hatfield, PA, U.S.A.). Two minutes later, the grids were washed twice with distilled water. Samples on the grids were stained with 2% (w/v) uranyl acetate solution and subsequently air-dried for 1 h. TEM and EDX spectroscopy analyses were conducted using a Tecnai 20 TEM (FEI, Hillsboro, OR, U.S.A) operated at 200 kV. TEM images were obtained using a charge-coupled device (CCD) camera and FEI-imaging software installed on the Tecnai 20 TEM. The hydrodynamic size of gold particles (i.e. AuNP clusters formed on agglomerates of 3D probe–PCT complexes) was determined through DLS analysis using a 90 Plus Zeta Potential/Particle Size Analyzer (Brookhaven Instruments, NY, U.S.A.).
Results and discussion
Design, synthesis and construction of anti-PCT 3D probes
The HBV capsid that consists of 240 subunit proteins (i.e. core proteins) was genetically modified by inserting hexa-histidine peptide (H6) and tandem repeat of B domain of Staphylococcal protein A (SPAB) to the N-terminus and flexible loop region of core protein, respectively (Fig. 1). The modified core proteins were expressed in E. coli and self-assembled into the capsid-like particles (H6-SPAB-capsid) in the cytoplasm. As both of the N-terminus and loop region of native core protein are located on the external surface of HBV capsid,40 the inserted H6 and SPAB were also exposed to the outside of the modified capsid. We then attached anti-PCT polyclonal antibodies (IgGs) onto the modified capsids through specific and strong interaction between SPAB and Fc region of anti-PCT IgGs (Kd = 6.2 × 10−10 M),41 resulting in the construction of anti-PCT 3D probes (Fig. 1).
One-step-immunoassay for accurate and rapid detection of PCT
Recently, we reported a novel one-step-immunoassay method using antibody-conjugated 3D probes involved in pre-assay solution where gold ions (Au3+) were chemisorbed onto the H6 peptides on the 3D probes via coordination bond, and also free Au3+ is present in the same solution. As soon as a patient serum and reducing agent (L-ascorbic acid, LAA) are mixed with the pre-assay solution above, the 3D probes bind to a disease-specific marker, immediately followed by the agglomeration of 3D probe–marker binding complexes. At the same time, the clusters of gold nanoparticles (AuNP) form on the surface of the 3D probe–marker agglomerate through the reduction of both chemisorbed and free Au3+ by LAA, resulting in the formation of large gold particles, which enables self-enhancement of optical signals, measured as optical density (O.D.) at a particular wave length (564 nm in this study, Fig. 2a) (ESI Fig. S2–S4†).42–44 However, this assay method has intrinsic problems, because assay performance is significantly affected not only by serum viscosity that varies depending on individual physiological condition but also by non-specific adsorption of free gold ions of pre-assay solution to various serum proteins, which is associated with molecular diffusion of 3D probes and markers and gold particle formation, respectively.
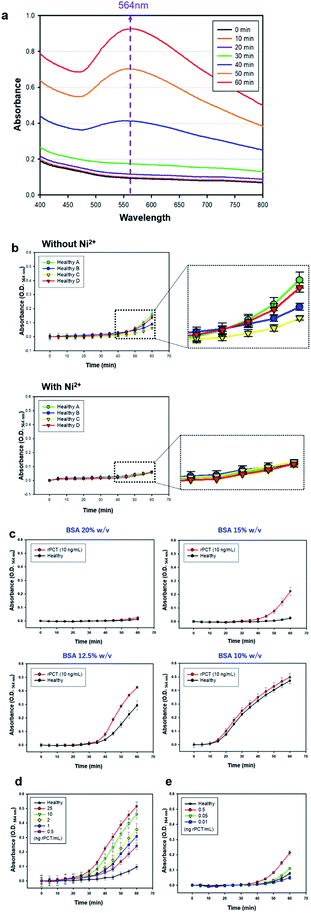 |
| Fig. 2 Results of advanced one-step-immunoassay of PCT. (a) Time-course absorbance profile of the one-step-immunoassay, showing that absorbance is maximized at 564 nm. (b) Time-course change in absorbance at 564 nm (O.D.564 nm) in the one-step-immunoassay of 4 healthy sera with Ni2+ and without Ni2+. (c) Time-course change in O.D.564 nm in the one-step-immunoassay of healthy serum and standard serum solution containing rPCT (10 ng mL−1) with BSA at different concentrations (10, 12.5, 15, and 20% w/v). Time-course change in O.D.564 nm in the one-step-immunoassay of healthy sera and standard serum solutions containing rPCT at different concentrations (d) from 0.5 to 25 ng rPCT per mL and (e) from 0.01 to 0.5 ng rPCT per mL. | |
In this study, we also included polyclonal anti-PCT IgG-conjugated 3D probes and both chemisorbed and free Au3+ in pre-assay solution but obviously improved assay performance through resolving the problems above as follows: (1) nickel ion (Ni2+) was added to each serum before the serum is mixed with pre-assay solution, (2) the volume of serum to be added to the pre-assay solution was minimized, and (3) bovine serum albumin (BSA) was added to assay solution prior to beginning one-step-immunoassay. The addition of Ni2+ to individual serum implies the prior binding of Ni2+ to various serum proteins before each serum sample is mixed with pre-assay solution, which enables consistent and true optical signals by preventing non-specific adsorption of free Au3+ in pre-assay solution to the serum proteins. As shown in Fig. 2b, the addition of Ni2+ (0.04 μL, 25% w/v) to different healthy sera (1.96 μL) did not emit any false signals even at 1 h after the one-step-immunoassay began, while the immunoassay without the Ni2+ addition to the same sera caused a notable false signals during the same period of assay. The reduced serum volume and added BSA contribute towards keeping the viscosity of total assay solution nearly constant, leading to constant diffusion of 3D probes and disease marker molecules and hence helping the markers to be detected by 3D probes with consistent efficiency. Fig. 2c shows that when total assay solution (40 μL) contains 6 μL of BSA (15% w/v) solution, the assay signals between standard serum solution containing rPCT (10 ng mL−1) and healthy sera was the most apparently differentiated. As the concentration of BSA solution decreased to 12.5% w/v or lower, false positive signals from healthy sera appeared more rapidly, which is presumably because the lower content of BSA caused lower viscosity of assay solution, higher molecular diffusion of Au3+ and LAA, and hence higher reduction rate of Au3+, thereby leading to more rapid formation of gold particles even in healthy sera. The limit of detection (LOD) of this assay was estimated in a wide range of PCT concentration (0.01 to 25 ng mL−1) (Fig. 2d and e), showing that the absorbance (O.D.564 nm) gradually decreases as PCT concentration sequentially decreases from 25 to 0.05 ng mL−1 but O.D.564 nm at 0.01 ng PCT per mL is nearly equal to that of healthy sera. Therefore, it seems that the LOD lies between 0.01 and 0.05 ng PCT per mL, indicating that this assay is sensitive enough to accurately diagnose sepsis patients even with mild symptoms.33
Application of one-step-immunoassay to diagnosis of sepsis patients
The one-step-immunoassay was applied to the diagnosis of 30 sepsis patients using 30 healthy sera as negative controls. (Total assay solution (40 μL) contains 24 μL of pre-assay solution, 6 μL of BSA (15% w/v) solution, 2 μL of patient or healthy serum (1.96 μL serum + 0.04 μL Ni2+ (25% w/v) solution), and 8 μL of LAA.) In the assay of patient sera, the appreciable dark blue color began to appear between 35 and 45 min and continuously increased up to the end of assay, or 60 min, while for healthy sera, no color change was observed during the same period of assay, which was measured as O.D.564 nm as presented in Fig. 3a. However, it is worthy of note that O.D.564 nm of two sera (#5 and #14) and one serum (#10) out of 30 patient sera was below and slightly above cut-off-value (COV, calculated as 0.085), respectively, indicating that two false negative and one weak positive signals were observed for the 30 patients who were diagnosed definitely as sepsis (Fig. 3b). The appearance of these wrong signals is presumed due to hook effect. In general, immunoassay signals increase as concentration of target analyte (i.e. antigen) increases, but when concentration of target antigen is much higher than that of capture probes (i.e. the antigen-binding antibodies), the assay signals rather decreases because too much extra antigens prevent signal-generating reporter probes (e.g. enzyme-linked secondary antibodies) from binding to antigen–antibody complexes, which is defined as hook effect.45,46 The hook effect can happen in this one-step-immunoassay as illustrated as Fig. 3c. That is, when PCT concentration is relatively too high compared to 3D probe concentration, the extra PCT can prevent the agglomeration of 3D probe–PCT binding complexes because all anti-PCT IgGs of 3D probes are saturated, or coated with PCT (Fig. 3c).
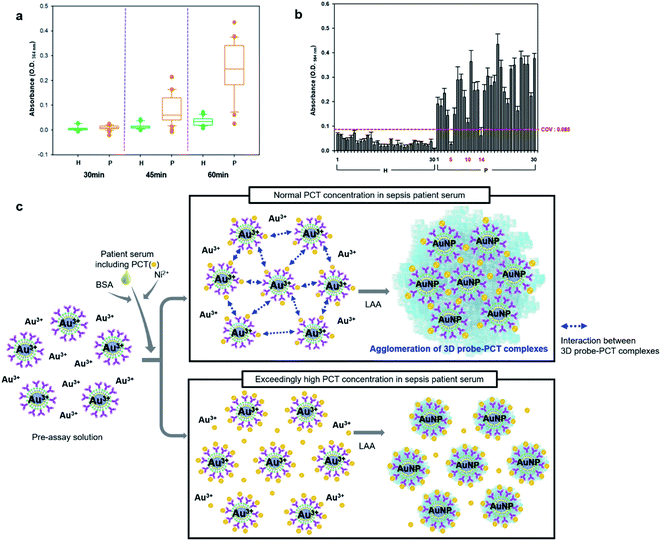 |
| Fig. 3 Hook effect-associated problem in the one-step-immunoassay of PCT. (a) Time-course box plot of O.D.564 nm with quantiles and (b) O.D.564 nm at 60 min in the one-step-immunoassay of PCT using 30 healthy (H) and 30 sepsis patients sera (P) (COV: cut-off-value (the average O.D.564 nm of healthy sera + 3 × standard deviation)). (c) Schematic illustration of hook effect problems in the PCT detection through the one-step-immunoassay. | |
Enhanced diagnostic accuracy by resolving hook effect problem in the detection of PCT
When all the PCT-binding sites of 3D probes are occupied by PCT, the interaction between the 3D probe–PCT complexes is totally interfered, causing significantly lowered assay signals even at high PCT concentrations. In patients with severe sepsis, serum concentration of PCT is known to be 100–200 ng mL−1.47,48 We used the same pre-assay solution containing anti-PCT 3D probes at the concentration of 0.005 mg mL−1, which was used for the one-step-immunoassays of PCT at the concentrations of 0.05–25 ng mL−1 (Fig. 2 and 3) to detect PCT at much higher concentrations (50–200 ng mL−1), and then the assay signals notably decreased as presented in Fig. 4a. When a new pre-assay solution containing the anti-PCT 3D probes at 10-times higher concentration (0.05 mg mL−1) was used in the same immunoassays of Fig. 4a, the assay signals dramatically increased, i.e. increased proportionally to the PCT concentration in a higher range (50 to 200 ng mL−1) (Fig. 4b). Further, the new pre-assay solution was used to diagnose sepsis using the patient sera #5, #10, and #14 of Fig. 3b, and unlike the results of Fig. 3b, true positive signals were generated from all the three sera (Fig. 4c and d), indicating that #5, #10, and #14 are the sera of severe sepsis patients with high PCT concentration. Through increasing anti-PCT 3D probes in the pre-assay solution, the lowered assay signals by hook effect were successfully corrected to true positive signals.
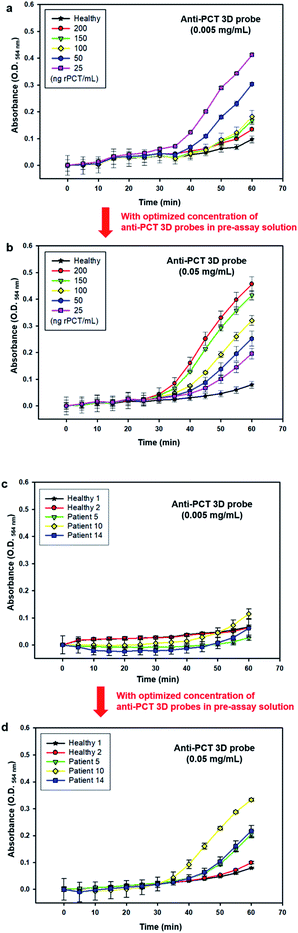 |
| Fig. 4 Improvement of one-step-immunoassay through resolving the hook effect-associated problem of Fig. 3. Time-course change in O.D.564 nm in the one-step-immunoassay of standard serum solutions containing rPCT at the concentration of 25 to 200 ng mL−1 using the pre-assay solution containing anti-PCT 3D probe at the concentration of (a) 0.005 mg mL−1 and (b) 0.05 mg mL−1. Time-course change in O.D.564 nm in the one-step-immunoassay of two healthy and three sepsis patient sera (#5, #10, and #14, showing false negative signals in Fig. 3b) using the pre-assay solution containing anti-PCT 3D probe at the concentration of (c) 0.005 mg mL−1 and (d) 0.05 mg mL−1. | |
Quantitative diagnostic performance: one-step-immunoassay vs. CMIA
The detection of serum PCT is now commercially performed through CMIA, which is available at Green Cross Laboratory. Standard serum solutions containing PCT were prepared through spiking rPCT in healthy sera at the concentrations of 0.5–200 ng mL−1 and were tested using CMIA at Green Cross Laboratory. From Fig. 5a, 0.5 ng PCT per mL serum, a threshold concentration below which the individual is not regarded as sepsis patient, was shown to be below LOD of CMIA. The highest PCT concentration, 200 ng mL−1, was not clearly measured either, because the concentration is likely beyond the detection range (Fig. 5a). Furthermore, it is worthy of note that the PCT concentrations measured by CMIA poorly correlated with the standard PCT concentrations (Fig. 5b). However, as presented in Fig. 5c and d, the assay signals measured by one-step-immunoassay show a fairly good linearity with the standard PCT concentrations in the entire range of concentration (0.05–200 ng mL−1).
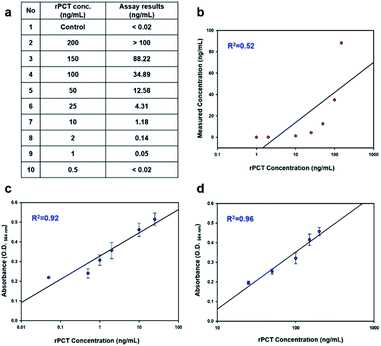 |
| Fig. 5 Quantitative assays of PCT: CMIA vs. advanced one-step-immunoassay. (a) Results of CMIA using standard serum solutions containing rPCT at the concentration of 0.5 to 200 ng mL−1. (b) Linearity between measured signals and actual rPCT concentrations for the assay results of (a). Linearity between measured signals and actual rPCT concentrations for the results of advanced one-step-immunoassay of standard serum solutions containing rPCT (c) at the low and medium concentrations of rPCT (0.05 to 25 ng mL−1) and (d) at the high concentrations of rPCT (25 to 200 ng mL−1). (The concentration of anti-PCT 3D probe in pre-assay solution was 0.005 mg mL−1 in (c) and 0.05 mg mL−1 in (d).). | |
Conclusions
One-step-immunoassay using pre-assay solution containing 3D probes (i.e. anti-marker polyclonal IgGs attached on the modified capsid that is constructed through inserting H6 and SPAB onto the external surface of HBV capsid) and both the 3D probe-chemisorbed and free Au3+ enables self-enhancement of optical signals at a particular wave length. This immunoassay is based on disease marker-induced formation of gold nanoparticle clusters on the surface of the 3D probe-marker agglomerates in assay solution. Although the one-step-immunoassay is highly efficient in terms of assay speed and convenience, it suffers from the following intrinsic problems: (1) non-specific adsorption of free Au3+ in pre-assay solution to various serum proteins, which hampers marker concentration-dependent generation of assay signals and (2) severe variation in viscosity of total assay solution depending on added serum viscosity, which significantly affects diffusion of 3D probes, markers, free Au3+, and reducing agent in assay solution and often results in inconsistent assay signals.
For resolving these problems, we modified the one-step-immunoassay as follows: (1) nickel ion (Ni2+) was added to each serum before the serum is mixed with pre-assay solution, which always enables the generation of true positive signals for all sepsis patients by preventing non-specific adsorption of free Au3+ to the serum proteins and (2) bovine serum albumin (BSA) was added to assay solution prior to beginning one-step-immunoassay with minimizing the volume of serum to be added to the pre-assay solution, which contributes towards keeping the viscosity of total assay solution nearly constant, leading to constant diffusion of all assay components (3D probes, disease marker, Au3+, and reducing agent) and hence helping the markers to be detected with consistent efficiency. The LOD of this modified one-step-immunoassay lies between 0.01 and 0.05 ng PCT per mL, indicating that this assay is sensitive enough to accurately diagnose sepsis patients even at an early stage.
Furthermore, to avoid hook effect-associated problem that happens when PCT concentration is relatively too high compared to 3D probe concentration and hence the extra PCT prevents the normal agglomeration of 3D probe–PCT binding complexes, we optimized the 3D probe concentration in pre-assay solution, and as a result, the false negative signals from the severe sepsis patients with high PCT concentration was successfully converted to true positive signals. Standard serum samples containing PCT at the concentrations of 0.5 to 200 ng mL−1 were also tested using CMIA, but the assay signals poorly correlated with the standard PCT concentrations, while the one-step-immunoassay signals from the same standard serum samples show a fairly good linearity with the entire range of PCT concentration. Although the diagnosis of sepsis is demonstrated as a proof-of-concept here, this approach can provide quantitative accuracy in the detection of other diseases markers as well as high assay speed and convenience and therefore can be used as a general diagnostic platform for point-of-care testing of various bacterial infection-related diseases.
Conflicts of interest
There are no conflicts to declare.
Acknowledgements
This study was supported by Cellemedy Inc. and the National Research Foundation of Korea (NRF) grant (Grant No. 2019R1A2C3005771).
References
- K. Kamalpreet, M. Rajiv and T. Aparna, A novel marker procalcitonin may help stem the antibiotic overuse in emergency setting, Int. J. Appl. Basic Med. Res., 2013, 3, 77–83 CrossRef PubMed.
- M. Singer, C. S. Deutschman, C. W. Seymour, M. Shankar-Hari, D. Annane, M. Bauer, R. Bellomo, G. R. Bernard, J. D. Chiche, C. M. Coopersmith, R. S. Hotchkiss, M. M. Levy, J. C. Marshall, G. S. Martin, S. M. Opal, G. D. Rubenfeld, T. van der Poll, J. L. Vincent and D. C. Angus, The third international consensus definition for sepsis and septic shock (Sepsis-3), JAMA, 2016, 315, 801–810 CrossRef CAS PubMed.
- D. C. Angus and T. van der Poll, Severe sepsis and septic shock, N. Engl. J. Med., 2013, 369, 840–851 CrossRef CAS PubMed.
- A. Lever and I. Mackenzie, Sepsis: definition, epidemiology, and diagnosis, BMJ [Br. Med. J.], 2007, 335, 879–883 CrossRef CAS PubMed.
- G. S. Martin, D. M. Mannino, S. Eaton and M. Moss, The epidemiology of sepsis in the United States from 1979 to 2000, N. Engl. J. Med., 2003, 348, 1546–1554 CrossRef PubMed.
- M. Y. Memar, M. Varshochi, B. Shokouhi, M. Asgharzadeh and H. S. Kafil, Procalcitonin: marker of pediatric bacterial infection, Biomed. Pharmacother., 2017, 96, 936–943 CrossRef CAS PubMed.
- J. A. Russell, Management of sepsis, N. Engl. J. Med., 2006, 355, 1699–1713 CrossRef CAS PubMed.
- M. M. Levy, M. P. Fink, J. C. Marshall, E. Abraham, D. Angus, D. Cook, J. Cohen, S. M. Opal, J. L. Vincent and G. Ramsay, 2001 sccm/esicm/accp/ats/sis international sepsis definitions conference, Intensive Care Med., 2003, 29, 530–538 CrossRef PubMed.
- C. Pierrakos and J.-L. Vincent, Sepsis biomarkers: a review, Crit. Care, 2010, 14, R15 CrossRef PubMed.
- S. D. Carrigan, G. Scott and M. Tabrizian, To resolve the challenges of sepsis diagnosis, Clin. Chem., 2004, 50, 1301–1314 CrossRef CAS PubMed.
- B. M. P. Tang, G. D. Eslick, J. C. Craig and A. S. McLean, Accuracy of procalcitonin for sepsis diagnosis in critically ill patients: Systematic review and meta-analysis, Lancet Infect. Dis., 2007, 7, 210–217 CrossRef CAS PubMed.
- M. Christ-Crain and B. Mūller, Biomarkers in respiratory tract infections: diagnostic guides to antibiotic prescription, prognostic markers and mediators, Eur. Respir. J., 2007, 30, 556–573 CrossRef CAS PubMed.
- P. Ohlsson, K. Petersson, P. Augustsson and T. Laurell, Acoustic impedance-matched buffers enable the separation of bacteria from blood cells at high cell concentrations, Sci. Rep., 2018, 8, 1–11 CAS.
- P. Tissari, A. Zumla, E. Tarkka, S. Mero, L. Savolainen, M. Vaara, A. Aittakorpi, S. Laakso, M. Lindfors, H. Piiparinen, M. Mäki, C. Carder, J. Huggett and V. Gant, Accurate and rapid identification of bacterial species from positive blood cultures using a DNA-based microarray platform: an observational study, Lancet, 2010, 375, 224–230 CrossRef CAS.
- D.-K. KANG, M. Monsur Ali, K. Zhang, S. S. Huang, E. Peterson, M. A. Digman, E. Gratton and W. Zhao, Rapid detection of single bacteria in unprocessed blood using Integrated Comprehensive Droplet Digital Detection, Nat. Commun., 2014, 5, 1–10 Search PubMed.
- G. Yang, X. Meng, Y. Wang, M. Yan, Z. P. Aguilar and H. Xu, 2-Step lectin-magnetic separation (LMS) strategy combined with AuNP-based colorimetric system for S. aureus detection in blood, Sens. Actuators, B, 2019, 279, 87–94 CrossRef CAS.
- L. E. Lehmann, K.-P. Hunfeld, M. Steinbrucker, V. Brade, M. Book, H. Seifert, T. Bingold, A. Hoeft, H. Wissing and F. Stüber, Improved detection of bloodstream pathogens by real-time PCR in severe sepsis, Intensive Care Med., 2010, 36, 49–56 CrossRef CAS PubMed.
- A. Lee, S. Mirrett, L. B. Reller and M. P. Weinstein, Detection of bloodstream infections in adults: How many blood cultures are needed?, J. Clin. Microbiol., 2007, 45, 3546–3548 CrossRef PubMed.
- J.-C. Cheng, C.-L. Huang, C.-C. Lin, C.-C. Chen, Y.-C. Chang, S.-S. Chang and C.-P. Tseng, Rapid detection and identification of clinically important bacteria by high-resolution melting analysis after broad-range ribosomal RNA real-time PCR, Clin. Chem., 2006, 52, 1997–2004 CrossRef CAS PubMed.
- S. Juul, C. J. F. Nielsen, R. Labouriau, A. Roy, C. Tesauro, P. W. Jensen, C. Harmsen, E. L. Kristoffersen, Y.-L. Chiu, R. Frøhlich, P. Fiorani, J. Cox-Singh, D. Tordrup, J. Koch, A.-L. Bienvenu, A. Desideri, S. Picot, E. Petersen, K. W. Leong, Y.-P. Ho, M. Stougaard and B. R. Knudsen, Droplet microfluidics platform for highly sensitive and quantitative detection of malaria-causing Plasmodium parasites based on enzyme activity measurement, ACS Nano, 2012, 6, 10676–10683 CrossRef CAS PubMed.
- G. J. Adema and P. D. Baas, A novel calcitonin-encoding mRNA is produced by alternative processing of calcitonin/calcitonin gene-related peptide-I pre-mRNA, J. Biol. Chem., 1992, 11, 7943–7948 CrossRef.
- D. Gendrel and C. Bohuon, Procalcitonin, a marker of bacterial infection, Infection, 1997, 25, 133–134 CrossRef CAS PubMed.
- A. M. C. Van Rossum, R. W. Wulkan and A. M. Oudesluys-Murphy, Procalcitonin as an early marker of infection in neonates and children, Lancet Infect. Dis., 2004, 4, 620–630 CrossRef CAS PubMed.
- M. Assicot, D. Gendrel, H. Carsin, J. Raymond, J. Guilbaud and C. Bohuon, High serum procalcitonin concentrations in patients with sepsis and infection, Lancet, 1993, 341, 515–518 CrossRef CAS.
- B. Al-Nawas, I. Krammer and P. M. Shah, Procalcitonin in the diagnosis of severe infections, Eur. J. Med. Res., 1996, 1, 331–333 CAS.
- P. Maruna, K. Nedelnikova and R. Gurlich, Physiology and genetics of procalcitonin, Physiol. Res., 2000, 49, S57–S62 CAS.
- P. Dandona, D. Nix, M. F. Wilson, A. Aljada, J. Love, M. Assicot and C. Bohuon, Procalcitonin levels increased after endotoxin injection in normal subjects, J. Clin. Endocrinol. Metab., 1994, 79, 1605–1608 CAS.
- C. Clech, F. Ferriere, P. Karoubi, J. P. Fosse, M. Cupa, P. Hoang and Y. Cohen, Diagnostic and prognostic value of procalcitonin in patients with septic shock, Crit. Care Med., 2004, 32, 1166–1169 CrossRef PubMed.
- L. Simon, F. Gauvin, D. K. Amre, P. Saint-Louis and J. Lacroix, Serum procalcitonin and C-reactive protein levels as markers of bacterial infection: a systematic review and meta-analysis, Clin. Infect. Dis., 2004, 39, 206–217 CrossRef CAS PubMed.
- M. Christ-Carin and B. Müller, Procalcitonin in bacterial infection, hype, hope, more or less?, Swiss Med. Wkly., 2005, 135, 451–460 Search PubMed.
- A. Galetto-Lacour, S. A. Zamora and A. Gervaix, Bedside procalcitonin and C-reactive protein tests in children with fever without localizing signs of infection seen in a referral center, Pediatrics, 2003, 112, 1054–1060 CrossRef PubMed.
- B. Uzzan, R. Cohen, P. Nicolas, M. Cucherat and G.-Y. Perret, Procalcitonin as a diagnostic test for sepsis in critically ill adults and after surgery or trauma: a systematic review and meta-analysis, Crit. Care Med., 2006, 34, 1996–2003 CrossRef CAS PubMed.
- M. Meisner, Update procalcitonin measurements, Ann. Lab. Med., 2014, 34, 263–273 CrossRef CAS PubMed.
- M. Meisner, F. M. Brunkhorst, H. B. Reith, J. Schmidt, H. G. Lestin and K. Reinhart, Clinical experiences with a new semi-quantitative solid-phase immunoassay for rapid measurement of procalcitonin, Clin. Chem. Lab. Med., 2000, 38, 989–995 CAS.
- B. Kocazeybek, S. Kucukoglu and Y. A. Öner, Procalcitonin and C-reactive protein levels in infective endocarditis: correlation with etiology and prognosis, Chemotherapy, 2003, 49, 76–84 CrossRef CAS PubMed.
- R. H. Snider Jr, H. Richard, E. S. Nylen and K. L. Becker, Procalcitonin and its component peptides in systemic inflammation: immunochemical characterization, J. Invest. Med., 1997, 45, 552–560 CAS.
- K. T. Whang, P. M. Steinwald, J. C. White, E. S. Nylen, R. H. Snider, G. L. Simon, R. L. Goldberg and K. L. Becker, Serum calcitonin precursors in sepsis and systemic inflammation, J. Clin. Endocrinol. Metab., 1998, 83, 3296–3301 CAS.
- M. Oberhoffer, H. Vogelsang, L. Jäger and K. Reinhart, Katacalcin and calcitonin immunoreactivity in different types of leukocytes indicate intracellular procalcitonin content, J. Crit. Care, 1999, 14, 29–33 CrossRef CAS PubMed.
- K. C. Kwon, J. H. Ryu, J.-H. Lee, E. J. Lee, I. C. Kwon and K. Kim, J. Lee. Proteinticle/gold core/shell nanoparticles for targeted cancer therapy without nanotoxicity, Adv. Mater., 2014, 26, 6436–6441 CrossRef CAS PubMed.
- S. A. Wynne, R. A. Crowther and A. G. W. Leslie, The crystal structure of the human hepatitis B virus capsid, Mol. Cell, 1999, 3, 771–780 CrossRef CAS PubMed.
- E. J. Lee, E. Lee, H. J. Kim, J.-H. Lee, K.-Y. Ahn, J.-S. Park and J. Lee, Self-assembled proteinticle nanostructures for 3-dimensional display of antibodies, Nanoscale, 2014, 6, 14919–14925 RSC.
- J. H. Kwon, H.-T. Kim, J.-H. Lee, R. Kim, M. Heo, J. Shin, H.-Y. Lee and Y. J. Cha, J. Lee. Signal self-enhancement by coordinated assembly of gold nanoparticles enables accurate one-step-immunoassays, Nanoscale, 2017, 9, 16476–16484 RSC.
- J. H. Kwon, H. T. Kim, S. J. Sim, Y. J. Cha and J. Lee, Performance of point-of-care diagnosis of AIDS: label-free one-step-immunoassay vs. lateral flow assay, Analyst, 2018, 143, 936–942 RSC.
- J. H. Kwon, H. H. Kim, H. B. Cho, Y. J. Cha and J. Lee, Viral antigen nanoparticles for discriminated and quantitative detection of different subtypes of anti-virus immunoglobulins, Nanoscale, 2019, 11, 18282–18289 RSC.
- S. A. Fernando and G. S. Wilson, Studies of the ‘hook’ effect in the one-step sandwich immunoassay, J. Immunol. Methods, 1992, 151, 47–66 CrossRef CAS PubMed.
- R. A. Haddad, D. Giachirio and A. L. Barkan, Interpretation of common endocrine laboratory tests: technical pitfalls, their mechanisms and practical considerations, Clin. Diabetes Endocrinol., 2019, 5, 12 CrossRef PubMed.
- M. J. Ruiz-Alvarez, S. García-Valdecasas, R. De Pablo, M. Sanchez García, C. Coca, T. W. Groeneveld, A. Roos, M. R. Daha and I. Arribas, Diagnostic efficacy and prognostic value of serum procalcitonin concentration in patients with suspected sepsis, J. Intensive Care Med., 2009, 24, 63–71 CrossRef CAS PubMed.
- D. von Heimburg, W. Stieghorst, R. Khorram-Sefat and N. Pallua, Procalcitonin—a sepsis parameter in severe burn injuries, Burns, 1998, 24, 745–750 CrossRef CAS PubMed.
Footnote |
† Electronic supplementary information (ESI) available. See DOI: 10.1039/d1ra02494a |
|
This journal is © The Royal Society of Chemistry 2021 |
Click here to see how this site uses Cookies. View our privacy policy here.