DOI:
10.1039/D1RA02297K
(Paper)
RSC Adv., 2021,
11, 13040-13046
Catalytic enantioselective bromohydroxylation of cinnamyl alcohols†
Received
23rd March 2021
, Accepted 23rd March 2021
First published on 7th April 2021
Abstract
This work describes an effective enantioselective bromohydroxylation of cinnamyl alcohols with (DHQD)2PHAL as the catalyst and H2O as the nucleophile, providing a variety of corresponding optically active bromohydrins with up to 95% ee.
Electrophilic halogenation of olefins allows installation of two stereogenic centers onto the C–C double bond and is one of the most important transformations in organic chemistry.1 Optically active halogen containing products resulting from asymmetric halogenation would serve as versatile chiral building blocks for organic synthesis. As a result, extensive efforts have been devoted to the development of asymmetric halogenation process. In recent years, great progress has been made in both intramolecular2,3 and intermolecular4,5 reaction processes with various types of olefins and nucleophiles. However, there are still challenges remaining to be addressed. In many cases, the developed catalytic systems often only apply to certain ranges of substrates and the reaction reactivity as well as selectivity can't be rationally adjusted. The substrate scope is also often difficult to be logically extended and requires much experimentation, largely due to the complexity of the reaction systems and the lack of clear understanding of the reaction mechanisms.
Halohydroxylation of olefins simply with H2O as nucleophile is a classic electrophilic addition reaction in organic chemistry and produces synthetically useful halohydrins (Scheme 1). Asymmetric version of such process has been challenging with only a few reports.6,7 As part of our general intertest in asymmetric halogenation,8 recently we have been investigating the intermolecular asymmetric reaction processes, particularly with unfunctionalized olefins, which has been a long standing challenging problem. During such studies, we have found that up to 92% ee could be achieved for the bromoesterification of unfunctionalized olefins with (DHQD)2PHAL (Scheme 2, eqn (a)).9 This work represents an early example of asymmetric halogenation for unfunctionalized olefins with high enantioselectivity. To our delight, high enantioselectivity can also be achieved for bromohydroxylation with H2O upon further investigation, giving optically active bromohydrins with up to 98% ee (Scheme 2, eqn (b)).10 In our efforts to expand the reaction scope of the asymmetric bromohydroxylation, we have found that cinnamyl alcohols are effective substrates, giving the corresponding bromohydrins with up to 95% ee. Herein, we report our preliminary studies on this subject.
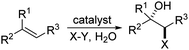 |
| Scheme 1 Asymmetric halohydroxylation of olefins. | |
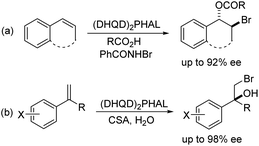 |
| Scheme 2 Asymmetric oxybromination of olefins. | |
Initial studies were carried out with (E)-3-(4-bromophenyl)prop-2-en-1-ol (1a) as substrate. Several bromine reagents were examined with 10 mol% (DHQD)2PHAL (3a) (Fig. 1) as the catalyst and 10 mol% (−)-camphorsulfonic acid (CSA) as additive in acetone/H2O (10
:
1) at −30 °C (Table 1, entries 1–5). N-Bromobenzamide gave the highest ee (76%) (Table 1, entry 5). Among the catalysts investigated (Table 1, entries 5–9), (DHQD)2PHAL (3a) was the choice of the catalyst with N-bromobenzamide. Solvent studies (Table 1, entries 5 and 10–15) showed that the highest ee (83%) was obtained with CH3CN/H2O (10
:
1) (Table 1, entry 10). Addition of 10 mol% (−)-CSA increased both yield and ee (Table 1, entry 10 vs. 16). The best result was obtained with (−)-CSA among the additives examined (Table 1, entry 10 vs. entries 17–21). Slightly higher ee (85%) but lower yield was obtained when the reaction temperature was lowered to −40 °C (Table 1, entry 22 vs. 10).
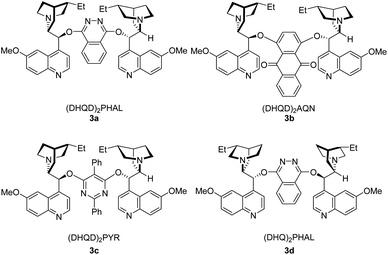 |
| Fig. 1 Selected examples of catalyst examined. | |
Table 1 Studies on reaction conditionsa
With the optimized reaction conditions in hand, the substrate scope was subsequently investigated with 10 mol% (DHQD)2PHAL (3a), N-bromobenzamide (1.2 eq.), and 10 mol% (−)-CSA in CH3CN/H2O (10
:
1) at −30 °C. As shown in Table 2, the bromohydroxylation can be extended to various cinnamyl alcohols, giving the corresponding bromohydrins in 46–87% yields and 55–95% ee's (Table 2, entries 1–17). The reaction outcome was significantly influenced by the substituent on the phenyl group. In general, the enantioselectivity increased as a substituent was introduced onto the phenyl group. For mono-substituted substrates, it appeared that higher ee was obtained with the para-substituent (Table 2, entry 5 vs. 6 vs. 7). Up to 90% ee was achieved with p-Ph substituted cinnamyl alcohol (Table 2, entry 4). For 4-substituted substrates, the enantioselectivity remained similar when a second Me group was introduced to the 3 position (Table 2, entries 9–12). However, significantly higher ee's were obtained when the Me group was introduced to the 2-position, giving the corresponding bromohydrins in 90–95% ee (Table 2, entries 13–17). With 2-Me, 4-Br-substituted cinnamyl alcohol (1m), MeOH was also found to be effective nucleophile, giving the corresponding bromoether (2r) in 75% yield and 90% ee (Table 2, entry 18). A similar ee but lower yield was obtained when the hydroxyl group was replaced with the MeO group, giving the bromohydrin (2s) in 31% yield and 80% ee (Table 2, entry 19). The exact reason for this difference is not clear at this moment.
Table 2 Asymmetric bromohydroxylation of cinnamyl alcoholsa

|
Entry |
Substrate |
Product |
Yieldb (%) |
eec (%) |
Reactions were carried out with substrate 1 (0.50 mmol), (DHQD)2PHAL (0.050 mmol), (−)-CSA (0.050 mmol), and PhCONHBr (0.60 mmol) in CH3CN (5.0 mL) and water (0.50 mL) at −30 °C for 72 h unless otherwise noted. Isolated yield. Determined by chiral HPLC analysis. For entry 1, the absolute configuration was determined by comparing the optical rotation of the corresponding epoxide with the reported one11 upon treatment with K2CO3 in acetone (Scheme 3). For others, the absolute configurations were tentatively assigned by analogy. The reaction was carried out at −40 °C for 168 h. MeOH was used as nucleophile. |
|
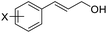 |
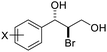 |
|
|
1 |
X = p-Br, 1a |
2a (X-ray) |
70 |
83 |
2 |
X = p-Cl, 1b |
2b |
64 |
80 |
3 |
X = p-F, 1c |
2c |
75 |
76 |
4d |
X = p-Ph, 1d |
2d |
87 |
90 |
5 |
X = p-Me,1e |
2e (X-ray) |
76 |
82 |
6 |
X = m-Me,1f |
2f |
71 |
62 |
7 |
X = o-Me,1g |
2g |
77 |
70 |
8 |
X = H,1h |
2h |
46 |
55 |
|
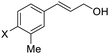 |
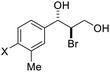 |
|
|
9 |
X = Br, 1i |
2i |
70 |
80 |
10 |
X = Cl, 1j |
2j |
71 |
80 |
11 |
X = F, 1k |
2k |
84 |
80 |
12 |
X = Me, 1l |
2l |
73 |
82 |
|
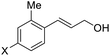 |
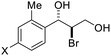 |
|
|
13 |
X = Br, 1m |
2m |
72 |
95 |
14 |
X = Cl, 1n |
2n |
78 |
94 |
15 |
X = F, 1o |
2o |
83 |
91 |
16 |
X = Ph, 1p |
2p |
84 |
94 |
17 |
X = Me, 1q |
2q |
87 |
90 |
18e |
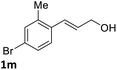 |
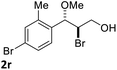 |
75 |
90 |
19 |
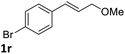 |
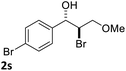 |
31 |
80 |
The absolute configuration of bromohydrin 2a was determined by converting it to the corresponding epoxide 4 with K2CO3 (Scheme 3) and comparing the optical rotation of the epoxide with the reported one.11 The bromohydroxylation reaction can also be carried out on a relatively large scale. For example, 1.1341 g of bromohydrin 2m was obtained in 70% yield with 95% ee (Scheme 4). As shown in Scheme 5, bromohydrin 2m can be converted to bromoacetal 5 in 86% yield without loss of the ee. Sulfide 6 was obtained in 65% yield and 95% ee when 2m was reacted with sodium thiophenolate.
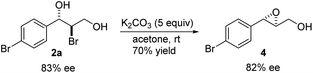 |
| Scheme 3 Determination of absolute configuration of bromohydrin 2a. | |
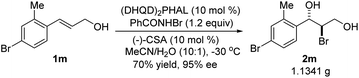 |
| Scheme 4 Bromohydroxylation on gram scale. | |
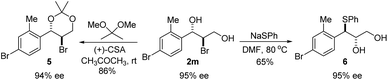 |
| Scheme 5 Synthetic transformations of bromohydrin 2m. | |
Optically active bromoether like 2r could also serve as useful intermediates for further transformations (Scheme 6). Treating 2r with NaN3 in DMF at 80 °C gave azide 7 in 50% yield and 90% ee with inversion of configuration. The bromide of 2r could also be converted to chloride 8 in 90% ee while the yield was somewhat low. Epoxide 9 was obtained in 87% yield and 90% ee by treatment of 2r with NaOH in dioxane and water. When 2r was reacted with PhSNa in DMF at 80 °C, sulfide 10 was isolated in 73% yield and 90% ee. The reaction likely proceeded via epoxide 9. The synthetic application is further illustrated in Scheme 7. Azide 11 and chloride 12 were obtained from 9 in 80% and 78% yield, respectively, without erosion of the optical activity.12
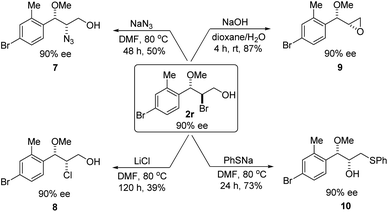 |
| Scheme 6 Synthetic transformations of bromoether 2r. | |
 |
| Scheme 7 Synthetic transformations of epoxide 9. | |
A precise understanding of the reaction mechanism awaits further study. As previously described,10 two possible transition state models are outlined in Fig. 2. The substrate is likely docked in the chiral pocket through π,π-stacking with quinoline of the catalyst. Such π,π-interaction appeared to be enhanced by the substituents on the phenyl groups, consequently leading to the significant increase of the enantioselectivity. In model A, N-bromobenzamide was activated by both the tertiary amine of the catalyst and additive (−)-CSA to increase its electrophility toward the double bond of the reacting substrate. In model B, the tertiary amine of the catalyst could first be protonated by additive (−)-CSA, and N-bromobenzamide would subsequently be activated by the resulting quaternary ammonium salt via hydrogen bonding.
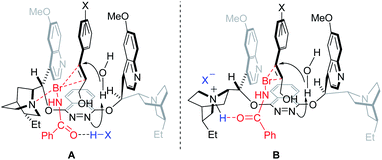 |
| Fig. 2 Two possible transition state models. | |
Conclusions
In summary, bromohydroxylation of olefins is a classic and important electrophilic addition reaction in organic chemistry. Asymmetric version of this reaction process has been challenging. In this work, we have found that cinnamyl alcohols are effective substrates for asymmetric bromohydroxylation with (DHQD)2PHAL as catalyst, (−)-CSA additive, PhCONHBr as bromine source, and H2O as nucleophile, providing the corresponding optically active bromohydrins with up to 95% ee. The resulting bromohydrin and related bromoether can be transformed into various highly functionalized molecules with maintained ee's. The current reaction process represents a significant progress in asymmetric bromohydroxylation. Further understanding reaction mechanism, developing more effective catalyst system, and expanding the substrate scope are currently underway.
Conflicts of interest
There are no conflicts to declare.
Acknowledgements
We gratefully acknowledge the National Natural Science Foundation of China (21632005) and Changzhou University for the financial support. We also thank Miao Zhao at Changzhou University for some experimental contributions.
Notes and references
- For leading reviews on halogenations of olefins, see:
(a) M. D. Dowle and D. I. Davies, Synthesis and synthetic utility of halolactones, Chem. Soc. Rev., 1979, 8, 171 RSC;
(b) G. Li, S. R. S. S. Kotti and C. Timmons, Recent development of regio- and stereoselective aminohalogenation reaction of alkenes, Eur. J. Org. Chem., 2007, 2745 CrossRef CAS;
(c) A. J. Cresswell, S. T.-C. Eey and S. E. Denmark, Catalytic, stereoselective dihalogenation of alkenes: challenges and opportunities, Angew. Chem., Int. Ed., 2015, 54, 15642 CrossRef CAS PubMed.
- For leading reviews on intramolecular asymmetric halogenation of olefins, see:
(a) G. Chen and S. Ma, Enantioselective halocyclization reactions for the synthesis of chiral cyclic compounds, Angew. Chem., Int. Ed., 2010, 49, 8306 CrossRef CAS PubMed;
(b) A. Castellanos and S. P. Fletcher, Current methods for asymmetric halogenation of olefins, Chem.–Eur. J., 2011, 17, 5766 CrossRef CAS PubMed;
(c) C. K. Tan, L. Zhou and Y.-Y. Yeung, Organocatalytic enantioselective halolactonizations: strategies of halogen activation, Synlett, 2011, 1335 CAS;
(d) U. Hennecke, New catalytic approaches towards the enantioselective halogenation of alkenes, Chem.–Asian J., 2012, 7, 456 CrossRef CAS PubMed;
(e) S. E. Denmark, W. E. Kuester and M. T. Burk, Catalytic, asymmetric halofunctionalization of alkenes-a critical perspective, Angew. Chem., Int. Ed., 2012, 51, 10938 CrossRef CAS PubMed;
(f) K. Murai and H. Fujioka, Recent progress in organocatalytic asymmetric halocyclization, Heterocycles, 2013, 87, 763 CrossRef CAS;
(g) S. R. Chemler and M. T. Bovino, Catalytic aminohalogenation of alkenes and alkynes, ACS Catal., 2013, 3, 1076 CrossRef CAS PubMed;
(h) C. K. Tan and Y.-Y. Yeung, Recent advances in stereoselective bromofunctionalization of alkenes using N-bromoamide reagents, Chem. Commun., 2013, 49, 7985 RSC;
(i) C. B. Tripathi and S. Mukherjee, Catalytic enantioselective halocyclizations beyond lactones: emerging routes to enantioenriched nitrogenous heterocycles, Synlett, 2014, 25, 163 CAS;
(j) Y. A. Cheng, W. Z. Yu and Y.-Y. Yeung, Recent advances in asymmetric intra- and intermolecular halofunctionalizations of alkenes, Org. Biomol. Chem., 2014, 12, 2333 RSC;
(k) S. Zheng, C. M. Schienebeck, W. Zhang, H.-Y. Wang and W. Tang, Cinchona alkaloids as organocatalysts in enantioselective halofunctionalization of alkenes and alkynes, Asian J. Org. Chem., 2014, 3, 366 CrossRef CAS.
- For leading references on intramolecular asymmetric halogenation of olefins, see:
(a) T. Inoue, O. Kitagawa, O. Ochiai, M. Shiro and T. Taguchi, Catalytic asymmetric iodocarbocyclization reaction, Tetrahedron Lett., 1995, 36, 9333 CrossRef CAS;
(b) R. B. Grossman and R. J. Trupp, The first reagent-controlled asymmetric halolactonizations. Dihydroquinidine–halogen complexes as chiral sources of positive halogen ion, Can. J. Chem., 1998, 76, 1233 CrossRef CAS;
(c) S. H. Kang, S. B. Lee and C. M. Park, Catalytic enantioselective iodocyclization of γ-hydroxy-cis-alkenes, J. Am. Chem. Soc., 2003, 125, 15748 CrossRef CAS PubMed;
(d) M. Wang, L. X. Gao, W. P. Mai, A. X. Xia, F. Wang and S. B. Zhang, Enantioselective iodolactonization catalyzed by chiral quaternary ammonium salts derived from cinchonidine, J. Org. Chem., 2004, 69, 2874 CrossRef CAS PubMed;
(e) A. Sakakura, A. Ukai and K. Ishihara, Enantioselective halocyclization of polyprenoids induced by nucleophilic phosphoramidites, Nature, 2007, 445, 900 CrossRef CAS PubMed;
(f) D. C. Whitehead, R. Yousefi, A. Jaganathan and B. Borhan, An organocatalytic asymmetric chlorolactonization, J. Am. Chem. Soc., 2010, 132, 3298 CrossRef CAS PubMed;
(g) W. Zhang, S. Zheng, N. Liu, J. B. Werness, I. A. Guzei and W. Tang, Enantioselective bromolactonization of conjugated (Z)-enynes, J. Am. Chem. Soc., 2010, 132, 3664 CrossRef CAS PubMed;
(h) L. Zhou, C. K. Tan, X. Jiang, F. Chen and Y.-Y. Yeung, Asymmetric bromolactonization Using amino-thiocarbamate catalyst, J. Am. Chem. Soc., 2010, 132, 15474 CrossRef CAS PubMed;
(i) G. E. Veitch and E. N. Jacobsen, Tertiary aminourea-catalyzed enantioselective iodolactonization, Angew. Chem., Int. Ed., 2010, 49, 7332 CrossRef CAS PubMed;
(j) K. Murai, T. Matsushita, A. Nakamura, S. Fukushima, M. Shimura and H. Fujioka, Asymmetric bromolactonization catalyzed by a C3-symmetric chiral trisimidazoline, Angew. Chem., Int. Ed., 2010, 49, 9174 CrossRef CAS PubMed;
(k) U. Hennecke, C. H. Müller and R. Fröhlich, Enantioselective haloetherification by asymmetric opening of meso-halonium ions, Org. Lett., 2011, 13, 860 CrossRef CAS PubMed;
(l) Z.-M. Chen, Q.-W. Zhang, Z.-H. Chen, H. Li, Y.-Q. Tu, F.-M. Zhang and J.-M. Tian, Organocatalytic asymmetric halogenation/semipinacol rearrangement: highly efficient synthesis of chiral α-oxa-quaternary β-haloketones, J. Am. Chem. Soc., 2011, 133, 8818 CrossRef CAS PubMed;
(m) O. Lozano, G. Blessley, T. M. D. Campo, A. L. Thompson, G. T. Giuffredi, M. Bettati, M. Walker, R. Borman and V. Gouverneur, Organocatalyzed enantioselective fluorocyclizations, Angew. Chem., Int. Ed., 2011, 50, 8105 CrossRef CAS PubMed;
(n) V. Rauniyar, A. D. Lackner, G. L. Hamilton and F. D. Toste, Asymmetric electrophilic fluorination using an anionic chiral phase-transfer catalyst, Science, 2011, 334, 1681 CrossRef CAS PubMed;
(o) S. E. Denmark and M. T. Burk, Enantioselective bromocycloetherification by Lewis base/chiral Brønsted acid cooperative catalysis, Org. Lett., 2012, 14, 256 CrossRef CAS PubMed;
(p) M. C. Dobish and J. N. Johnston, Achiral counterion control of enantioselectivity in a Brønsted acid catalyzed iodolactonization, J. Am. Chem. Soc., 2012, 134, 6068 CrossRef CAS PubMed;
(q) M. T. Bovino and S. R. Chemler, Catalytic enantioselective alkene aminohalogenation/cyclization involving atom transfer, Angew. Chem., Int. Ed., 2012, 51, 3923 CrossRef CAS PubMed;
(r) D. H. Paull, C. Fang, J. R. Donald, A. D. Pansick and S. F. Martin, Bifunctional catalyst promotes highly enantioselective bromolactonizations to generate stereogenic C-Br Bonds, J. Am. Chem. Soc., 2012, 134, 11128 CrossRef CAS PubMed;
(s) H. J. Lee and D. Y. Kim, Catalytic enantioselective bromolactonization of alkenoic acids in the presence of palladium complexes, Tetrahedron Lett., 2012, 53, 6984 CrossRef CAS;
(t) J. E. Tungen, J. M. J. Nolsøe and T. V. Hansen, Asymmetric iodolactonization utilizing chiral squaramides, Org. Lett., 2012, 14, 5884 CrossRef CAS PubMed;
(u) K. Ikeuchi, S. Ido, S. Yoshimura, T. Asakawa, M. Inai, Y. Hamashima and T. Kan, Catalytic desymmetrization of cyclohexadienes by asymmetric bromolactonization, Org. Lett., 2012, 14, 6016 CrossRef CAS PubMed;
(v) X. Zeng, C. Miao, S. Wang, C. Xia and W. Sun, Asymmetric 5-endo chloroetherification of homoallylic alcohols toward the synthesis of chiral β-chlorotetrahydrofurans, Chem. Commun., 2013, 49, 2418 RSC;
(w) F. Romanov-Michailidis, L. Guénée and A. Alexakis, Enantioselective organocatalytic fluorination-induced Wagner–Meerwein rearrangement, Angew. Chem., Int. Ed., 2013, 52, 9266 CrossRef CAS PubMed;
(x) C. B. Tripathi and S. Mukherjee, Catalytic enantioselective iodoetherification of oximes, Angew. Chem., Int. Ed., 2013, 52, 8450 CrossRef CAS PubMed;
(y) Q. Yin and S.-L. You, Enantioselective chlorocyclization of indole derived benzamides for the synthesis of spiro-indolines, Org. Lett., 2013, 15, 4266 CrossRef CAS PubMed;
(z) A. Armstrong, D. C. Braddock, A. X. Jones and S. Clark, Catalytic asymmetric bromolactonization reactions using (DHQD)2PHAL-benzoic acid combinations, Tetrahedron Lett., 2013, 54, 7004 CrossRef CAS;
(a
a) W. Xie, G. Jiang, H. Liu, J. Hu, X. Pan, H. Zhang, X. Wan, Y. Lai and D. Ma, Highly enantioselective bromocyclization of tryptamines and its application in the synthesis of (-)-chimonanthine, Angew. Chem., Int. Ed., 2013, 52, 12924 CrossRef CAS PubMed;
(a
b) X. Han, C. Dong and H.-B. Zhou, C3-Symmetric cinchonine-squaramide-catalyzed asymmetric chlorolactonization of styrene-type carboxylic acids with 1,3-dichloro-5,5-dimethylhydantoin: an efficient method to chiral isochroman-1-ones, Adv. Synth. Catal., 2014, 356, 1275 CrossRef CAS;
(a
c) C.-L. Zhu, J.-S. Tian, Z.-Y. Gu, G.-W. Xing and H. Xu, Iron(II)-catalyzed asymmetric intramolecular olefin aminochlorination using chloride ion, Chem. Sci., 2015, 6, 3044 RSC;
(a
d) Y. Cai, P. Zhou, X. Liu, J. Zhao, L. Lin and X. Feng, Diastereoselectively switchable asymmetric haloaminocyclization for the synthesis of cyclic sulfamates, Chem.–Eur. J., 2015, 21, 6386 CrossRef CAS PubMed;
(a
e) Y.-M. Yu, Y.-N. Huang and J. Deng, Catalytic asymmetric chlorocyclization of 2-vinylphenylcarbamates for Synthesis of 1,4-Dihydro-2H-3,1-benzoxazin-2-one Derivatives, Org. Lett., 2017, 19, 1224 CrossRef CAS PubMed;
(a
f) R. Nishiyori, A. Tsuchihashi, A. Mochizuki, K. Kaneko, M. Yamanaka and S. Shirakawa, Design of chiral bifunctional dialkyl sulfide catalysts for regio-, diastereo-, and enantioselective bromolactonization, Chem.–Eur. J., 2018, 24, 16747 CrossRef CAS PubMed;
(a
g) Y. Nishikawa, Y. Hamamoto, R. Satoh, N. Akada, S. Kajita, M. Nomoto, M. Miyata, M. Nakamura, C. Matsubara and O. Hara, Enantioselective bromolactonization of trisubstituted olefinic acids catalyzed by chiral pyridyl phosphoramides, Chem.–Eur. J., 2018, 24, 18880 CrossRef CAS PubMed;
(a
h) W. Wang, H. He, M. Gan, H. Wang, Y. Wang and X. Jiang, Enantioselective syntheses of α-exo-methylene-lactones via organocatalytic halolactonization, Adv. Synth. Catal., 2019, 361, 4797 CrossRef CAS;
(a
i) Q. Cao, J. Luo and X. Zhao, Chiral sulfide catalysis for desymmetrizing enantioselective chlorination, Angew. Chem., Int. Ed., 2019, 58, 1315 CrossRef CAS PubMed.
- For leading reviews on intermolecular asymmetric halogenation of olefins, see:
(a) J. Chen and L. Zhou, Recent progress in the asymmetric intermolecular halogenation of alkenes, Synthesis, 2014, 586 CAS;
(b) M. L. Landry and N. Z. Burns, Catalytic enantioselective dihalogenation in total synthesis, Acc. Chem. Res., 2018, 51, 1260 CrossRef CAS PubMed;
(c) Y. Cai, X. Liu, P. Zhou and X. Feng, Asymmetric catalytic halofunctionalization of α,β-unsaturated carbonyl compounds, J. Org. Chem., 2019, 84, 1 CrossRef CAS PubMed.
- For leading references on intermolecular asymmetric halogenation of olefins, see:
(a) Y. Cai, X. Liu, Y. Hui, J. Jiang, W. Wang, W. Chen, L. Lin and X. Feng, Catalytic asymmetric bromoamination of chalcones: highly efficient synthesis of chiral α-Bromo-β-Amino ketone derivatives, Angew. Chem., Int. Ed., 2010, 49, 6160 CrossRef CAS PubMed;
(b) Y. Cai, X. Liu, J. Jiang, W. Chen, L. Lin and X. Feng, Catalytic asymmetric chloroamination reaction of α,β-unsaturated γ-keto esters and chalcones, J. Am. Chem. Soc., 2011, 133, 5636 CrossRef CAS PubMed;
(c) Y. Cai, X. Liu, J. Li, W. Chen, W. Wang, L. Lin and X. Feng, Asymmetric iodoamination of chalcones and 4-aryl-4-oxobutenoates catalyzed by a complex based on scandium(III) and a N,N’-dioxide ligand, Chem.–Eur. J., 2011, 17, 14916 CrossRef CAS PubMed;
(d) K. C. Nicolaou, N. L. Simmons, Y. Ying, P. M. Heretsch and J. S. Chen, Enantioselective dichlorination of allylic alcohols, J. Am. Chem. Soc., 2011, 133, 813 Search PubMed;
(e) G.-X. Li, Q.-Q. Fu, X.-M. Zhang, J. Jiang and Z. Tang, First asymmetric intermolecular bromoesterification catalyzed by chiral Brønsted acid, Tetrahedron: Asymmetry, 2012, 23, 245 CrossRef CAS;
(f) A. Alix, C. Lalli, P. Retailleau and G. Masson, Highly enantioselective electrophilic α-bromination of enecarbamates: chiral phosphoric acid and calcium phosphate salt catalysts, J. Am. Chem. Soc., 2012, 134, 10389 CrossRef CAS PubMed;
(g) W. Zhang, N. Liu, C. M. Schienebeck, X. Zhou, I. I. Izhar, I. A. Guzei and W. Tang, Enantioselective intermolecular bromoesterification of allylic sulfonamides, Chem. Sci., 2013, 4, 2652 RSC;
(h) Y. Cai, X. Liu, P. Zhou, Y. Kuang, L. Lin and X. Feng, Iron-catalyzed asymmetric haloamination reactions, Chem. Commun., 2013, 49, 8054 RSC;
(i) D. X. Hu, G. M. Shibuya and N. Z. Burns, Catalytic enantioselective dibromination of allylic alcohols, J. Am. Chem. Soc., 2013, 135, 12960 CrossRef CAS PubMed;
(j) J. Qi, G.-T. Fan, J. Chen, M.-H. Sun, Y.-T. Dong and L. Zhou, Catalytic enantioselective bromoamination of allylic alcohols, Chem. Commun., 2014, 50, 13841 RSC;
(k) D. X. Hu, F. J. Seidl, C. Bucher and N. Z. Burns, Catalytic chemo-, regio-, and enantioselective bromochlorination of allylic alcohols, J. Am. Chem. Soc., 2015, 137, 3795 CrossRef CAS PubMed;
(l) M. L. Landry, D. X. Hu, G. M. Mckenna and N. Z. Burns, Catalytic enantioselective dihalogenation and the selective synthesis of (-)-deschloromytilipin A and (-)-danicalipin A, J. Am. Chem. Soc., 2016, 138, 5150 CrossRef CAS PubMed;
(m) W.-S. Huang, L. Chen, Z.-J. Zheng, K.-F. Yang, Z. Xu, Y.-M. Cui and L.-W. Xu, Catalytic asymmetric bromochlorination of aromatic allylic alcohols promoted by multifunctional Schiff base ligands, Org. Biomol. Chem., 2016, 14, 7927 RSC;
(n) C. Lebée, F. Blanchard and G. Masson, Highly enantioselective intermolecular iodo- and chloroamination of enecarbamates catalyzed by chiral phosphoric acids or calcium phosphate salts, Synlett, 2016, 27, 559 Search PubMed;
(o) S. Guo, F. Cong, R. Guo, L. wang and P. Tang, Asymmetric silver-catalysed intermolecular bromotrifluoromethoxylation of alkenes with a new trifluoromethoxylation reagent, Nat. Chem., 2017, 9, 546 CrossRef CAS PubMed;
(p) B. Soltanzadeh, A. Jaganathan, Y. Yi, H. Yi, R. J. Staples and B. Borhan, Highly regio- and enantioselective vicinal dihalogenation of allyl amides, J. Am. Chem. Soc., 2017, 139, 2132 CrossRef CAS PubMed;
(q) P. Zhou, L. Lin, L. Chen, X. Zhong, X. Liu and X. Feng, Iron-catalyzed asymmetric haloazidation of α,β-unsaturated ketones: construction of organic azides with two vicinal stereocenters, J. Am. Chem. Soc., 2017, 139, 13414 CrossRef CAS PubMed;
(r) A. J. Burckle, B. Gàl, F. J. Seidl, V. H. Vasilev and N. Z. Burns, Enantiospecific solvolytic functionalization of bromochlorides, J. Am. Chem. Soc., 2017, 139, 13562 CrossRef CAS PubMed;
(s) F. J. Seidl, C. Min, J. A. Lopez and N. Z. Burns, Catalytic regio- and enantioselective haloazidation of allylic alcohols, J. Am. Chem. Soc., 2018, 140, 15646 CrossRef CAS PubMed;
(t) N. Li, H. Yu, R. Wang, J. Shen, W.-Q. Wu, K. Liu, T.-T. Sun, Z.-Z. Zhang, C.-Z. Yao and J. Yu, Enantioselective intermolecular iodoacetalization of enol ethers catalyzed by chiral Co(III)-complex-templated Brønsted acids, Tetrahedron Lett., 2018, 59, 3605 CrossRef CAS;
(u) V. Wedek, R. V. Lommel, C. G. Daniliuc, F. D. Proft and U. Hennecke, Organocatalytic, enantioselective dichlorination of unfunctionalized alkenes, Angew. Chem., Int. Ed., 2019, 58, 9239 CrossRef CAS PubMed;
(v) T. Arai, K. Horigane, T. K. Suzuki, R. Itoh and M. Yamanaka, Catalytic asymmetric iodoesterification of simple alkenes, Angew. Chem., Int. Ed., 2020, 59, 12680 CrossRef CAS PubMed;
(w) D. C. Steigerwald, B. Soltanzadeh, A. Sarkar, C. C. Morgenstern, R. J. Staples and B. Borhan, Ritter-enabled catalytic asymmetric chloroamidation of olefins, Chem. Sci., 2021, 12, 1834 RSC.
- For oxyfluorination of enamides, see: T. Honjo, R. J. Phipps, V. Rauniyar and F. D. Toste, A doubly axially chiral phosphoric acid catalyst for the asymmetric tandem oxyfluorination of enamides, Angew. Chem., Int. Ed., 2012, 51, 9684 CrossRef CAS PubMed.
- For halohydroxylation, see:
(a) B. Soltanzadeh, A. Jaganathan, R. J. Staples and B. Borhan, Highly stereoselective intermolecular haloetherification and haloesterification of allyl amides, Angew. Chem., Int. Ed., 2015, 54, 9517 CrossRef CAS PubMed;
(b) Y.-M. Cao, D. Lentz and M. Christmann, Synthesis of enantioenriched bromohydrins via divergent reactions of racemic intermediates from anchimeric oxygen borrowing, J. Am. Chem. Soc., 2018, 140, 10677 CrossRef CAS PubMed;
(c) W. Li, P. Zhou, G. Li, L. Lin and X. Feng, Catalytic asymmetric halohydroxylation of α,β-unsaturated ketones with water as the nucleophile, Adv. Synth. Catal., 2020, 362, 1982 CrossRef CAS.
-
(a) D. Huang, H. Wang, F. Xue, H. Guan, L. Li, X. Peng and Y. Shi, Enantioselective bromocyclization of olefins catalyzed by chiral phosphoric acid, Org. Lett., 2011, 13, 6350 CrossRef CAS PubMed;
(b) D. Huang, X. Liu, L. Li, Y. Cai, W. Liu and Y. Shi, Enantioselective bromoaminocyclization of allyl N-tosylcarbamates catalyzed by a chiral phosphine-Sc(OTf)3 complex, J. Am. Chem. Soc., 2013, 135, 8101 CrossRef CAS PubMed;
(c) H. Huang, H. Pan, Y. Cai, M. Liu, H. Tian and Y. Shi, Enantioselective 6-endo bromoaminocyclization of 2,4-dienyl N-tosylcarbamates catalyzed by a chiral phosphine oxide-Sc(OTf)3 complex. A dramatic additive effect, Org. Biomol. Chem., 2015, 13, 3566 RSC;
(d) W. Liu, H. Pan, H. Tian and Y. Shi, Enantioselective 6-exo-bromoaminocyclization of homoallylic N-tosylcarbamates catalyzed by a novel monophosphine-Sc(OTf)3 complex, Org. Lett., 2015, 17, 3956 CrossRef CAS PubMed;
(e) Z. Li and Y. Shi, Chiral phosphine oxide-Sc(OTf)3 complex catalyzed enantioselective bromoaminocyclization of 2-benzofuranylmethyl N-tosylcarbamates. Approach to a novel class of optically active spiro compounds, Org. Lett., 2015, 17, 5752 CrossRef CAS PubMed;
(f) H. Pan, H. Huang, W. Liu, H. Tian and Y. Shi, Phosphine oxide-Sc(OTf)3 catalyzed highly regio- and enantioselective bromoaminocyclization of (E)-cinnamyl tosylcarbamates. An approach to a class of synthetically versatile functionalized molecules, Org. Lett., 2016, 18, 896 CrossRef CAS PubMed;
(g) X. Tan, H. Pan, H. Tian and Y. Shi, Phosphine oxide-Sc(OTf)3 catalyzed enantioselective bromoaminocyclization of tri-substituted allyl N-tosylcarbamates, Sci. China: Chem., 2018, 61, 656 CrossRef CAS.
- L. Li, C. Su, X. Liu, H. Tian and Y. Shi, Catalytic asymmetric intermolecular bromoesterification of unfunctionalized olefins, Org. Lett., 2014, 16, 3728 CrossRef CAS PubMed.
-
(a) X. Zhang, J. Li, H. Tian and Y. Shi, Catalytic asymmetric bromination of unfunctionalized olefins with H2O as a nucleophile, Chem.–Eur. J., 2015, 21, 11658 CrossRef CAS PubMed;
(b) J. Li, Z. Li, X. Zhang, B. Xu and Y. Shi, Catalytic enantioselective bromohydroxylation of aryl olefins with flexible functionalities, Org. Chem. Front., 2017, 4, 1084 RSC.
- Y. Gao, R. M. Hanson, J. M. Klunder, S. Y. Ko, Hi. Masamune and K.
B. Sharpless, Catalytic asymmetric epoxidation and kinetic resolution modified procedures including in situ derivatization, J. Am. Chem. Soc., 1987, 109, 5765 CrossRef CAS.
- The stereochemistry of 5–11 was tentatively assigned based on mechanistic considerations.
Footnote |
† Electronic supplementary information (ESI) available: Experimental, characteriza-tion data, X-ray structures of 2a, 2e, and 12, HPLC data for determination of enantiomeric excesses, and NMR spectra. CCDC 1941798, 1963482, and 2027431. For ESI and crystallographic data in CIF or other electronic format see DOI: 10.1039/d1ra02297k |
|
This journal is © The Royal Society of Chemistry 2021 |
Click here to see how this site uses Cookies. View our privacy policy here.