DOI:
10.1039/D1RA01912K
(Paper)
RSC Adv., 2021,
11, 11529-11535
Synthesis of zirconocene complexes and their use in slurry-phase polymerisation of ethylene†
Received
10th March 2021
, Accepted 11th March 2021
First published on 19th March 2021
Abstract
A new family of zirconocene complexes of the type (3-RInd#)2ZrX2 (where Ind# = C6Me5H and R = Me, Et and Ph) have been synthesised and fully characterised. Six new crystal structures have been reported (meso-(3-EtInd#)2ZrBr2, rac-(3-EtInd#)2ZrCl2, rac-(3-EtInd#)2Zr(CH2Ph)2, meso-(3-EtInd#)2Zr(CH2Ph)2, meso-(3-MeInd#)2ZrBr2 and meso-(3-MeInd#)2Zr(CH2Ph)2). The complexes were studied for slurry-phase ethylene polymerisation when immobilised on solid polymethylaluminoxane (sMAO). Variation in the initiation group was found to have greater influence over polymerisation activity for meso-catalysts than rac-catalysts, with meso-alkyl catalysts showing higher polymerisation activities than meso-halide. Below 70 °C, polymerisation activity follows the order sMAO-meso-(3-EtInd#)2Zr(CH2Ph)2, sMAO-meso-(3-EtInd#)2ZrCl2 and sMAO-meso-(3-EtInd#)2ZrBr2 (activities of 657, 561, and 452 kgPE molM−1 h−1 bar−1, respectively). sMAO-meso-(3-EtInd#)2ZrBr2 produces HDPE with the highest molecular weight, followed by sMAO-meso-(3-EtInd#)2ZrCl2 and sMAO-meso-(3-EtInd#)2Zr(CH2Ph)2 (Mw of 503, 406, and 345 kg mol−1, respectively, at 50 °C). sMAO-meso-(3-MeInd#)2ZrBr2 produced HDPE with almost identical molecular weights to sMAO-meso-(3-EtInd#)2ZrCl2 (395 kg mol−1 at 50 °C).
Introduction
Group 4 metallocenes are generally defined as d0, pseudo-tetrahedral organometallic compounds in which the transition metal atom bears two η5-cyclopentadienyl-based ligands and two σ-ligands.1 Upon the activation of group 4 metallocenes by co-catalysts (e.g. MAO),2 the resulting highly electrophilic alkyl metallocene cations, stabilised by non-nucleophilic, very weakly coordinating anions, are known to be the active species for α-olefin polymerisation.3–5 The stability of the cationic intermediate and the electron density, accessibility and geometry of the active site, all of which are influenced by ligand structure, are believed to be the main influencing factors towards reactivity and stereospecificity.6
The discovery of ‘single-site’ metallocene-based catalysts has revived α-olefin polymerisation chemistry as these catalyst systems enable the production of (co)polyolefins with tuneable molecular structures, stereochemistries and molecular weight distributions (MWD, Mw/Mn).7–14 Self-immobilisation of catalyst and co-catalysts for olefin polymerisation has shown that the high excess of MAO can reduced by more than 90%.15 Yet these catalysts are rarely used for industrial processes, with a few exceptions including Dowlex (Dow) and Sclairtech (Nova Chem. Corp.); due to the incompatibility with the existing gas or slurry phase olefin polymerisation processes. By immobilising metallocene catalysts onto support materials, the processability problem is solved and the advantages of metallocene catalysts, including narrow MWD, high activity, and precise control over polymer microstructure, are preserved to a great extent. Supporting metallocene complexes also allows for the reduction of the ratio of MAO to metallocene,16 and have an dramatic effect on the tacticity when polymerising α-olefins.8 Many materials were tested as olefin polymerisation supports including inorganic solids such as silica,17 silicate clays,18 MgCl2,19 and polymeric aluminoxanes.20 Various functionalised polymers have also been investigated as supports. Silica is the most commonly used support for heterogeneous metallocene catalysts. Precontacting the silica surface with MAO was reported to increase catalyst loadings.21–24 The use of layered double hydroxides (LDHs), a class of anionic clays consisting of positively charged Brucite-like layers with weakly bound anions intercalated between them, as catalyst supports for olefin polymerisation has been recently reported by the O'Hare group.25–27 Heat treatment of MAO for a prolonged period was reported to increase polymerisation activity of up to 25%. This is believed to be caused by the aggregation of MAO to form larger particles which are insoluble in hydrocarbon solvents. This insoluble ‘solid MAO’ can act simultaneously as both co-catalyst and support in slurry phase ethylene polymerisation.28,29
Results and discussion
Synthesis of (3-RInd#)2ZrX2 (R = Me, Et or Ph; X= Cl, Br or CH2Ph)
3-PhInd#Li was synthesised according to a modified literature procedure (Fig. S1–S10†).30 Two equivalents 3-PhInd#Li were reacted with one equivalent of ZrCl4 to afford a 50
:
50 mixture of rac- and meso-(3-PhInd#)2ZrCl2 (1) as an orange yellow solid in 45% yield (Scheme 1). The two sets of resonances corresponding to each isomer are indistinguishable in the 1H NMR spectrum; resonances between 6.90 and 7.90 ppm correspond to the phenyl protons, while two singlets at 5.86 and 6.52 ppm correspond to the cyclopentadienyl protons and singlets between 1.60 and 2.80 ppm correspond to the methyl groups on the indenyl rings (Fig. S11†).
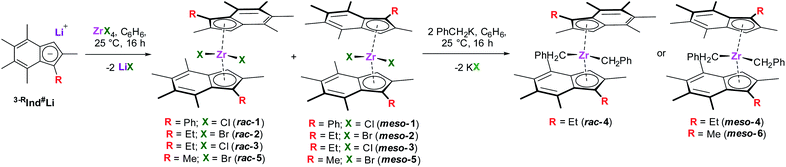 |
| Scheme 1 Synthesis of rac-(3-PhInd#)2ZrCl2 (rac-1), meso-(3-PhInd#)2ZrCl2 (meso-1), rac-(3-EtInd#)2ZrBr2 (rac-2), meso-(3-EtInd#)2ZrBr2 (meso-2), rac-(3-EtInd#)2ZrCl2 (rac-3), meso-(3-EtInd#)2ZrCl2 (meso-3), rac-(3-EtInd#)2Zr(CH2Ph)2 (rac-4), meso-(3-EtInd#)2Zr(CH2Ph)2 (meso-4), rac-(3-MeInd#)2ZrBr2 (rac-5), meso-(3-MeInd#)2ZrBr2 (meso-5), and meso-(3-MeInd#)2Zr(CH2Ph)2 (meso-6). | |
3-EtInd#Li was synthesised according to a previously reported procedure.30 Two equivalents of 3-EtInd#Li were reacted with one equivalent ZrBr4 to afford an orange solid comprising of a 40
:
60 mixture of rac- and meso-(3-EtInd#)2ZrBr2 after work-up. Recrystallisation of the isomeric mixture yielded meso-(3-EtInd#)2ZrBr2 (meso-2) as orange crystals in 6% yield. Similar to 1, meso-2 shows diagnostic resonances corresponding to the cyclopentadienyl proton and methyl groups of the indenyl rings. meso-2 also shows two doublet of quartets at 2.69 and 3.22 ppm and a triplet at 1.05 ppm corresponding to the diastereotopic methylene and methyl protons of the ethyl groups respectively (Fig. S13†).
rac-(3-EtInd#)2ZrCl2 (rac-3) and meso-(3-EtInd#)2ZrCl2 (meso-3) were prepared according to a literature procedure.30 They were reacted with two equivalents KCH2Ph to afford rac-(3-EtInd#)2Zr(CH2Ph)2 (rac-4) and meso-(3-EtInd#)2Zr(CH2Ph)2 (meso-4) as a yellow solids, both in 93% yield after work-up. The C2-symmetry of rac-4 results in magnetically equivalent indenyl rings and benzyl groups, as evidenced in the 1H NMR spectrum; the phenyl protons are seen as a doublet, doublet of doublets, and triplet at 6.62, 7.08, and 6.80 ppm, respectively, while two doublets at 0.67 and 0.87 ppm correspond to the diastereotopic benzylic protons (Fig. S15†). Similar to meso-Me2SB(3-EtI*)Zr(CH2Ph)2,31 the symmetry of meso-4 results in magnetically inequivalent benzyl groups in the 1H NMR spectrum; the ortho, meta, para, and benzylic protons of one benzyl group are observed as a doublet at 6.46 ppm, a doublet of doublets at 7.04 ppm, a triplet at 6.75 ppm, and a singlet at 0.24 ppm, while for the other benzyl group they appear at 6.58, 7.06, 6.79, and 0.79 ppm (Fig. S17†). 3-MeInd#Li was synthesised according to a previously reported procedure.32 Two equivalents 3-MeInd#Li were reacted with one equivalent ZrBr4 to afford an orange solid comprising of a 20
:
80 mixture of rac- and meso-(3-MeInd#)2ZrBr2, and impurities. Recrystallisation of the isomeric mixture in toluene at −30 °C yielded meso-(3-MeInd#)2ZrBr2 (meso-5) as orange crystals in 6% yield. The 1H NMR spectrum of meso-5 shows that the two indenyl rings are magnetically equivalent, with diagnostic resonances corresponding to the cyclopentadienyl protons and indenyl methyl groups (Fig. S19†). One equivalent meso-5 was further reacted with two equivalents of KCH2Ph to afford meso-(3-MeInd#)2Zr(CH2Ph)2 (meso-6) as a yellow solid in 88% yield. Similar to meso-4, the Cs-symmetry of meso-6 results in magnetically inequivalent benzyl groups in the 1H NMR spectrum (Fig. S21†).
Orange crystals of meso-2, rac-4, and meso-4 were grown from pentane at room temperature, while crystals of rac-3, meso-5 and meso-6 were grown from a toluene solution at −30 °C and room temperature. The solid-state molecular structures are depicted in Fig. 1, with selected bond lengths and angles presented in Table 1.
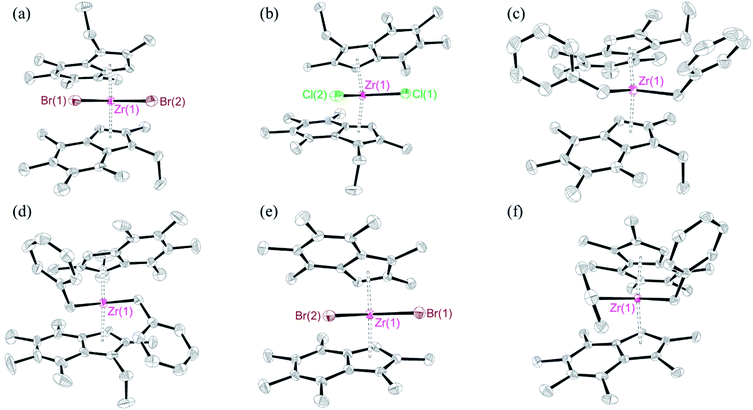 |
| Fig. 1 Molecular structure of (a) meso-(3-EtInd#)2ZrBr2 (meso-2), (b) rac-(3-EtInd#)2ZrCl2 (rac-3), (c) meso-(3-EtInd#)2Zr(CH2Ph)2 (meso-4), (d) rac-(3-EtInd#)2Zr(CH2Ph)2 (rac-4), (e) meso-(3-MeInd#)2ZrBr2 (meso-5), and (f) meso-(3-MeInd#)2Zr(CH2Ph)2 (meso-6). Hydrogen atoms omitted for clarity, ellipsoids drawn at 50% probability. | |
Table 1 Selected bond lengths (Å) and angles (°) for meso-(3-EtInd#)2ZrBr2 (meso-2), rac-(3-EtInd#)2ZrCl2 (rac-3), (meso-(3-EtInd#)2Zr(CH2Ph)2 (meso-4), rac-(3-EtInd#)2Zr(CH2Ph)2 (rac-4), meso-(3-MeInd#)2ZrBr2 (meso-5), and meso-(3-MeInd#)2Zr(CH2Ph)2 (meso-6)a
|
Complex |
Zr-X |
Zr-Cpcent |
α |
δ |
TA |
X = Br, Cl or CH2Ph; α, δ and TA: crystallographic parameters are defining in Fig. S23. |
meso-2 |
meso-(3-EtInd#)2ZrBr2 |
2.6041(5) |
2.2425(11) |
52.44(14) |
131.93(4) |
81.49 |
2.5927(5) |
2.2430(11) |
rac-3 |
rac-(3-EtInd#)2ZrCl2 |
2.4183(6) |
2.2453(8) |
51.72(10) |
132.44(3) |
173.14 |
2.4514(6) |
2.2374(8) |
meso-4 |
meso-(3-EtInd#)2Zr(CH2Ph)2 |
2.307(2) |
2.3045(8) |
51.80(11) |
132.99(3) |
102.95 |
2.3284(19) |
2.2666(9) |
rac-4 |
rac-(3-EtInd#)2Zr(CH2Ph)2 |
2.307(7) |
2.322(3) |
51.9(4) |
133.63(11) |
157.30 |
2.299(7) |
2.281(3) |
meso-5 |
meso-(3-MeInd#)2ZrBr2 |
2.6133(4) |
2.2500(10) |
54.35(13) |
133.62(4) |
35.46 |
2.5745(4) |
2.2525(10) |
meso-6 |
meso-(3-MeInd#)2Zr(CH2Ph)2 |
2.3302(18) |
2.2763(9) |
53.66(10) |
133.92(3) |
112.56 |
2.3132(18) |
2.3419(10) |
For meso-2, the average Zr–Br bond length (2.598 Å) and Zr–Cpcent distance (2.243 Å) are within the range of those reported for other unbridged dibromobisindenyl zirconocene complexes.16–18 The ring tilt angle (α) (52.44°) and angle about the metal centre (δ) (131.93) are similar to meso-(3-MeInd#)2ZrCl2 (53.28° and 132.02°, respectively).32 The torsion angle (TA) measures the twist of the two indenyl rings relative to one another (Fig. S23†). The introduction of ethyl groups causes increased steric strain, resulting in meso-2 adopting ‘gauche’ conformation, which is reflected in the TA angle of 81.49° compared to 37.16° for meso-(3-MeInd#)2ZrCl2.32
The average Zr–Cl bond length and Zr–Cpcent distance of rac-3 (2.435 and 2.241 Å) are in good agreement with the 3-methyl analogue rac-(3-MeInd#)2ZrCl2 (2.421 and 2.228 Å).32 The α and δ values (Fig. S23†) of rac-3 (51.72° and 132.44°) are comparable to rac-(3-MeInd#)2ZrCl2 (51.58° and 133.59°) and rac-(3-EtInd#)2HfCl2 (51.80° and 132.56°), as are the torsion angles (173.14, 171.31, and 173.01°, respectively), implying that the introduction of 3-ethyl groups has little-to-no impact on the molecular frameworks. Structure comparisons of rac-3 with the unmethylated analog, rac-(1-EtInd)2ZrCl2, show similar average Zr–Cl bond lengths (2.435 and 2.442 Å) and Zr–Cpcent distances (2.241 and 2.233 Å).33 The α and δ values for rac-3 (51.72° and 132.44°) are slightly larger than for rac-(1-EtInd)2ZrCl2 (49.22° and 131.98°). However, the TA value for rac-3 (173.14°) is significantly larger than for rac-(1-EtInd)2ZrCl2 (120.02°) due to the increased sterics of methylation. For meso-4, the average Zr–CH2Ph bond length (2.318 Å) is similar to rac-4 (2.303 Å) and the hexamethylated analogue rac-(3-MeInd#)2Zr(CH2Ph)2 (2.320 Å),32 and is slightly longer than (4,7-FInd)2Zr(CH2Ph)2 (2.294 Å).34 Similar to meso-2, meso-4 has a TA value of 102.95°, adopting ‘gauche’ conformation to minimise the steric clash between the ethyl and benzyl groups. The average Zr–Br bond length and Zr–Cpcent distances of meso-5 (2.594 and 2.251 Å) are similar to meso-2 (2.598 and 2.243 Å). The α value for meso-5 (54.35°) is slightly larger than meso-(3-MeInd#)2ZrCl2 (53.28°) due to the larger Br ligands.32 The TA value of 35.46° for meso-5 is comparable to meso-(3-MeInd#)2ZrCl2 (37.16°),32 however, is significantly less than meso-2 (81.49°) due to the decrease in steric strain on exchanging ethyl for methyl. For meso-6, the average Zr–CH2Ph bond length (2.322 Å) is comparable to rac-4 (2.302 Å) and rac-(3-MeInd#)2Zr(CH2Ph) (2.320 Å).32 However, comparisons between the α values of meso-6 and rac-4 are challenging because the complexes adopt different conformations, reflected in the TA values of 112.56° and 157.30°, in order to minimise the steric interactions between the indenyl and benzyl ligands.
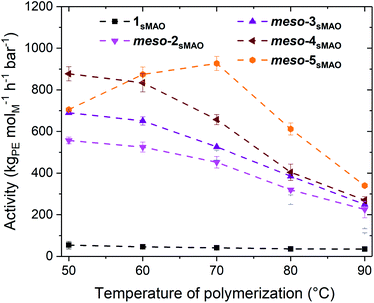 |
| Fig. 2 Polymerisation activity as a function of temperature of polymerisation using sMAO supported 50 : 50 rac- and meso-(3-PhInd#)2ZrCl2 (1sMAO; black square), meso-(3-EtInd#)2ZrBr2 (meso-2sMAO; pink down triangle), meso-(3-EtInd#)2ZrCl2 (meso-3sMAO; purple triangle), meso-(3-EtInd#)2Zr(CH2Ph)2 (meso-4sMAO; brown right triangle), and meso-(3-MeInd#)2ZrBr2 (meso-5sMAO; orange hexagon). Polymerisation conditions: ethylene (2 bar), pre-catalyst (10 mg), hexane (50 mL), [AlsMAO]0/[Zr]0 = 200, TiBA (1000 eq.), and 30 minutes. | |
Slurry-phase ethylene polymerisation
A 50
:
50 mixture of rac- and meso-(3-PhInd#)ZrCl2 (1), meso-(3-EtInd#)2ZrBr2 (meso-2), rac-(3-EtInd#)2ZrCl2 (rac-3), meso-(3-EtInd#)2ZrCl2 (meso-3), rac-(3-EtInd#)2Zr(CH2Ph)2 (rac-4), meso-(3-EtInd#)2Zr(CH2Ph)2 (meso-4) meso-(3-MeInd#)2ZrBr2 (meso-5sMAO) were immobilised on solid polymethylaluminoxane (sMAO)28,29,35 according to a literature procedure with an initial aluminium to zirconium ([AlsMAO]0/[Zr]0) loading of 200.30 Slurry-phase ethylene polymerisations were conducted in 150 mL ampoules with 50 mL hexane, 10 mg pre-catalyst and 2 bar ethylene at 50–90 °C for 30 minutes with triisobutylaluminium (TiBA, Al(CH2CH(CH3)2)3) scavenger with [Alscav]0/[M]0 of 1000 (Fig. 2).
1sMAO shows significantly lower polymerisation activities than rac-3sMAO and meso-3sMAO, likely due to the large steric bulk of the phenyl groups. rac-3sMAO displays higher polymerisation activities than meso-3sMAO below 70 °C, and almost identical polymerisation activities above 70 °C, while rac-4sMAO and meso-4sMAO display similar polymerisation activities across the entire temperature range. rac-3 shows significantly higher polymerisation activities when immobilised on sMAO compared to MAO modified silica (activities of 561 and approximately 75 kgPE molM−1 h−1 bar−1 respectively at 70 °C).30 rac-3sMAO and rac-4sMAO show lower polymerisation activities than the 3-methyl analogues (under similar polymerisation conditions with [AlsMAO]0/[Zr]0 = 300); activities of 858 and 870 kgPE molM−1 h−1 bar−1 at 60 °C for rac-3sMAO and sMAO-rac-(3-MeInd#)2ZrCl2 respectively, and activities of 864 and 1063 kgPE molM−1 h−1 bar−1 at 60 °C for rac-4sMAO and sMAO-rac-(3-MeInd#)2Zr(CH2Ph)2 respectively.35 However, meso-3sMAO shows polymerisation activities approximately double sMAO-meso-(3-MeInd#)2ZrCl2 (651 and 343 kgPE molM−1 h−1 bar−1 respectively at 60 °C).35
Looking at the meso-catalysts, below 70 °C polymerisation activity follows the order meso-4sMAO, meso-3sMAO, and meso-2sMAO (activities of 657, 561, and 452 kgPE molM−1 h−1 bar−1, respectively). This is likely due to faster formation of the active species, as catalysts containing halide initiation groups first need to be alkylated.36,37 meso-4sMAO shows less resilience to higher polymerisation temperatures and, as a result, the polymerisation activities converge to approximately 250 kgPE molM−1 h−1 bar−1 at 90 °C.
Reducing the steric bulk in the indenyl ligand leads to increases in polymerisation activities; the 3-methyl catalyst meso-5sMAO shows higher polymerisation activities than the corresponding 3-ethyl catalyst meso-2sMAO (maximum activities of 927 kgPE molM−1 h−1 bar−1 at 70 °C and 503 kgPE molM−1 h−1 bar−1 at 50 °C, respectively). Unlike the other catalysts, which show continual decreases in activity with increasing temperature, meso-5sMAO shows a peak in activity at 70 °C (927 kgPE molM−1 h−1 bar−1) which could be related to the fact that meso-5sMAO possesses the largest gap aperture (α = 54.35(13)°).38,39 meso-5sMAO displays lower polymerisation activity than the ethylene bridged analogue sMAO-meso-(EBI*)ZrCl2; activities of 874 and 1331 kgPE molM−1 h−1 bar−1 respectively at 60 °C (under similar polymerisation conditions with [Al]0/[Zr]0 = 300),35 and lower than solution phase of well-known metallocene complexes.40
The molecular weights (Mw) and molecular weights distribution (Mw/Mn) of the polyethylenes produced were analysed by gel permeation chromatography (GPC) (Fig. 3). rac-3sMAO and rac-4sMAO produce polymers with similar molecular weights; 391 and 381 kg mol−1 at 50 °C, which is expected due to their similar polymerisation activities. rac-3sMAO produces polymers with lower molecular weights than the dimethyl silyl bridged analog, Mw of 447 mol−1 at 50 °C for sMAO-rac-Me2SB(3-EtI*)ZrCl2. rac-3-HfsMAO produced polymers with the lowest molecular weights (297 kg mol−1 at 50 °C). For the meso-3-ethyl catalysts, meso-2sMAO produced polymers with the highest molecular weights, followed by meso-3sMAO and meso-4sMAO (Mw of 503, 406, and 345 kg mol−1, respectively, at 50 °C). meso-5sMAO produced polymers with almost identical molecular weights to meso-3sMAO (395 kg mol−1 at 50 °C). All polymers show the expected decreases in polymer molecular weights with increasing polymerisation temperature, attributed to stronger chain transfer reactions at elevated temperatures.41 No long chain branching was observed by GPC or NMR spectroscopy,42,43 but very large molecular weights distribution were observed (Mw/Mn around 5–8, Tables S4–S9†) which will point towards several catalytic species on the surface.44
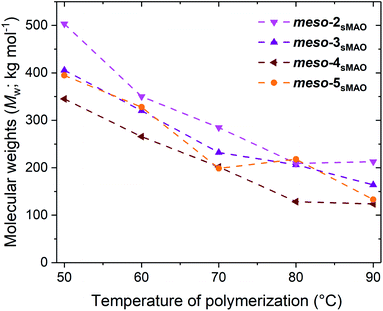 |
| Fig. 3 Molecular weights (Mw) as a function of temperature of polymerisation using sMAO supported meso-(3-EtInd#)2ZrBr2 (meso-2sMAO; pink down triangle), meso-(3-EtInd#)2ZrCl2 (meso-3sMAO; purple triangle), meso-(3-EtInd#)2Zr(CH2Ph)2 (meso-4sMAO; brown right triangle), and meso-(3-MeInd#)2ZrBr2 (meso-5sMAO; orange hexagon). Polymerisation conditions: ethylene (2 bar), pre-catalyst (10 mg), hexane (50 mL), [AlsMAO]0/[M]0 = 200, TiBA (1000 eq.), and 30 minutes. | |
Conclusions
A new family of unbridged bis(peralkylindenyl) zirconocene complexes of the type (3-RInd#)2ZrX2 have been synthesised and fully characterised. Six new crystal structures have been reported.
When immobilised on solid polymethylaluminoxane (sMAO) these complexes produce HDPE in slurry-phase ethylene polymerisations. The supported meso-alkyl catalysts showing higher polymerisation activities than meso-halide catalysts; polymerisation activity follows the order meso-4sMAO, meso-3sMAO, and meso-2sMAO.
These metallocene complexes and their inorganic based supported catalysts show promising results for use as slurry-phase polymerisation catalysts. Further testing with hydrogen, co-monomers and α-olefins will be carried out.
Experimental section
General details, synthesis and characterisation of ligands precursors, NMR spectra, X-ray crystallography data and further polymerisation data are included in the ESI.†
Synthesis of (3-PhInd#)2ZrCl2 (1)
2.0 equivalents 3-PhInd#Li (2.00 g, 7.45 mmol) and 1.0 equivalent ZrCl4 (0.868 g, 3.73 mmol) were stirred in benzene (100 mL) for 16 hours at room temperature. The reaction mixture was allowed to settle, filtered and the resulting orange filtrate dried in vacuo to afford a 50
:
50 mixture of rac-(3-PhInd#)2ZrCl2 (rac-1) and meso-(3-PhInd#)2ZrCl2 (meso-1) as an orange-yellow solid in 45% yield (1.15 g, 1.68 mmol). 1H NMR (chloroform-d1, 400 MHz, 298 K) δ (ppm): 7.75 (Ph-H, 2H, d, 3JHH = 7.5 Hz), 7.56 (Ph-H, 2H, d, 3JHH = 7.5 Hz), 7.28 (Ph-H, 4H, m), 7.18 (Ph-H, 6H, m), 7.14 (Ph-H, 2H, m), 6.91 (Ph-H, 2H, m), 6.88 (Ph-H, 2H, m), 6.42 (Ind-H, 2H, s), 5.76 (Ind-H, 2H, s), 2.63 (Ind-CH3, 6H, s), 2.19 (Ind-CH3, 6H, s), 2.17 (Ind-CH3, 12H, s), 2.11 (Ind-CH3, 12H, s), 2.08 (Ind-CH3, 6H, s), 1.92 (Ind-CH3, 6H, s), 1.88 (Ind-CH3, 6H, s), 1.54 (Ind-CH3, 6H, s). 13C{1H} NMR (chloroform-d1, 101 MHz, 298 K) δ (ppm): 136.1 (Ind-Ph), 136.0 (Ind-Ph), 134.9 (Ind), 134.8 (Ind), 134.0 (Ph-H), 133.7 (Ind), 133.6 (Ind), 132.5 (Ind), 132.4 (Ph-H), 132.2 (Ph-H), 132.1 (Ph-H), 131.8 (Ind), 130.7 (Ind), 130.5 (Ind), 130.5 (Ind), 127.9 (Ind), 127.7 (Ph-H), 127.6 (Ind), 127.6 (Ind), 127.3 (Ph-H), 127.3 (Ph-H), 127.1 (Ph-H), 127.0 (Ph-H), 127.0 (Ph-H), 125.8 (Ind), 125.4 (Ind), 125.1 (Ind), 124.4 (Ind), 98.6 (Ind-H), 94.4 (Ind-H), 18.8 (Ind-CH3), 18.5 (Ind-CH3), 18.3 (Ind-CH3), 17.1 (Ind-CH3), 16.9 (Ind-CH3), 16.8 (Ind-CH3), 16.7 (Ind-CH3), 16.7 (Ind-CH3), 16.6 (Ind-CH3), 15.1 (Ind-CH3). CHN analysis (%): calculated C 70.15, H 6.18; observed C 70.26, H 6.38. HRMS (ESI): expected m/z 682.1705; observed 682.1736 [M]+.
Synthesis of (3-EtInd#)2ZrBr2 (meso-2)
2.0 equivalents 3-EtInd#Li (2.00 g, 9.08 mmol) and 1.0 equivalent ZrBr4 (1.87 g, 4.54 mmol) were stirred in benzene (100 mL) for 16 hours at room temperature. The reaction mixture was allowed to settle, filtered and the resulting orange filtrate dried in vacuo to afford a 40
:
60 mixture of rac-(3-EtInd#)2ZrBr2 and meso-(3-EtInd#)2ZrBr2 as an orange solid. Recrystallisation of the isomeric mixture in DCM at −30 °C yielded meso-(3-EtInd#)2ZrBr2 (meso-2) as orange crystals in 6% yield (0.178 g, 0.263 mmol). Orange crystals of meso-(3-EtInd#)2ZrBr2 suitable for a single crystal X-ray diffraction study were grown in pentane at room temperature. meso-(3-EtInd#)2ZrBr2: 1H NMR (chloroform-d1, 400 MHz, 298 K) δ (ppm): 6.25 (Ind-H, 2H, s), 3.22 (Ind-CH2–CH3, 2H, dq, 2JHH = 15.0 Hz, 3JHH = 7.5 Hz), 2.69 (Ind-CH2–CH3, 2H, dq, 2JHH = 15.0 Hz, 3JHH = 7.5 Hz), 2.54 (Ind-CH3, 12H, s), 2.27 (Ind-CH3, 6H, s), 2.23 (Ind-CH3, 6H, s), 1.70 (Ind-CH3, 6H, s), 1.05 (Ind-CH2–CH3, 6H, t, 3JHH = 7.5 Hz). 13C{1H} NMR (chloroform-d1, 101 MHz, 298 K) δ (ppm): 134.2 (Ind), 133.4 (Ind), 130.9 (Ind), 130.8 (Ind), 127.8 (Ind), 126.0 (Ind), 125.6 (Ind), 125.2 (Ind), 94.6 (Ind-H), 23.0 (Ind-CH2–CH3), 17.6 (Ind-CH3), 17.1 (Ind-CH3), 16.8 (Ind-CH3), 16.6 (Ind-CH3), 15.6 (Ind-CH2–CH3), 14.6 (Ind-CH3). CHN analysis (%): calculated C 56.71, H 6.25; observed C 56.61, H 6.33. HRMS (ESI): expected m/z 674.0695; observed 674.0724 [M]+.
Synthesis of rac-(3-EtInd#)2Zr(CH2Ph)2 (rac-4)
1.0 equivalent rac-(3-EtInd#)2ZrCl2 (45.2 mg, 0.0768 mmol) and 2.0 equivalents KCH2Ph (20.0 mg, 0.154 mmol) were stirred in benzene (1 mL) for 16 hours at room temperature. The reaction mixture was allowed to settle, filtered and the resulting yellow filtrate dried in vacuo to afford rac-(3-EtInd#)2Zr(CH2Ph)2 (rac-4) as a yellow solid in 93% yield (50.2 mg, 0.0717 mmol). Yellow crystals of rac-(3-EtInd#)2Zr(CH2Ph)2 suitable for a single crystal X-ray diffraction study were grown in pentane at −30 °C. 1H NMR (chloroform-d1, 400 MHz, 298 K) δ (ppm): 7.08 (m-Ph-H, 4H, dd, 3JHH = 7.3, 7.4 Hz), 6.80 (p-Ph-H, 2H, t, 3JHH = 7.3 Hz), 6.62 (o-Ph-H, 4H, d, 3JHH = 7.4 Hz), 4.91 (Ind-H, 2H, s), 2.76 (Ind-CH2–CH3, 2H, dq, 2JHH = 14.8 Hz, 3JHH = 7.4 Hz), 2.51 (Ind-CH3, 6H, s), 2.45 (Ind-CH2–CH3, 2H, dq, 2JHH = 14.8 Hz, 3JHH = 7.4 Hz), 2.28 (Ind-CH3, 6H, s), 2.23 (Ind-CH3, 6H, s), 2.01 (Ind-CH3, 6H, s), 1.82 (Ind-CH3, 6H, s), 0.99 (Ind-CH2–CH3, 6H, t, 3JHH = 7.4 Hz), 0.87 (Zr-CH2-Ph, 2H, d, 2JHH = 11.2 Hz), 0.67 (Zr-CH2-Ph, 2H, d, 2JHH = 11.2 Hz). 13C{1H} NMR (chloroform-d1, 101 MHz, 298 K) δ (ppm): 152.8 (Zr–CH2-Ph), 133.3 (Ind), 131.0 (Ind), 128.3 (Ind), 127.9 (Ind), 127.8 (Ind), 127.6 (m-Ph), 127.1 (o-Ph), 126.4 (Ind), 123.2 (Ind), 121.0 (p-Ph), 119.8 (Ind), 100.8 (Ind-H), 66.0 (Zr-CH2-Ph), 21.6 (Ind-CH2–CH3), 17.1 (Ind-CH3), 16.8 (Ind-CH3), 16.7 (Ind-CH3), 16.6 (Ind-CH3), 16.0 (Ind-CH2–CH3), 13.1 (Ind-CH3). CHN analysis (%): calculated C 78.91, H 8.06; observed C 78.91, H 8.15.
Synthesis of meso-(3-EtInd#)2Zr(CH2Ph)2 (meso-4)
1.0 equivalent meso-(3-EtInd#)2ZrCl2 (45.2 mg, 0.0768 mmol) and 2.0 equivalents KCH2Ph (20.0 mg, 0.154 mmol) were stirred in benzene (1 mL) for 16 hours at room temperature. The reaction mixture was allowed to settle, filtered, and the resulting yellow filtrate dried in vacuo to afford meso-(3-EtInd#)2Zr(CH2Ph)2 (meso-4) as a yellow solid in 93% yield (49.9 mg, 0.0713 mmol). Orange crystals of meso-(3-EtInd#)2Zr(CH2Ph)2 suitable for a single crystal X-ray diffraction study were grown in pentane at −30 °C. 1H NMR (chloroform-d1, 400 MHz, 298 K) δ (ppm): 7.06 (m-Ph-H, 2H, dd, 3JHH = 7.3, 7.6 Hz), 7.04 (m-Ph-H, 2H, dd, 3JHH = 7.3, 7.6 Hz), 6.79 (p-Ph-H, 1H, t, 3JHH = 7.3 Hz), 6.75 (p-Ph-H, 1H, t, 3JHH = 7.3 Hz), 6.58 (o-Ph-H, 2H, d, 3JHH = 7.6 Hz), 6.46 (o-Ph-H, 2H, d, 3JHH = 7.6 Hz), 5.61 (Ind-H, 2H, s), 2.71 (Ind-CH2–CH3, 2H, dq, 2JHH = 14.8 Hz, 3JHH = 7.4 Hz), 2.45 (Ind-CH3, 6H, s), 2.38 (Ind-CH2–CH3, 2H, dq, 2JHH = 14.8 Hz, 3JHH = 7.4 Hz), 2.22 (Ind-CH3, 6H, s), 2.18 (Ind-CH3, 6H, s), 2.10 (Ind-CH3, 6H, s), 1.77 (Ind-CH3, 6H, s), 0.95 (Ind-CH2–CH3, 6H, t, 3JHH = 7.4 Hz), 0.79 (Zr–CH2-Ph, 2H, s), 0.24 (Zr–CH2-Ph, 2H, s). 13C{1H} NMR (chloroform-d1, 101 MHz, 298 K) δ (ppm): 153.8 (Zr–CH2-Ph), 152.7 (Zr–CH2-Ph), 133.1 (Ind), 131.8 (Ind), 127.6 (m-Ph), 127.5 (Ind), 127.5 (m-Ph), 127.3 (Ind), 127.2 (o-Ph), 126.9 (Ind), 126.6 (o-Ph), 126.5 (Ind), 123.3 (Ind), 121.1 (p-Ph), 120.7 (p-Ph), 118.9 (Ind), 100.7 (Ind-H), 67.5 (Zr-CH2-Ph), 66.3 (Zr-CH2-Ph), 22.0 (Ind-CH2–CH3), 17.1 (Ind-CH3), 16.8 (Ind-CH3), 16.7 (Ind-CH3), 16.6 (Ind-CH3), 16.2 (Ind-CH2–CH3), 13.1 (Ind-CH3). CHN analysis (%): calculated C 78.91, H 8.06; observed C 78.79, H 8.16.
Synthesis of meso-(3-MeInd#)2ZrBr2 (meso-5)
2.0 equivalents Ind#Li (2.00 g, 9.70 mmol) and 1.0 equivalent ZrBr4 (1.99 g, 4.85 mmol) were stirred in benzene (100 mL) for 16 hours at room temperature. The reaction mixture was allowed to settle, filtered and the resulting red filtrate dried in vacuo to afford an orange solid comprising of a 20
:
80 mixture of rac-(3-MeInd#)2ZrBr2 and meso-(3-MeInd#)2ZrBr2 and impurities. Recrystallisation of the isomeric mixture in toluene at −30 °C yielded meso-(3-MeInd#)2ZrBr2 (meso-5) as orange crystals, suitable for a single crystal X-ray diffraction study, in 6% yield (0.180 g, 0.277 mmol). 1H NMR (chloroform-d1, 400 MHz, 298 K) δ (ppm): 5.90 (Ind-H, 2H, s), 2.64 (Ind-CH3, 6H, s), 2.55 (Ind-CH3, 6H, s), 2.22 (Ind-CH3, 6H, s), 2.20 (Ind-CH3, 6H, s), 2.17 (Ind-CH3, 6H, s), 2.12 (Ind-CH3, 6H, s). 13C{1H} NMR (chloroform-d1, 101 MHz, 298 K) δ (ppm): 134.0 (Ind), 133.4 (Ind), 131.0 (Ind), 129.6 (Ind), 127.4 (Ind), 127.4 (Ind), 126.8 (Ind), 121.2 (Ind), 96.7 (Ind-H), 17.5 (Ind-CH3), 16.7 (Ind-CH3), 16.6 (Ind-CH3), 16.6 (Ind-CH3), 16.6 (Ind-CH3), 16.2 (Ind-CH3). CHN analysis (%): calculated C 55.46, H 5.90; observed C 55.50, H 6.02. HRMS (EI): expected m/z 646.0382; observed 646.0366 [M]+.
Synthesis of meso-(3-MeInd#)2Zr(CH2Ph)2 (meso-6)
1.0 equivalent meso-(Ind#)2ZrBr2 (49.9 mg, 0.0768 mmol) and 2.0 equivalents KCH2Ph (20.0 mg, 0.154 mmol) were stirred in benzene (1 mL) for 16 hours at room temperature. The reaction mixture was allowed to settle, filtered and the resulting yellow filtrate dried in vacuo to afford meso-(3-MeInd#)2Zr(CH2Ph)2 (meso-6) as a yellow solid in 88% yield (45.6 mg, 0.0678 mmol). Yellow crystals of meso-(3-MeInd#)2Zr(CH2Ph)2 suitable for a single crystal X-ray diffraction study were grown in toluene at room temperature. 1H NMR (chloroform-d1, 500 MHz, 298 K) δ (ppm): 7.09 (m-Ph-H, 2H, dd, 3JHH = 7.3, 7.6 Hz), 7.04 (m-Ph-H, 2H, dd, 3JHH = 7.3, 7.6 Hz), 6.82 (p-Ph-H, 1H, t, 3JHH = 7.3 Hz), 6.76 (p-Ph-H, 1H, t, 3JHH = 7.3 Hz), 6.61 (o-Ph-H, 2H, d, 3JHH = 7.6 Hz), 6.48 (o-Ph-H, 2H, d, 3JHH = 7.6 Hz), 5.37 (Ind-H, 2H, s), 2.51 (Ind-CH3, 6H, s), 2.22 (Ind-CH3, 6H, s), 2.21 (Ind-CH3, 6H, s), 2.18 (Ind-CH3, 6H, s), 2.12 (Ind-CH3, 6H, s), 1.71 (Ind-CH3, 6H, s), 1.11 (Zr–CH2-Ph, 2H, s), 0.31 (Zr–CH2-Ph, 2H, s). 13C{1H} NMR (chloroform-d1, 125 MHz, 298 K) δ (ppm): 152.9 (Zr–CH2-Ph), 152.8 (Zr–CH2-Ph), 132.9 (Ind), 131.5 (Ind), 128.0 (Ind), 127.9 (Ind), 127.7 (m-Ph), 127.5 (m-Ph), 127.4 (Ind), 127.1 (o-Ph), 126.8 (o-Ph), 126.3 (Ind), 125.1 (Ind), 121.1 (p-Ph), 120.7 (p-Ph), 113.6 (Ind), 100.1 (Ind-H), 67.6 (Zr-CH2-Ph), 66.8 (Zr-CH2-Ph), 17.6 (Ind-CH3), 16.9 (Ind-CH3), 16.6 (Ind-CH3), 16.5 (Ind-CH3), 14.9 (Ind-CH3), 13.5 (Ind-CH3).
Synthesis of sMAO supported metallocene catalysts
200 equivalents sMAO (39.7 or 41.1% wtAl; 0.300 g, 4.41 or 4.57 mmolAl) were dispersed in toluene (25 mL) to form a colourless slurry. 1.0 equivalent of a metallocene complex (0.0221 or 0.0229 mmol) was dissolved in toluene (25 mL) to form a colored solution which was then quickly added to the sMAO at room temperature. A mixture of rac- and meso-isomer of the complex, and the Al
:
complex molar ratio of 200
:
1 were used unless stated otherwise. The reaction mixture was heated to 60 °C for 2 hours with occasional swirling. The coloured solid was allowed to settle from the clear, colourless solution which was decanted, and the solid was dried in vacuo to afford the product as follows:
Ethylene polymerisation
A supported metallocene catalyst (10.0 mg, 1.0 eqcomplex), TiBA (1000 eq.) and hexane (50 mL) were added to a 150 mL Rotaflo ampoule containing a stirrer bar. The ampoule was sealed and degassed before being heated to the desired temperature at the stirring speed of 1000 rpm. The ampoule was then opened to ethylene (2 bar). On completion of the test, the ampoule was closed to ethylene and degassed. The resulting polyethylene was filtered on a glass sintered frit, washed with pentane (2 × 25 mL) and dried. All polymerisation tests were carried out, at least, in duplicate and run for 30 minutes, unless stated otherwise, or until the stirring ceased entirely. The activities were reported as an average with ±1 ESD error. The molecular weights (Mw) of the resulting polyethylenes were determined by GPC and reported with their corresponding molecular weight distribution (Mw/Mn) values.
Conflicts of interest
There are no conflicts to declare.
Acknowledgements
P. A., J. V. L., J.-C. B., and Z. R. T. (SCG Research Fellowship) would like to thank SCG Chemicals Co., Ltd (Thailand) for financial support; Chemical Crystallography (University of Oxford) for the use of the diffractometers; and Ms Liv Thobru (Norner AS, Norway) for GPC analysis.
Notes and references
- L. Resconi, L. Cavallo, A. Fait and F. Piemontesi, Chem. Rev., 2000, 100, 1253–1346 CrossRef CAS PubMed.
- W. Kaminsky and H. Sinn, Adv. Polym. Sci., 2013, 258, 1–28 CrossRef CAS; Methylaluminoxane: Key Component for New Polymerization Catalysts, ed. W. Kaminsky, Springer. Polyolefins: 50 years after Ziegler and Natta II Search PubMed.
- N. Schneider, M. E. Huttenloch, U. Stehling, R. Kirsten, F. Schaper and H. H. Brintzinger, Organometallics, 1997, 16, 3413–3420 CrossRef CAS.
- M. Bochmann, J. Chem. Soc. Dalton Trans., 1996, 255–270 RSC.
- G. G. Hlatky, Chem. Rev., 2000, 100, 1347–1376 CrossRef CAS PubMed.
- B. Wang, Coord. Chem. Rev., 2006, 250, 242–258 CrossRef CAS.
- O. Olabisi, M. Atiqullah and W. Kaminsky, J. Macromol. Sci., Part C, 1997, 37, 519–554 CrossRef.
- W. Kaminsky and H. Winkelbach, Top. Catal., 1999, 7, 61–67 CrossRef CAS.
- W. Kaminsky, Catal. Today, 1994, 20, 257–271 CrossRef CAS.
- W. Kaminsky, J. Chem. Soc., Dalton Trans., 1998, 1413–1418 RSC.
- W. Kaminsky, Adv. Catal., 2001, 46, 89–159 CAS.
- W. Kaminsky and A. Laban, Appl. Catal., A, 2001, 222, 47–61 CrossRef CAS.
- W. Kaminsky, J. Polym. Sci., Polym. Chem., 2004, 42, 3911–3921 CrossRef CAS.
- W. Kaminsky, A. Funck and H. Hähnsen, Dalton Trans., 2009, 8803–8810 RSC.
- H. G. Alt, Dalton Trans., 2005, 3271–3276 RSC.
- W. Kaminsky, Macromol. Symp., 1995, 97, 79–89 CrossRef.
- C. Janiak and B. Rieger, Angew. Chem., 1994, 215, 47–57 CAS.
- P. A. Zapata, C. Belver, R. Quijada, P. Aranda and E. Ruiz-Hitzky, App. Catal. A Gen., 2013, 453, 142–150 CrossRef CAS.
- D. Bianchini, J. H. Z. dos Santos, T. Uozumi and T. Sano, J. Mol. Catal. A: Chem., 2002, 185, 223–235 CrossRef CAS.
- C. Janiak, B. Rieger, R. Voelkel and H.-G. Braun, J. Polym. Sci., Polym. Chem., 1993, 31, 2959–2968 CrossRef CAS.
- H. Cramail, K. Radhakrishnan and A. Deffieux, Compt. Rend., 2002, 5, 49–52 CrossRef CAS.
- T. Dalet, H. Cramail and A. Deffieux, Macromol. Chem. and Phys., 2004, 205, 1394–1401 CrossRef CAS.
- M. M. Mortazavi, S. Ahmadjo, J. H. Z. Dos Santos, H. Arabi, M. Nekoomanesh, G. H. Zohuri, R. Brambilla and G. B. Galland, J. Appl. Polym. Sci., 2013, 130, 4568–4575 CAS.
- F. Silveira, M. D. C. Martins Alves, F. C. Stedile, S. B. Pergher and J. H. Z. dos Santos, J. Mol. Catal. A Chem., 2010, 315, 213–220 CrossRef CAS.
- J.-C. Buffet, Z. R. Turner, R. T. Cooper and D. O'Hare, Polym. Chem., 2015, 6, 2493–2503 RSC.
- J.-C. Buffet, N. Wanna, T. A. Q. Arnold, E. K. Gibson, P. P. Wells, Q. Wang, J. Tantirungrotechai and D. O'Hare, Chem. Mater., 2015, 27, 1495–1501 CrossRef CAS.
- J. C. Buffet, C. F. H. Byles, R. Felton, C. P. Chen and D. O'Hare, Chem. Commun., 2016, 52, 4076–4079 RSC.
- A. F. R. Kilpatrick, J.-C. Buffet, P. Nørby, N. H. Rees, N. P. Funnell, S. Sripothongnak and D. O'Hare, Chem. Mater., 2016, 28, 7444–7450 CrossRef CAS.
- A. F. R. Kilpatrick, N. H. Rees, S. Sripothongnak, J.-C. Buffet and D. O'Hare, Organometallics, 2018, 37, 156–164 CrossRef CAS.
- P. Angpanitcharoen, G. Hay, J.-C. Buffet, Z. R. Turner, T. A. Q. Arnold and D. O'Hare, Polyhedron, 2016, 116, 216–222 CrossRef CAS.
- P. Angpanitcharoen, J. V. Lamb, Z. R. Turner, J.-C. Buffet and D. O'Hare, Mol. Catal., 2020, 498, 111275 CrossRef CAS.
- T. A. Q. Arnold, J.-C. Buffet, Z. R. Turner and D. O'Hare, J. Organomet. Chem., 2015, 792, 55–65 CrossRef CAS.
- N. E. Grimmer, N. J. Coville, C. B. de Koning, J. M. Smith and L. M. Cook, J. Organomet. Chem., 2000, 616, 112–127 CrossRef CAS.
- N. Piccolrovazzi, P. Pino, G. Consiglio, A. Sironi and M. Moret, Organometallics, 1990, 9, 3098–3105 CrossRef CAS.
- T. A. Q. Arnold, Z. R. Turner, J.-C. Buffet and D. O'Hare, J. Organomet. Chem., 2016, 822, 85–90 CrossRef CAS.
- T. K. Trefz, M. A. Henderson, M. Linnolahti, S. Collins and J. S. McIndoe, Chem. -Eur. J., 2015, 21, 2980–2991 CrossRef CAS PubMed.
- S. Collins, M. Linnolahti, M. G. Zamora, H. S. Zijlstra, M. T. Rodríguez Hernández and O. Perez-Camacho, Macromolecules, 2017, 50, 8871–8884 CrossRef CAS.
- C. Janiak, K. C. H. Lange, U. Versteeg, D. Lentz and P. H. M. Budzelaar, Chem. Ber., 1996, 129, 1517–1529 CrossRef CAS.
- C. Janiak, U. versteeg, K. C. H. Lange, R. Weimann and E. Hahn, J. Organomet. Chem., 1995, 501, 219–234 CrossRef CAS.
- W. Kaminsky, R. Engehausen, K. Zoumis, W. Spaleck and J. Rohrmann, Makromol. Chem., 1992, 193, 1643–1651 CrossRef CAS.
- M. A. Parvez, M. Rahaman, M. A. Suleiman, J. B. P. Soares and I. A. Hussein, Int. J. Polym. Sci., 2014, 1–10 CAS.
- A. Malmberg, J. Liimatta, A. Lehtinen and B. Löfgren, Macromolecules, 1999, 32, 6687–6696 CrossRef CAS.
- M. Pollard, K. Klimke, R. Graf, H. W. Spiess, M. Wilhelm, O. Sperber, C. Piel and W. Kaminsky, Macromolecules, 2004, 37, 813–825 CrossRef CAS.
- A. Ahlers and W. Kaminsky, Makromol. Chemie., Rapid. Commun., 1988, 9, 457–461 CrossRef CAS.
Footnote |
† Electronic supplementary information (ESI) available: NMR spectroscopy, X-ray crystallography, polymerisation data. CCDC 2058030–2058035, 2058038 and 2058039. For ESI and crystallographic data in CIF or other electronic format see DOI: 10.1039/d1ra01912k |
|
This journal is © The Royal Society of Chemistry 2021 |