DOI:
10.1039/D1RA01906F
(Paper)
RSC Adv., 2021,
11, 16096-16105
Recovery of boron from brines with high magnesium content by solvent extraction using aliphatic alcohol
Received
10th March 2021
, Accepted 13th April 2021
First published on 30th April 2021
Abstract
The recovery of boron from salt lake brines has become an effective way to meet the increasing demand, particularly in China. In this study, nine commercially available monohydric alcohols with different structures were selected for boron extraction from a salt lake brine with high magnesium content. Passing through the optimization, isodecanol was finally selected for the detailed study on boron recovery from brine due to its moderate viscosity, lower solubility water entrainment and high extraction efficiency. Parameter effects on boron extraction were systematically studied, including equilibrium pH, organic concentration, phase ratio, temperature, and salting-out effect. A McCabe–Thiele diagram was established to determine the boron extraction and stripping stages. An organic solution containing 2.5 mol L−1 isodecanol was used for a simulated three-stage counter-current extraction test under optimized extraction conditions, and the boron extraction reached 99.07%. A simulated four-stage counter-current stripping test was carried out with water with the stripping efficiency of 98.71%. In total the boron recovery reached 97.79%. Additionally, the mechanism of boron extraction by isodecanol was investigated using both slope analysis method and Fourier transform infrared spectroscopy (FTIR). The stoichiometric ratio of isodecanol to boron required to form boric acid ester was found to be 1.268. These results indicate that the extraction system has great potential for commercial application in boron recovery from salt lake brines with high magnesium content.
1. Introduction
Boron is important to modern industry and has been widely used in many fields, such as chemical catalysis, preparation of building materials and electronic equipment, and generation of nuclear energy. Hard rock ores and salt lake brines are major sources of boron. Salt lake brines account for 33% of the total boron reserves in China, while easily processed boron–magnesium hard rock ores only account for 6.7%.1 Currently, both the reserves and grades of boron–magnesium ores are declining rapidly, leading to a serious resource shortage of boron ores. Therefore, effective and environmentally friendly extraction of boron from salt lake brines has become an important research topic. At present, numerous methods for boron extraction and separation from brines have been widely investigated, including chemical precipitation,2,3 ion exchange,4,5 solvent extraction,6–8 adsorption,9,10 reverse osmosis,11,12 and electrodialysis.13 Compared with other methods, the solvent extraction process has many advantages such as being simple in operation, widely adaptable for different feed solutions, and efficient for boron recovery. Therefore, it has been applied in some commercial practices and is regarded as the most promising method. The mechanism of boron extraction can be classified into three categories:14 (1) formation of neutral esters by reaction of boric acid with alcohol extractants; (2) complexation of borate ions with chelating extractants; and (3) extraction driven by different solubilities of boric acid in two phases. On the basis of these mechanisms, many researchers have developed different types of extractants for boron extraction, such as long-chain alcohols,7,15–17 phenols,18 amine compounds,19,20 and ionic liquids.21,22 The monohydric alcohol is suitable for extracting boric acid under the conditions of high salting-out and acidity. Diol has higher extraction efficiency than monohydric alcohol, but it is difficult to realize large-scale industrial production due to high viscosity and solution loss. Other systems, such as hydroxyl-containing aromatic compounds and amine compounds, have good effects on extracting boron from alkaline salt lake brines. Ionic liquids are still in the stage of laboratory research and have the problems of difficult synthesis.
Nowadays, extraction systems using monohydric alcohols has been widely investigated and used in industrial applications. The extraction mechanism involves monobasic alcohol and boric acid forming boric acid ester compounds, and the extent of combination of alcohol and boric acid determines the extraction efficiency of boron by the extractant. The monohydric alcohols documented in literature include isobutanol, isooctanol, n-octanol and isoamyl alcohol. For example, Zhang et al.15 used 2-ethylhexanol to extract boron from salt lake brine with high magnesium content. A three-stage continuous counter-current extraction and stripping process were conducted and the results showed that an overall boron extraction efficiency was 99.5% with a purity of 95.5%. However, the solubility of 2-ethylhexanol in water was high of 1.0 g L−1, which limited its large-scale applications. Peng et al.7 extracted boron from Qinghai Salt Lake with 2 mol L−1 2-butyl-1-octanol at pH = 1.3. In three-stage continuous counter-current extraction, 99.35% of boron was extracted. A stripping efficiency of 99.63% was obtained using water. However, the price of 2-butyl-1-octanol is high for application to boron extraction in salt lakes on a large scale. Hejda et al.,23 Han et al.,24 and Xu et al.25 also compared the extraction performances of small molecule monohydric alcohols (isobutanol, isoamylol, n-hexanol, n-octanol, isooctanol, etc.), and it was concluded that isooctanol had a highest extraction efficiency. Previous studies were not found to make a systematical comparison in their application of long-chain monohydric alcohols with different structures, especially in extracting boron from salt lake brine with high magnesium content. The effects of physical properties such as structural characteristics, viscosity, and water solubility on the extraction of boron were not explored in detail.
In this paper, different carbon chain structures of monohydric alcohols were selected to extract boron from East Taijinar Salt Lake (Mg/Li = 19.83), and the effects of their structures, solubilities and water entrainment on the extraction performance were systematically compared. Isodecanol was finally selected for further study due to its high extraction efficiency, low solubility and water entrainment amount.
2. Experimental
2.1. Materials and reagents
A concentrated brine with high magnesium content was obtained from the East Taijinar Salt Lake of China, after concentration to recover potassium. The brine composition, pH and density are listed in Table 1. Isodecanol and other monohydric alcohols (n-octanol, 6-methylheptanol, 2-ethylhexanol, 2-octanol, 3,5,5-trimethyl-1-hexanol, n-decanol, isodecanol, 2-propyl-1-heptanol, and isotridecanol) were industrial grade with purity >98%, and they were used for extraction without further treatment. All of them were purchased from Shanghai Aladdin Reagent Co., Ltd. Sulfonated kerosene was used as the diluent and was provided by Sinopharm Group Chemical Reagent Co. Organic solutions were prepared by dissolving alcohol into kerosene to desired concentrations. HCl (37 wt%) were purchased from Beijing Chemical Works. All other reagents used in the tests were analytical reagents, and deionized water was used for aqueous sample preparation.
Table 1 Composition of the concentrated East Taijinar Salt Lake brine
Component |
Li+ |
Na+ |
Mg2+ |
B |
Cl− |
SO42− |
pH |
Density/(g mL−1) |
Concentration (g L−1) |
5.16 |
1.45 |
102.31 |
7.32 |
298.03 |
35.12 |
4.50 |
1.30 |
2.2. Experimental methods
2.2.1. Extraction experiment. The organic phase was mixed with brine at a designated O/A ratio, and then the mixture was placed into a 250 mL beaker and stirred with a magnetic stirrer. The pH was adjusted to the specified value by adding 2 M HCl to the mixture, and then stirring was continued for 15 min to reach equilibrium at room temperature (293 K). And the temperature experiment was conducted in table thermostatic oscillator at variable temperatures, where the temperature was varied over the range of 273–333 K. After the reaction was completed, the mixture was settled for 10 min to separate two phases, and the aqueous phase in the bottom was directly taken for analysis after dilution to determine the element concentrations. The element concentrations in the top organic phase were determined after stripping with 0.25 mol L−1 NaOH.
2.2.2. Stripping experiment. The loaded organic solution and stripping agent were added to 100 mL hexagonal jars at a designed O/A ratio, shaken in a mechanical oscillator (HD100B, Jintan Yineng, China) for 15 min at room temperature (293 K), and then allowed to settle for 10 min to separate the phases. The stripping water phase in the bottom was separated and the element content in the aqueous phase was analysed.
2.2.3. Simulated counter-current extraction. A simulated three-stage counter-current test of boron extraction was carried out at an O/A ratio of 1
:
1 as shown in Fig. 1. The test was stopped after 11 contact rows where extraction equilibrium was expected as the variance of boron concentration in the raffinate aqueous solution was almost neglected. The boron concentrations in the raffinate from the last contact rows of row 11 and row 12 were used to determine calculate the extraction efficiency of boron. The method was also adopted in the simulated multistage counter-current stripping experiment.
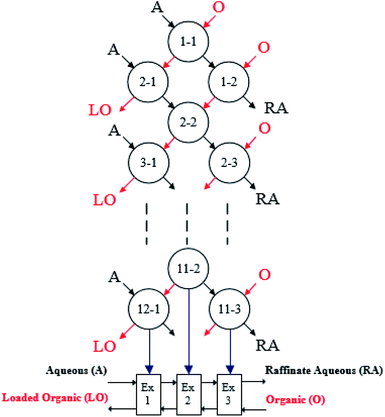 |
| Fig. 1 Process of simulated three-stage counter-current extraction. The aqueous phase (A), organic phase (O), loaded organic (LO), raffinate aqueous (RA) and extraction stage (Ex) are represented in the process. | |
2.3. Analysis
The element concentrations in the aqueous phase were determined by inductively coupled plasma-optical emission spectroscopy (ICP-OES, Optima 5300 V, PerkinElmer, USA) after dilution to a suitable range. The concentration of Cl− was obtained by titration from standard 0.05 mol L−1 AgNO3. And the concentration of SO42− was obtained by precipitation from a 5% solution of BaCl2. The viscosity was measured using a viscometer (NDJ-8S, Pingxuan, China) at room temperature (293 K). The amount of water entrained in the extracted organic phase was determined by an intelligent automatic water titrator (ZSD-2J, Anting, China). The repeatability of the results was checked by repeating the experiment at least twice to ensure that the standard deviation was within 5%. The FTIR spectra were measured in the 4000–500 cm−1 range using a Fourier infrared spectrometer (Nicolet IS50, Thermo, America).
The extraction efficiency (E), stripping efficiency (S) and distribution ratio (D) are calculated with eqn (1)–(3), respectively.
|
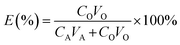 | (1) |
|
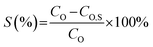 | (2) |
|
 | (3) |
where
CA and
CO are the equilibrium concentrations of elements in the aqueous phase and organic phase, respectively;
VA and
VO are the volumes of the aqueous phase and organic phase, respectively; and
CO,S is the equilibrium concentration of elements in the organic phase after stripping.
3. Results and discussion
3.1. Boron extraction with various structural monohydric alcohols
Several monohydric alcohols have been extensively investigated for boron extraction due to their low price, easy availability and efficient extraction.15 Although boron extraction with monohydric alcohols passes through a mechanism involving ester formation by reaction of hydroxyl groups with boric acid, the structure of the carbon chain has a significant effect on boron extraction. Boron extractions from a concentrated magnesium brine using nine monohydric alcohols with different carbon chains were studied (Fig. 2). The chemical reagents, structures, and some physical properties of the alcohols are shown in Table 2. The percentage (w/w) of water entrainment was measured at an O/A ratio of 1
:
1 and room temperature with results also shown in Table 2.
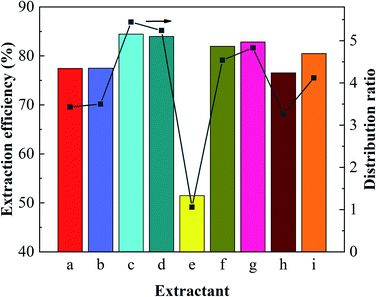 |
| Fig. 2 Extraction performance of different monohydric alcohols. Alcohol concentration, 3 mol L−1; O/A = 1; pH = 2. | |
Table 2 Physical properties of different monohydric alcohols
|
Alcohol |
Structure |
Density (g mL−1) |
Viscosity (cp) |
Solubility in water (g L−1) |
Water entrained in organic (%, w/w) |
A |
(a) n-Octanol |
 |
0.83 |
9.90 |
1.20 |
0.55 |
(b) n-Decanol |
 |
0.83 |
15.10 |
0.12 |
0.53 |
B |
(c) Isooctanol |
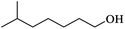 |
0.82 |
10.00 |
1.70 |
0.25 |
(d) 2-Ethylhexanol |
 |
0.83 |
9.80 |
1.70 |
0.27 |
(e) 2-Octanol |
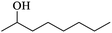 |
0.82 |
9.80 |
1.70 |
0.44 |
(f) 3,5,5-Trimethyl-1-hexanol |
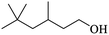 |
0.82 |
14.20 |
1.00 |
0.16 |
(g) Isodecanol |
 |
0.84 |
16.30 |
0.17 |
0.17 |
(h) 2-Propyl-1-heptanol |
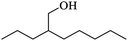 |
0.83 |
16.90 |
0.17 |
0.27 |
(i) Isotridecanol |
 |
0.84 |
40.70 |
— |
0.16 |
From Fig. 2, it is obviously that boron extraction with 2-octanol (d), a secondary alcohol was much lower than other primary alcohol. This suggests that secondary alcohols are inefficient for boron extraction maybe due to the high steric hindrance for borate ester formation, and it has been proved that the activities of secondary alcohols were much lower than those of primary alcohols in ester formation with carboxylic acids.26,27
By comparing the extraction performances and physical properties of the alcohols represented as group A and group B in Table 2, names straight-chain alcohols and branched aliphatic alcohols. It was found that the extraction performances of the latter were better than those of the former. These results could be attributed to the fact that the electron-donating properties of the branched alkyl groups made the hydroxyl oxygen atoms strongly electronegative, and thus the covalent bond formed between the oxygen atom and the boron atom were stronger. The generated borate esters were more stable and difficult to hydrolyze. Therefore, the extraction efficiencies for boron were higher than those with straight-chain alcohols. In addition, the extents of water entrainments by monohydric alcohols with branched chains are obviously less than those of straight-chain alcohols.28 For example, the water entrainment amount of n-decanol is 0.533%, while that of isodecanol is only 0.170%. Reducing the water entrainment in the extraction process could significantly improve the phase separation and eliminate the occurrence of emulsification problem.29,30
Three alcohols marked c, g and i in Table 2 all have branched chains with different carbon chain numbers. The extraction efficiencies of all these three alcohols were more than 80% with isooctanol being the highest of 84.46% (Fig. 2). Table 2 also shows that the viscosity gradually increases with the increase in the number of carbon atoms. The viscosity of isotridecanol, with 13 carbon atoms, is the largest of 40.7 cp. Since high viscosity can detriment phase separation, isotridecanol is considered less suitable for boron extraction from salt lake brines compared with the other two alcohols. In addition, the solubilities of monohydric alcohols decrease gradually with increases of the number of carbon atoms due to the longer carbon chains with larger hydrophobic groups reducing the polarities of the monohydric alcohols. Excessive alcohol dissolution in the brine will reduce its recycle performance for boron extraction, and the organic loss in the brine should be intensively removed to meet the discharge requirement for clean production, which would inevitably increase the operating cost. Therefore, isodecanol (g), with lower water solubility, is more suitable than isooctanol (c) for the extraction of boron from the brine. The extraction efficiency of isodecanol was 82.85% with the water solubility of 0.17 g L−1 and water entrainment of only 0.170%. At present, there are few studies on the application of isodecanol in extracting boron from salt lakes with high magnesium content.7,15 Therefore, isodecanol was selected for in-depth study in this experiment.
3.2 Boron extraction with isodecanol
3.2.1. Effect of the isodecanol concentration. Organic phases containing various concentrations of isodecanol (1–4 mol L−1) in kerosene were used to extract boron from the brine at an O/A ratio of 1
:
1, with the results shown in Fig. 3. The extraction efficiency of boron increased with increasing isodecanol concentration. When the concentration of isodecanol was 2.5 mol L−1, the boron extraction efficiency was 80.20%. As the isodecanol concentration increased, the boron extraction efficiency increased slowly. When the concentration of isodecanol was 4 mol L−1, the boron extraction efficiency reached 88.48%. The distribution ratio showed the same trend as the extraction efficiency, and it also increased with increasing isodecanol concentration. This is due to more isodecanol molecules participated in the formation of borate esters with increasing alcohol concentration. When the reaction reached extraction equilibrium, the extraction efficiency was no longer changed. However, as shown in Fig. 4, the viscosity of the extraction system increased gradually with increasing isodecanol concentration. Too high viscosity could decrease the mass transfer rate and increase the phase separation time. In considering raising the boron extraction and lowering the system viscosity, 2.5 mol L−1 isodecanol was finally optimised for further investigations.
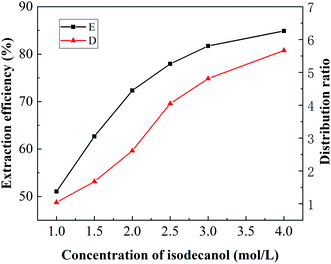 |
| Fig. 3 Effect of the isodecanol concentration on the extraction of boron. | |
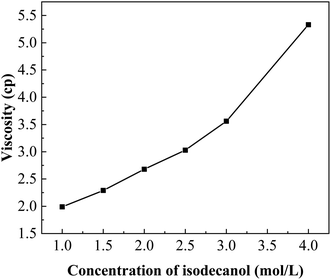 |
| Fig. 4 The viscosity of different isodecanol concentrations. | |
3.2.2. Effect of equilibrium pH. Boron was extracted with 2.5 mol L−1 isodecanol at an O/A ratio of 1
:
1, and various pH adjusted by adding hydrochloric acid to the mixed phase to study the effect of equilibrium pH on the extraction efficiency. As shown in Fig. 5, the extraction of boron was almost unchanged when the pH was 0–3.5. With the increase in equilibrium pH from 3.5 to 5.5, the extraction efficiency and distribution ratio for boron decreased rapidly from 87.38% to 37.07% and 6.92 to 0.59, respectively. These results could be attributed to the lower pH inhibiting the dissociation of boric acid and promoting its reaction with isodecanol. Boron existed mainly in the form B(OH)4− as the equilibrium pH increased, which was unfavourable for the formation of borate ester and thus reduced the extraction efficiency. Considering the amount of hydrochloric acid used and the corrosion resistance of the equipment, an equilibrium pH of 3.5 was selected for future research.
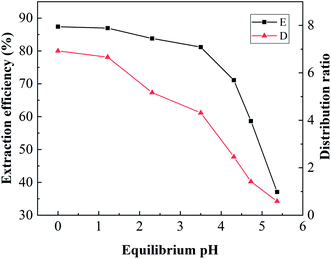 |
| Fig. 5 Effect of the equilibrium pH on the extraction of boron. | |
3.2.3. Effect of the phase ratio (O/A). To investigate the effect of the O/A ratio on boron extraction, an organic solution containing 2.5 mol L−1 isodecanol was mixed with the brine at different O/A ratios at an equilibrium pH of 3.5. The results are shown in Fig. 6. The extraction of boron increased with increasing O/A ratio. When the O/A ratio increased from 0.25 to 1, the extraction of boron increased significantly from 29.48% to 78.83%. As the O/A ratio further increased, the extraction of boron increased slightly. However, the concentration of boron in the organic phase decreased with increasing volume of the organic phase. When the O/A ratio was 3, the concentration of boron in the organic phase was only 2.65 g L−1. To obtain high boron extraction efficiency and boron loading in the organic phase, an O/A ratio of 1
:
1 was selected.
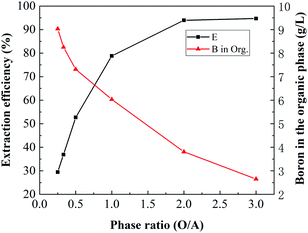 |
| Fig. 6 Effect of the phase ratio on the extraction of boron. | |
3.2.4. Effect of the temperature. Boron extraction from brine was conducted at different temperatures by using an organic solution containing 2.5 mol L−1 isodecanol at an O/A ratio of 1
:
1 and equilibrium pH of 3.5. The results are shown in Fig. 7. The extraction efficiency and distribution ratio gradually decreased with increasing temperature. When the temperature increased from 273 K to 333 K, the extraction efficiency decreased from 83.93% to 73.09% and the distribution ratio also decreased to 4.62. The enthalpy change (ΔH) for boron extraction could be determined based on the slope of the plot of lg
DB versus 1000/T (K−1) using eqn (4) of the van't Hoff equation (Fig. 8): |
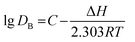 | (4) |
where R is the universal gas constant (8.314 J mol−1 K−1) and C is the integration constant. The ΔH of the system was determined to be −9.248 kJ mol−1, suggesting that the extraction of boron by isodecanol was an exothermic reaction and lowering the temperature was favourable for boron extraction. Considering that low temperature could increase the viscosity of the organic solution and phase separation time, room temperature (293 K) was chosen for the extraction of boron.
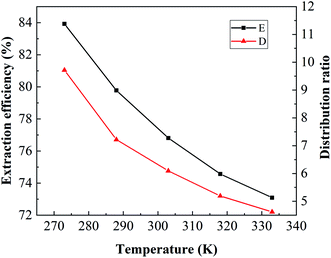 |
| Fig. 7 Effect of temperature on the extraction of boron. | |
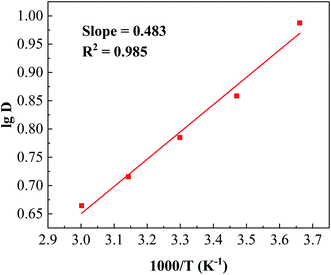 |
| Fig. 8 The linear relationship between the lg of the distribution ratio and 1000/T. | |
In addition, eqn (5) and eqn (6) were used to calculate the changes of Gibbs free energy (ΔG) and entropy (ΔS) at room temperature.
|
ΔG = − 2.303RT lg k
| (5) |
|
 | (6) |
where the lg
k was obtained from
Fig. 14 in Section 3.5. The values of the changes in Gibbs free energy (Δ
G) and entropy (Δ
S) were calculated as Δ
G = −0.126 kJ (mol
−1 K
−1) and Δ
S = −31.133 J mol
−1. These results indicated that the extraction of boron by isodecanol was a spontaneous process with decreased entropy.
3.2.5. Salting-out effect. To study the effect of the metal cations in brines on boron extraction, simulated brine solutions containing different concentrations of MgCl2, NaCl, LiCl and the same concentration of boron (6.95 g L−1) were mixed with 2.5 mol L−1 isodecanol at an O/A ratio of 1
:
1 and an equilibrium pH of 3.5. As shown in Fig. 9, the extraction efficiency increased with increasing metal cation concentration, which indicates that the addition of Mg2+, Na+ and Li+ can promote the extraction of boron. The possible reason for the explanation is that water molecules can be bound with metal cations, increasing the solution activities, which indirectly increased the concentration of boric acid and consequently shifted the extraction equilibrium towards the direction of esterification. In addition, Fig. 9 shows that the efficiency of boron gradually increased with the increase in metal cation concentration and increase in Mg2+ concentration has greater effect than that of Li+ and K+. When the Mg2+ concentration was increased from 0.85 mol L−1 to 4.25 mol L−1, the boron extraction efficiency increased significantly from 37.95% to 80.81%. This result could be attributed to the fact that the higher charge of Mg2+ leads to a stronger polarization capability, resulting in a stronger hydration effect. As the salt lake brine used in this experiment contains high concentration of magnesium is a naturally existed salting-out agent which was beneficial for the extraction of boron.
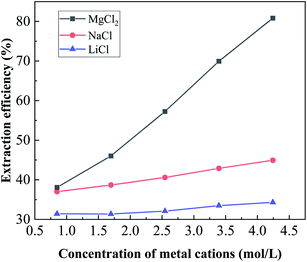 |
| Fig. 9 Effect of the different metal cation concentrations on the extraction of boron. | |
The salting-out effect of different metal cations was determined by the distribution ratio of boron extraction based on the empirical formula as shown in eqn (7) (reference):
|
lg (D1 − D2) = m lg n + lg C
| (7) |
where
D1 is the distribution ratio in the presence of the salting-out agent,
D2 is the distribution ratio in the absence of the salting-out agent, and
n is the concentration of added metal ions. The constants of
m and
C are related to the type of salting-out.
Fig. 10 shows the result of linear fitting of lg (
D1 −
D2) and lg
n, and the fitting parameters are shown in
Table 3. The fitting parameters show that the three salting-out agents all showed good linear relationships in
eqn (7). In addition, the constant
m changed in the order
m(MgCl2) >
m(NaCl) >
m(LiCl), which indicates that the order of salting-out capability for the three salts is MgCl
2 > NaCl > LiCl.
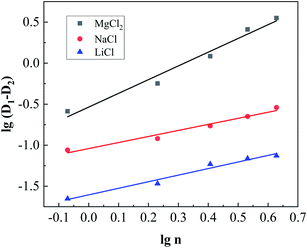 |
| Fig. 10 Linear relationship between lg (D1 − D2) and lg n. | |
Table 3 Linear fitting parameters of different salting-out agents
Salting-out agent |
M |
lg C |
R2 |
MgCl2 |
1.67 |
−0.54 |
0.98 |
NaCl |
0.80 |
−1.61 |
0.97 |
LiCl |
0.74 |
−1.04 |
0.97 |
3.3. Boron stripping with isodecanol
3.3.2. Effect of the phase ratio (O/A). To investigate the effect of the phase ratio on the boron stripping process, the loaded organic phase containing 4.3 g L−1 boron was mixed with deionized water at different O/A ratios ranging from 0.25–5. As shown in Fig. 11, with the increase in the O/A ratio, the stripping efficiency of boron decreased significantly, while the boron concentration in the aqueous phase increased significantly. This result may be due to the increase in boron concentration in the aqueous phase, which promoted its transfer to the organic phase. To obtain higher boron stripping and boron concentrations in the aqueous phase, a stripping ratio of 1
:
1 was selected.
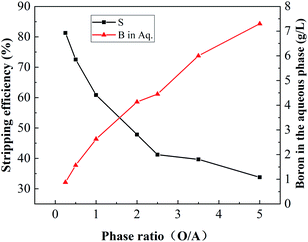 |
| Fig. 11 Effect of the phase ratio on the stripping process. | |
3.3.3. Effect of temperature on the stripping. The boron-loaded organic solution was mixed with deionized water at an O/A ratio of 1
:
1 and a temperature range of 273–323 K to study the stripping of boron with results shown in Fig. 12. Unlike the extraction process, the stripping efficiency increased gradually with increasing temperature, and it was suggested that the stripping process was an endothermic reaction. When the temperature was 293 K, the stripping efficiency was 62.7% and gradually increased to 74.17% when increased temperature to 323 K. High temperature could increase the organic loss via evaporation, leading to the increase in operating cost. As boron stripping was reasonably good at room temptation, further stripping tests were carried out at room temperature (293 K).
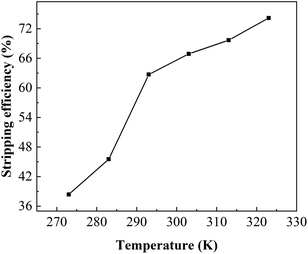 |
| Fig. 12 Effect of the temperature on the stripping process. | |
3.4. Simulated multistage counter-current extraction and stripping
The theoretical extraction and stripping stage were determined by a McCabe–Thiele diagram (Fig. 13). According to the above boron extraction and stripping experiments with different phase ratios, the concentrations of boron in the two phases at equilibrium were used to plot the equilibrium line. Fig. 13(a) shows that three theoretical stages were required to extract boron almost completely from the brine by using 2.5 mol L−1 isodecanol at an O/A ratio of 1
:
1. Eleven series of batch-row contacts of the three-stage counter-current extraction were conducted to reach extraction equilibrium (Fig. 1), and the results are shown in Table 5. It was found that 99.07% of boron in the brine was extracted through three-stage counter-current extraction, the concentration of boron in the loaded organic phase was 7.363 g L−1 (Table 5). And Fig. 13(b) also shows that four theoretical stages were required to strip boron more completely from the loaded organic phase with water at an O/A ratio of 1
:
1. The stripping of boron reached 98.71% with four-stage counter-current stripping, and the concentration of boron in the strip liquor reached 7.172 g L−1 (Table 5). The total boron recovery of the whole process was 97.79%. The above results showed that the system has a high boron recovery efficiency and can effectively selectively extract boron from salt lake brine with a high magnesium content.
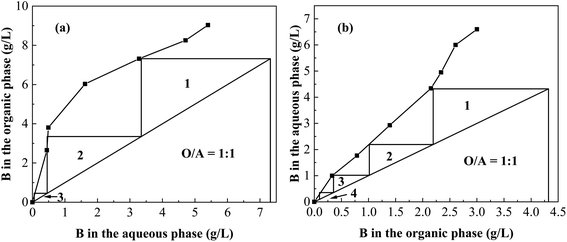 |
| Fig. 13 McCabe–Thiele diagram to determine the number of extraction stages (a) and stripping stages (b). | |
Table 5 Results of simulated multistage counter-current extraction and stripping
Stage |
Extraction |
Stripping |
B in Aq. (g L−1) |
B in Org. (g L−1) |
Total E (%) |
B in Aq. (g L−1) |
B in Org. (g L−1) |
Total S (%) |
1 |
1.20 |
7.36 |
83.69 |
7.17 |
2.36 |
67.23 |
2 |
0.12 |
1.01 |
98.38 |
1.69 |
1.19 |
83.55 |
3 |
0.07 |
0.11 |
99.07 |
0.51 |
0.35 |
95.22 |
4 |
— |
— |
— |
0.16 |
0.09 |
98.71 |
3.5. Extraction mechanism of boron by isodecanol
Boric acid is a weak acid, and it can hydrolyse in water to release protons,14 as shown in eqn (8). |
 | (8) |
The form of boric acid existing in the aqueous phase is related to pH, with a boric acid molecule present under acidic conditions and borate ions present under alkaline conditions. Isodecanol can react with the boric acid molecule as in eqn (9) to form borate, so a high distribution ratio can be obtained under acidic conditions.
|
 | (9) |
The distribution ratio D can be expressed as in eqn (10). Taking the logarithm of eqn (10) one can obtain the linear relationship between lg[ROH] and lg
D, as shown in eqn (11).
|
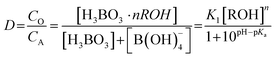 | (10) |
|
lg D = n lg[ROH] + lg K1 − lg(1 + 10pH−pKa)
| (11) |
Boron was extracted with different concentrations of isodecanol (1–4 mol L−1) at an O/A ratio of 1
:
1. Plotting lg
D against lg [ROH] gives a straight line with a slope equal to 1.268 (Fig. 14). This slope means that n = 1.268 in eqn (11), indicating that one molecule of boric acid reacts with 1.268 equivalents of isodecanol during extraction. To determine the structure of the extraction species, the Fourier transform infrared spectra (FTIR) of the organic phase before and after extraction were analysed, and the results are shown in Fig. 15. The O–H stretching vibration of isodecanol in the 3200–3550 cm−1 region disappeared after extraction. Additionally, high-intensity bands appeared at 1416 cm−1, 1335 cm−1 (B–O stretching vibration), and 665 cm−1 (BO3 deformation vibration). These results indicate that the hydroxyl group on isodecanol reacted with boric acid to ultimately generate ester compounds.
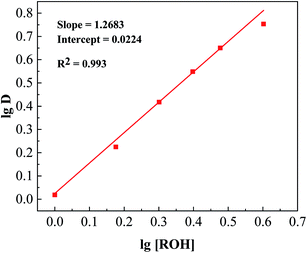 |
| Fig. 14 The linear relationship between the lg of the distribution ratio and lg of the concentration of isodecanol. | |
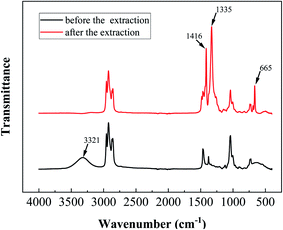 |
| Fig. 15 Fourier transform infrared spectra of the organic phase obtained before and after extraction. | |
4. Conclusions
For the boron extraction from a brine with high magnesium concentration isodecanol was preferred among nine commercially available monohydric alcohols studied. The efficiency of boron extraction with the alcohols having branched chains were significantly higher than those of the straight chain alcohols with the same number of carbon atoms. Isodecanol, as the preferred alcohol, was suitable for boron extraction from the East Taijinar Salt Lake brine due to its moderate viscosity of 16.3 cp, low solubility of 1.7 g L−1 and water entrainment of 0.170%. Under the optimal extraction conditions of 2.5 mol L−1 isodecanol at equilibrium pH = 3.5 and with an O/A ratio of 1
:
1, the single-stage extraction efficiency was 81.67%. The thermodynamic results showed that the extraction of boric acid by isodecanol was an enthalpy-driven exothermic process. The salting-out effect of cations promoted the extraction of boric acid by isodecanol, and the order of effectiveness was MgCl2 > NaCl > LiCl. With three-stage simulated counter-current extraction and four-stage simulated counter-current stripping, the total boron recovery reached 97.79%. The combination of infrared spectroscopy and slope analysis revealed that isodecanol reacted with boric acid at a stoichiometric ratio of 1.268 to form the borate ester. The above results showed that this process has the potential for large-scale application of boron extraction from salt lake brines with a high magnesium content.
Conflicts of interest
There are no conflicts to declare.
Acknowledgements
This work was supported by the National Natural Science Foundation of China (51774260), Beijing Natural Science Foundation (2202053), and the autonomic deployment project of Innovation Academy for Green Manufacture, Chinese Academy of Sciences (IAGM-2019-A15), the Key Research Program of Frontier Sciences of Chinese Academy of Sciences (Grant No. QYZDJ-SSW-JSC021) and CAS Interdisciplinary Innovation Team.
References
- L. L. Wang, Q. S. Wang and L. Wu, China Mining Magazine, 2019, 28, 74–78 Search PubMed.
- A. E. Yilmaz, R. Boncukcuoğlu, S. Bayar, B. A. Fil and M. M. Kocakerim, Korean J. Chem. Eng., 2012, 29, 1382–1387 CrossRef CAS.
- P. Remy, H. Muhr and E. Plasari, Environ. Prog., 2005, 24, 105–110 CrossRef CAS.
- J. Kluczka, T. Korolewicz, M. Zołotajkin and J. Adamek, Water Resour. Ind., 2015, 11, 46–57 CrossRef.
- N. B. Darwish, V. Kochkodan and N. Hilal, Desalination, 2015, 370, 1–6 CrossRef CAS.
- X. B. Fan, X. P. Yu, Y. F. Guo and T. L. Deng, J. Chem., 2018, 2018, 7530837 Search PubMed.
- X. W. Peng, L. J. Li, D. Shi, L. C. Zhang, H. F. Li, F. Nie and F. G. Song, Hydrometallurgy, 2018, 177, 161–167 CrossRef CAS.
- T. Kwon, M. Hirata, S. Sakuma, T. Hano and T. Yamagishi, Solvent Extr. Ion Exch., 2005, 23, 391–400 CrossRef CAS.
- J. Kluczka, W. Pudło and K. Krukiewicz, Chem. Eng. Res. Des., 2019, 147, 30–42 CrossRef CAS.
- Z. C. Çelik, B. Z. Can and M. M. Kocakerim, J. Hazard. Mater., 2008, 152, 415–422 CrossRef PubMed.
- S. Wang, Y. Zhou and C. Gao, J. Membr. Sci., 2018, 554, 244–252 CrossRef CAS.
- D. Chen, X. Zhao and F. Li, Desalination, 2015, 370, 72–78 CrossRef CAS.
- M. Noguchi, Y. Nakamura, T. Shoji, A. Iizuka and A. Yamasaki, J. Water Process. Eng., 2018, 23, 299–305 CrossRef.
- Y. Xu and J. Q. Jiang, Ind. Eng. Chem. Res., 2008, 47, 16–24 CrossRef CAS.
- R. Zhang, Y. M. Xie, J. F. Song, L. X. Xing, D. F. Kong, X. M. Li and T. He, Hydrometallurgy, 2016, 160, 129–136 CrossRef CAS.
- B. Tural, S. Tural and H. Hosgoren, Turk. J. Chem., 2007, 31, 163–170 CAS.
- M. Karakaplan, S. Tural, M. Sunkur and H. Hosgoren, Sep. Sci. Technol., 2003, 38, 1721–1732 CrossRef CAS.
- M. Yurdakoc, M. Karakaplan and H. Hossgooren, Sep. Sci. Technol., 1999, 34, 2615–2625 CrossRef CAS.
- N. Bicak, M. Gazi and B. F. Senkal, React. Funct. Polym., 2005, 65, 143–148 CrossRef CAS.
- M. Gazi and N. Bicak, React. Funct. Polym., 2007, 67, 936–942 CrossRef CAS.
- A. Fortuny, M. T. Coll and A. M. Sastre, Sep. Purif. Technol., 2012, 97, 137–141 CrossRef CAS.
- M. T. Coll, A. Fortuny and A. M. Sastre, Chem. Eng. Res. Des., 2014, 92, 758–763 CrossRef CAS.
- J. Hejda and V. Jedinakova, J. Radioanal. Chem., 1983, 80, 23–29 CrossRef CAS.
- L. J. Han, Y. J. Kong and H. M. Li, Nat. Prod. Res. Dev., 2006, 18, 144–146 Search PubMed.
- G. Y. Xu, H. Q. Yin, J. C. Sai E, Q. C. De and Q. W. Sun, J.
Hefei Univ. Technol. (Nat. Sci.), 2016, 39, 985–987 Search PubMed.
- Y. Wang, Chin. J. Pharm., 2004, 35, 266 Search PubMed.
- S. K. Stephenson, R. D. Offeman, G. H. Robertson and W. J. Orts, Chem. Eng. Sci., 2007, 62, 3019–3031 CrossRef CAS.
- C. Fong, T. L. Greaves, T. W. Healy and C. J. Drummond, J. Colloid Interface Sci., 2015, 449, 364–372 CrossRef CAS PubMed.
- W. Wu, D. Li, Z. Zhao, J. Chen, F. Zhang, S. Yin, M. Qian and X. Bian, J. Rare Earths, 2010, 28, 174–178 CrossRef.
- Y. Dong, X. Sun, Y. Wang, C. Huang and Z. Zhao, ACS Sustainable Chem. Eng., 2016, 4, 1573–1580 CrossRef CAS.
|
This journal is © The Royal Society of Chemistry 2021 |
Click here to see how this site uses Cookies. View our privacy policy here.