DOI:
10.1039/D1RA01375K
(Paper)
RSC Adv., 2021,
11, 12568-12577
Achievement of narrow-band blue-emitting phosphors KScSr1−yCaySi2O7:Bi3+ by the migration of luminescence centers†
Received
20th February 2021
, Accepted 24th March 2021
First published on 30th March 2021
Abstract
In recent years, efforts have been made to develop narrow-band emission phosphors with excellent performance. Herein, a series of KScSr1−yCaySi2O7:0.07Bi3+ narrow-band phosphors were synthesized by a co-substitution method, and the crystal structure, the occupancy of activated ions and luminescence properties were studied in detail. The substitution of Ca2+ for Sr2+ ions resulted in the migration of the activated Bi3+ from the K site to Sr site, accompanied by the regulation of the emission peak from 410 nm to 455 nm, the peak emission half width from 52 nm to 40 nm, and the color purity from the original 78% to 88%. In addition, a warm white LED with low CCT = 3401 K, CRI = 95.5, and CIE color coordinates of (0.3447, 0.3682) has been obtained through the combination of KSS0.6C0.4S:0.07Bi3+ with a commercial green and red phosphor on a UV (370 nm) chip. The results not only provided a strategy based on the manipulation of chemical composition and crystal structure to tune spectral distribution, but also broadens the choice of activators of narrow-band blue-emitting phosphors.
1. Introduction
Phosphor converted white light-emitting diodes (pc-LEDs) are emerging backlight units in modern liquid crystal display (LCD) applications.1 Over the past decade, many efficient phosphors have been developed, most of these phosphors have wide-band emission. The wavelength of some phosphors exceeds the sensitive area of human eyes (700 nm), resulting in the reduction of the luminous efficiency of pc-LEDs. Therefore, over the years people have been committed to improve the resolution of the LCD, power consumption and color gamut. The luminescence characteristics of light, such as position of emission peak and full-width at half-maximum (FWHM), will directly affect the space of color gamut.2–6 The improvement of color gamut is a hot issue because it can make the color of LCDs more vivid and attractive to consumers. Phosphors, often with narrow emission bands, can produce a wider gamut. Therefore, in order to obtain a large gamut in the pc-LED backlight, narrow-band emission phosphors with good performance need to be developed.
As early as a few years ago, the special luminescence characteristics of narrow-band phosphors attracted the attention of academic and industrial circles. For example, phosphors like Sr[Mg3SiN4]:Eu2+ (SMS) and Sr[Mg2Al2N4]:Eu2+ (SMA) were reported by Schnick's group, and they presented narrow emission, good luminescence efficient and thermal stability.7,8 Recently, Hubert's group reported KLi3SiO4:Eu2+ with a broad-band, near warm white emission (FWHM = 49 nm, λem = 515 nm) in the same oxide-based system.9,10 These studies tend to focus on the effects of symmetric cation sites and highly condensed skeletons on the emissive bands, and most of them are doped Eu2+ or Mn4+ ions. However, these elements, especially Eu2+, are usually reabsorbed in the visible region, resulting in a decrease in luminous efficiency. Therefore, it is necessary to develop a pair of narrow-band blue luminescent phosphors that is highly efficiently absorbed in the NUV region and matched well with NUV commercial LED chips to further improve the performance of LCD.
As a favored sensitizer or an activator in luminescent materials, the spectroscopy of Bi3+ ions has been extensively investigated.11 Compared with rare earth luminescence centers, the absorption of Bi3+ is mainly concentrated in the NUV region, with no reabsorption and high energy efficiency. Depending of the nature of the host lattice in which Bi3+ ion is inserted, this ion may show either a regular luminescence, a cluster-related luminescence, and so on. The regular Bi3+ luminescence results from intra-ionic transitions between the ground 6s2 and first excited 6s16p1 configurations. Absorption takes place from 1S0 fundamental state to 3P1, 3P2 and 1P1 excited states.12,13 Benefiting from its naked 6s and 6p orbits, Bi3+ has a broadly adjustable luminescence spectrum, affected by different crystal field environments, which opens up great possibilities for ion regulation. For instance, Peng's team reported CaMoO4:Bi3+ phosphors, half peak width is about 200 nm, mainly studies the cationic alternative implementation color adjustable launch in 2014.14 Moreover, they obtained (Y, Sc)(Nb, V)O4:Bi3+ phosphors, which also changed the half-peak width from 120 nm to 230 nm through ion regulation in 2016;15 In summary, Bi3+ ions can not only achieve efficient absorption in the NUV region, but also its naked orbits is very conducive to the matrix composition regulation, so as to obtain phosphors with excellent performance.
In our work, a series of narrow-band blue-emitting phosphors KScSrSi2O7:xBi3+ (0 < x < 0.13) and KScSr1−yCaySi2O7:0.07Bi3+ (0 < y < 0.05) have been prepared by high temperature solid state reaction. Take advantage of the sensitivity of Bi3+ ions to the surrounding coordination environment and crystal field, Ca2+ ions were introduced into KScSrSi2O:0.07Bi3+ to replace Sr2+, control the occupying selection of activated ion Bi3+ from K site to Sr site and the luminescence color was adjusted from purple to blue, the structure rigidity and lattice distortion of KScSr1−yCaySi2O7:0.07Bi3+ were changed by cationic component regulation, and a narrow-band emission with a half-peak width of 40 nm was achieved.
2. Experimental section
2.1 Sample preparation
A series of KScSrSi2O7:xBi3+ (KSSS:xBi3+) (0 < x < 0.13) and KScSr1−yCaySi2O7:0.07Bi3+ (KSS1−yCy:0.07Bi3+) (0 < y < 0.5) phosphors were synthesized by high temperature solid-state method. The raw materials used are K2CO3 (99.99%), Sc2O3 (99.99%), SrCO3 (99.9%), SiO2 (99.9%, D50∼10 μm), CaCO3 (99.9%) and Bi2O3 (99.99%). The raw materials are weighed and mixed in an agate mortar according to certain stoichiometric and fully ground for 30 minutes. Then the mixed powder samples are transferred into an alumina crucible and sintered at a high temperature of 1200 degrees for 5 h. Finally, all obtained powder samples are naturally cooled to room temperature in the furnace and ground for further experimental measurement.
2.2 Characterization
The phase structure of the sample was characterized by Bruker D8 X-ray diffractometer. The corresponding parameters were as follows: the radiation source was CuKα (λ = 0.15406 nm), the working voltage and current were 40 kV and 40 mA, respectively. The scanning range was 10–80° and the step was 0.02° per s. And the Findit software and Crystalmaker software were used for the structure. Crystal structure refinements were implemented using General Structure Analysis System (GASA). The emission and excitation spectra of samples were recorded by using a Hitachi F-7000 spectrophotometer equipped with a 450 W xenon lamp as the excitation source. The scanning speed was 1200 nm min−1 with a step of 0.2 nm. At room temperature, the decay curves were measured by Horiba FL3 with the width of the incident and outgoing slits is 1 nm, and the width of the slit of the sample is 29 nm.
3. Results and discussion
3.1 Crystal structure characterizations
The XRD patterns of KSSS:xBi3+ (0 < x < 0.13) and KSS1−yCy:0.07Bi3+ (0 < y < 0.5) are shown in Fig. S1.† The diffraction peaks of all the samples were consistent with the structure of KSSS standard card, indicating that all the samples were pure phase and the introduction of Bi3+ and Ca2+ ions did not change the crystal structure of the matrix. According to the rule of ion radius matching, Bi3+ ions were expected to occupy the positions of Sr2+ and K+ ions. On account of Bi3+ was very sensitive to different coordination environments, the crystal field environment around Bi3+ can be changed to achieve the regulation of the luminescence performance of Bi3+ doped phosphors. In this paper, the luminescence properties of phosphors were improved by using cationic substitution of Ca2+ for Sr2+ to adjust the coordination environment around Bi3+ ions.
As shown in Fig. 1(a), the crystal structure of KScSrSi2O7 belongs to the KMRESi2O7 family (M = Ba2+, Sr2+, RE = Y3+, Sc3+), which belongs to the P121/n1 space group and consists of a tetrahedron and octahedron containing Si2O7 groups and octahedral coordination with Sc atoms (〈Sc–O〉 = 2.12 Å). The framework of KMRESi2O7 is based on a ScO6 octahedron which shares each of its apices with an oxygen atom of the Si2O7 group. Adjacent ScO6 octahedra is connected to each other through Si2O7 units, whose Si–Si vectors are oriented parallel to the c axis. This connection creates layers of ScO6 polyhedra in the x–y plane, furthermore, a three-dimensional framework with 8-coordinated Sr atoms (〈Sr–O〉 = 2.71 Å) and 9-coordinated K cations (〈K–O〉 = 2.86 Å) located in voids. The three-dimensional connectivity generates a rigid lattice exhibiting highly condensed network. And the two cations are “stacked” in narrow channels, and they are arranged in strict order.16 In the KMRESi2O7 family, KSSS has a relatively small bond angle and bond length, which infers that it has a more rigid structure. There are three cation sites in KSSS, K+ (r = 1.55 Å, CN = 9), Sr2+ (r = 1.26 Å, CN = 8) and Sc3+ (r = 0.745 Å, CN = 6). Due to the exposed electron configuration in the outermost layer of Bi3+ ion, the luminescence of Bi3+ is very sensitive to the surrounding crystal field environment. The different symmetry of multivalent cations (Sr2+/K+/Sc3+) provide infinite possibilities for controlling the selection of Bi3+ at different sites and the regulation of luminescence performance. In order to further prove Bi3+ successfully doped into the crystal structure, KSSS:xBi3+ were refined with GSAS software, and the refining results were shown in Fig. 1(b). The cross represents the experiment raw data, the red solid line represents the calculated data, the blue solid line represents the difference between the observed data and calculated data, the green solid line represents the Bragg position. This refined result shows that the parameters meet the requirements, in other words, all samples are single phase. Other concentration refining results are shown in the Fig. S2.† It is known that the generation of cationic vacancies should contribute to the shrinkage of the crystal cell volume. The cationic vacancies should be produced when the Sr2+ ions positions were occupied by Bi3+ ions because of the integral charge balance. It can be expressed by the following formula (1):17
|
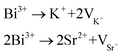 | (1) |
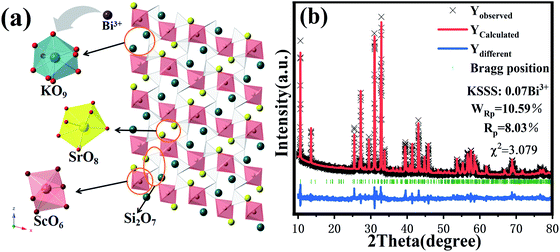 |
| Fig. 1 (a) The crystal structure of KScSrSi2O7; (b) Rietveld structure refinement patterns of KScSrSi2O7:0.07Bi3+. | |
3.2 Luminescence properties of KScSrSi2O7:xBi3+
Fig. 2(a) shows the emission spectra of KSSS:0.07Bi3+ at different excitation wavelengths of 340 nm and 370 nm, as well as the excitation spectra corresponding to the main emission peak of 415 nm and 500 nm. It is obvious that KSSS:0.07Bi3+ has a site-selective excitation and its emission spectrum varies with the excitation wavelength. And the excitation spectrum presents a wide 260–400 nm excitation band centering at 280 nm and 370 nm, which indicates that it can be well matched with commercial near ultraviolet LED chips. There are three different cation sites in KSSS crystal, and Bi3+ is extremely sensitive to the surrounding coordination environment, therefore, the asymmetric emission may be caused by the substitution of Bi3+ for different cation sites. It is generally believed that the doping of Bi ions at trivalent or bivalent sites with coordination number CN larger than or equal to 6 is the most probable.18 In order to further determine the number of potential luminous centers, the radius percentage difference between the doped ion Bi3+ and the potentially substituted ion (Sr2+/K+/Sc3+) in KSSS is calculated by using formula (2), and is listed in Table 1.19 |
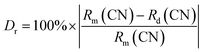 | (2) |
where Dr is the percentage difference of ion radius, CN is the coordination number, Rm (CN) and Rd (CN) are the radii of substituted ions and doped ions, respectively. Since the data of CN = 9 of Bi3+ ion is insufficient, a reasonable approximation is provided here. Table 1 presents the Rm (CN), Rd (CN) and Dr values. The corresponding Dr values between Bi3+ ions and cations (K+/Sr2+/Sc3+) are 20%, 7.1% and 38.2%, respectively, among them, the possibility of Sc3+ being replaced is very small and can be ignored. And Bi3+ occupies K and Sr sites in KScSrSi2O7.
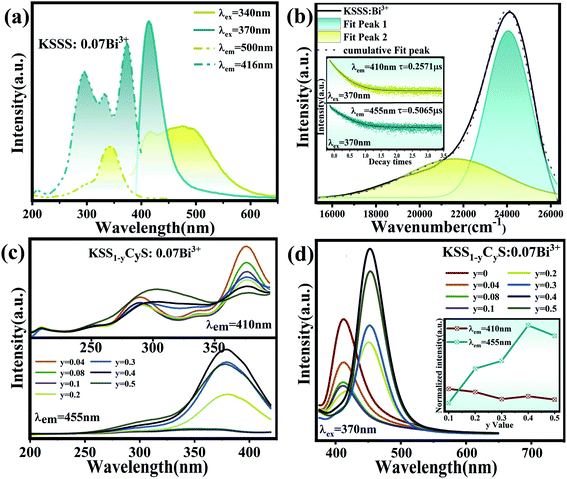 |
| Fig. 2 (a) The different PLE and PL spectra of KSSS:0.07Bi3+. (b) Gaussian fitting of KSSS:0.07Bi3+ emission spectra; the inset shows the average life of two sub-emission peaks (410 nm and 455 nm). (c) The PLE spectra of KSS1−yCyS:0.07Bi3+ under 410 nm and 455 nm different emission. (d) The PL spectra of KSS1−yCyS:0.07Bi3+ under 370 nm excitation; the inset shows the relative excitation intensity vs. various Ca2+ contents (y) at λem = 410 nm and λem = 455 nm. | |
Table 1 Ionic radii difference percentage (Dr) between matrix cations and doped Bi3+
Matrix cation (CN) |
Rm (Å) |
Doped ion (CN) |
Rd (Å) |
Dr (%) |
K+ (9) |
1.55 |
Bi3+ (9) |
1.24 |
20 |
Sr2+ (8) |
1.26 |
Bi3+ (8) |
1.17 |
7.1 |
Sc3+ (6) |
0.745 |
Bi3+ (6) |
1.03 |
38.2 |
According to the number of luminescence centers, the emission band of KSSS:0.07Bi3+ can be fitted to two sub-emission peaks, which are located at 410 nm and 455 nm, respectively, as shown in Fig. 2(b). The insets depicts the fluorescence lifetime of the two sub-emission peaks excited by 370 nm pulse laser (nano-LED), which both show second order exponential decay tendency as follows:21,22
|
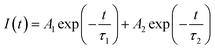 | (3) |
where
I(
t) is the intensity at
t, and
τ1 and
τ2 represent the short and long-decay components while
A1 and
A2 are fitting constants. The average lifetime
τ* can be calculated by the following
eqn (4):
20,22 |
τ* = (A1τ12 + A2τ22)/(A1τ1 + A2τ2)
| (4) |
The lifetimes of the two sub-emission peaks (410 nm and 460 nm) are 0.2571 μs and 0.5065 μs, respectively, which are consistent with the lifetimes of Bi3+. The difference in lifetime between different emission peaks confirms the existence of two possible emitting centers. In order to determine the origin of the asymmetric emission peaks, the crystallographic occupancy of ions in the matrix was calculated by using Van Uitert theoretical formula:23
|
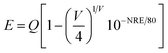 | (5) |
where
E represents the peak (cm
−1) of Bi
3+ ions,
Q is the free Bi
3+ ion at the lower energy edge position,
V and
E represent the valence and electron affinity of Bi
3+ ions, respectively.
N and
R represent the coordination number of the central cation and the radius of the matrix central cation replaced by the activated ion, respectively. Therefore, in the Van Uitert equation,
Q,
V and
E are all inherent attribute parameters of the matrix, hence the size of
E only depends on the product of
N and
R. For K and Sr, the value of
N ×
R is 13.95 and 10.08, respectively. Since the
E value is positively correlated with the
N ×
R, the origin of the two peaks can be qualitatively determined. According to the relation between
E value and
N ×
R, the two sub-peaks at 410 nm and 455 nm are generated by Bi
3+ ions occupying the K and Sr positions, respectively.
For further demonstrate the source of sub-emission peak, the following formula is used to calculate the distance of the centroid shift values of each emission center in KSSS:0.07Bi3+ and the crystal field splitting values and total shift values (D(A)) of Bi3+ ions at 6s6p level, as follows:24–27
|
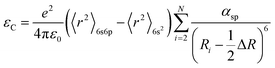 | (6) |
|
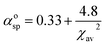 | (7) |
|
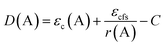 | (9) |
Formula (6) and (7), 〈r〉 stands for the radial radius of an electron in 6s6p configuration or 6s2 configuration, e is the elementary charge, and ε0 is the permittivity of vacuum. Thus, the value of
is constant. Ri stands for the distance between the center atom and the anion in the lattice. The summation is over all N anions that coordinate Bi3+. ½ΔR represents a correction for lattice relaxation around Bi3+, and ΔR for two kinds of cation and activate ion in KSSS radius difference. αosp is the spectroscopic polarizability of the anion, and its value is 2.82 × 10−30 m−3.χav is the electronegativity of the cation. In formula (8), βpoly is a constant that depends on the type of the coordination polyhedron. In this case, for KSSS:0.07Bi3+, βpoly = 1.35 × 109 pm2 cm−1. In formula (9), εc(A) is the centroid shift, εcfs(A) is the crystal field splitting. Finally, by formula (9), the total displacement value of energy level in KSSS:0.07Bi3+ shows D(K) < D(Sr), the related data are listed in Table 2. Combined with the previous analysis, it is verified again that the sub-emission peaks at 410 nm and 455 nm are derived from the emission of Bi3+ ions at K and Sr sites, respectively.
Table 2 The relative value of the energy level displacement in KSSS:0.07Bi3+
Occupying |
K+ |
Sr2+ |
av bond (R) |
2.890 |
2.690 |
ΔR |
0.031 |
0.009 |
εc(A) |
0.477 |
0.605 |
εcfs(A) |
2.001 |
2.309 |
D(A) |
D(K) < D(Sr) |
3.3 Controlling site-selection of Bi3+ via Ca2+ substitution for Sr2+
As is known to all, Bi3+ ion as a commonly used transition metal doped element, it's 6s and 6p electrons are in the outermost layer of the electron shell, which makes its luminescence properties are easily affected by the surrounding environment.28,29 As a consequence, Ca2+ ions are introduced to regulate the local lattice and improve the luminescence properties. Fig. 2(c) shows the excitation spectra of KSS1−yCyS:0.07Bi3+. When monitored at 410 nm, the resulting PLE spectrum contained two excitation band peaks at 370 nm (in the 350–420 nm range) and 290 nm (in the 200–350 nm range), and when monitored at 455 nm, the resulting PLE spectrum contains one excitation band peaks at 370 nm (in the range of 300–420 nm), which could be attributed to the electronic transitions from the ground state (1S0) to the excited state (3P1) of Bi3+ ions. With the concentration of Ca2+ (y) increasing, the emission intensities at 410 nm gradually decrease, while the emission intensities of 455 nm gradually increase and reach its maximum value when y = 0.4, and finally the concentration quenching occurs, as shown in Fig. 2(d). When Ca2+ concentration increased, it is speculated that Bi3+ ions may be transferred from one luminescence center to another in KSS1−yCyS crystals. The relationship between the emission intensity (fitting intensity at 410 nm and at 455 nm) and Ca2+ concentration is plotted in the inset of Fig. 2(d). The trend of monotonic decrease of emission intensity at 410 nm is in sharp contrast to that at 455 nm, which indicates that the occupation of Bi3+ transfers from one crystallization position to another in KSS1−yCyS.
In the above study, the occupying position of Bi3+ in the host was confirmed, that is, the 410 nm emission peak comes from the K site occupied by Bi3+ ions, and the 455 nm emission peak originates from the Sr site occupied by Bi3+ ions. Since the luminescence properties of Bi3+ are easily influenced by the surrounding crystal field environment, it is necessary to investigate the structural changes caused by the incorporation of Ca2+ ions. The main reason for the structural change is that Ca2+ has a smaller ionic radius compared with Sr2+. Firstly, at the Sr2+ site, Ca2+ with small radius cannot completely coordinate with 8O2− ions, leading to Ca2+ ions moving towards the surface and the gap of SrO8 polyhedrons and coordinating with 3O2− ions to form an isosceles triangular plane. Secondly, the addition of Ca2+ increases the distance between the cations, providing space to accommodate more activated ions. Finally, the introduction of small Ca2+ ions not only change the local environment of the lattice but also promote the mobility of the activated ions. Importantly, we used the modification of the SrO8 polyhedron to stimulate the sensitivity of the activated ion Bi3+ to its surrounding crystal field environment, leading to the redistribution of Bi3+ at two different cation positions. In order to understand the redistribution of Bi3+ ions more accurately, KSS1−yCyS:0.07Bi3+ was refined with GASA software. Similarly, KSS1−yCyS:0.07Bi3+ were refined with GSAS software, and the refining results were shown in Fig. S3.† All parameters met the experimental requirements. When the small radius of Ca2+ (r = 1.12 Å, CN = 8) is used to replace the large radius of Sr2+ (r = 1.26 Å, CN = 8) in the crystal, the cell should shrink and the cell parameters decrease. Fig. 3(a) shows the changes of cell volume V and cell parameters a, b and c with Ca2+ concentration y increasing, respectively. Curiously, when y increased from 0 to 0.2, both cell parameters and cell volume showed a decreasing trend, but when the replacement concentration was greater than 0.2, each parameter presented an increasing trend. The changes of polyhedron SrO8 are shown in the illustration. Obviously, the volume of polyhedron SrO8 also shows a trend of first increase and then decrease, which is due to the gradual entry of Bi3+ (r = 1.17 Å, CN = 8) ions with a larger radius into the larger Sr site. In other words, with the gradual substitution of Ca2+ for Sr2+ ions, Bi3+ ions gradually transferred from the K to the Sr site. The schematic diagram of the redistribution of Bi3+ in Sr and K sites are presented in Fig. 3(b). When y < 0.2, since the radius of Ca2+ is smaller than that of Sr2+, and the occupying transfer of Bi3+ ion is in the initial stage, the contraction degree of the crystal cell is greater than the expansion degree of the crystal cell, as a result all the parameters of the crystal cell show a state of decrease. When the substitution concentration y > 0.2, the expansion caused by the entry of Bi3+ ions with large radius into the Sr site is greater than the lattice contraction caused by the replacement of Sr2+ ions with Ca2+, hence the crystal cell shows the state of expansion.
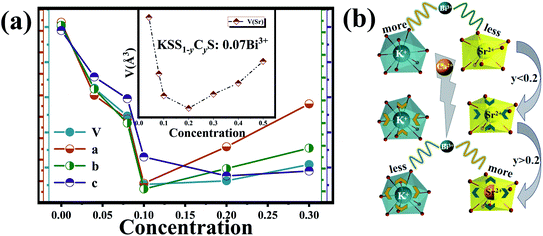 |
| Fig. 3 (a) The changes of cell parameters (V, a, b, c) of KSS1−yCyS:0.07Bi3+ with y increasing; the inset presents the variation of the volume of the SrO8 polyhedron. (b) Schematic diagram of the redistribution of Bi3+ in Sr and K sites with y increasing. | |
According to the above analysis, the emission peaks at 410 nm and 455 nm are originated from Bi3+ which occupied the K site and Sr site, respectively. Therefore, the migration of Bi3+ ions well explains the change of PL spectrum in Fig. 2(d). As more Bi3+ ions transfer from K site to Sr site, the intensity of 455 nm emission increases continuously, while that of 410 nm decreases gradually. When most of Bi3+ ions enter the Sr site, the emission intensity will not increase with the increase of Ca2+ concentration.
Generally, the luminescence of Bi3+ ion has a large absorption cross section and a wide emission due to its s–p transition.30 Surprisingly, the emission spectra of KSS0.6C0.4S:0.07Bi3+ shows a narrow-band blue-emission under the excitation of 370 nm, the FWHM is only 40 nm, as shown in Fig. 4(a). Generally, the coordination symmetry and rigidity of the crystal structure are the main factors affect the half-peak width of the emission spectrum, the highly symmetric and rigid structure are more likely to have a narrow emission peak. Conversely, the lower the symmetry of crystal structure, the wider the half-peak width.31–33 For a polyhedron, the symmetry of the polyhedron is usually characterized by the dispersion degree of the side length. The smaller the dispersion degree of the side length, the higher the symmetry of the polyhedron. The crystal distortion can be expressed by using the set of following equations:34–36
|
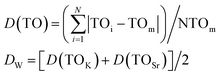 | (10) |
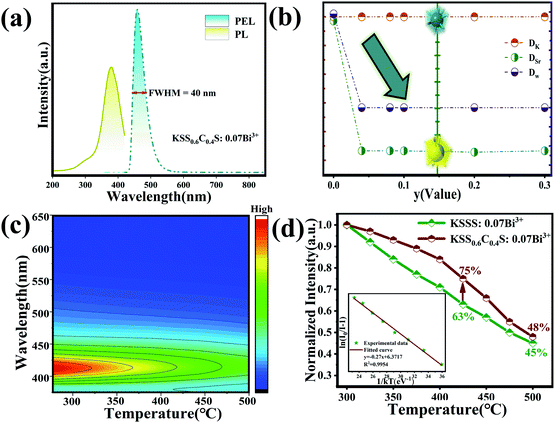 |
| Fig. 4 (a) The emission spectrum of KSS0.6C0.4S:0.07Bi3+ (λex = 370 nm). (b) Variation of the distortion degree of KSS1−yCyS:0.07Bi3+ samples. (c) Temperature-dependent emission spectra of KSS0.6C0.4S:0.07Bi3+ phosphors under 370 nm excitation. (d) The temperature-dependent normalized integrated emission intensities of KSSS:0.07Bi3+ and KSS0.6C0.4S:0.07Bi3+; The inset shows the functional relationship of ln[(I0/IT) − 1] versus 1/kT of KSS0.6C0.4S:0.07Bi3+. | |
Among them, TOi is the distances between cations and individual ligand anions, TOm is the average bond length. The distortion D(TO) is the average deviation of the bond length. Dw is the degree of distortion of the entire crystal, D(TOK) and D(TOSr) refer to the torsion resistance of polyhedra KO9 and SrO8, respectively, and the results are listed in Table 3. Obviously, when Sr2+ ions are replaced by Ca2+ ions, the distortion degree of SrO8 polyhedron in KSS1−yCyS:0.07Bi3+ crystal is significantly reduced (As shown in Fig. 4(b)), which indicates that the introduction of Ca2+ ions greatly improved the structural rigidity of Sr polyhedron, enhanced the overall symmetry of crystal cells and caused the redistribution of Bi3+ ions on the occupied space. With the increase of Ca2+ content, the number of Bi3+ entering the Sr site increases, while the number of Bi3+ at the K site decreases gradually, thus realizing the transfer of the main emission peak from 410 nm to 455 nm. The enhancement of structural rigidity and symmetry of the crystal makes the half-peak width of the emission peak significantly narrower.
Table 3 Variation of the distortion degree of KSS1−yCyS:0.07Bi3+ samples
|
y = 0 |
y = 0.04 |
y = 0.08 |
y = 0.10 |
y = 0.20 |
DK |
0.112 |
0.112 |
0.111 |
0.112 |
0.112 |
DSr |
0.166 |
0.068 |
0.068 |
0.067 |
0.068 |
DW |
0.139 |
0.090 |
0.090 |
0.090 |
0.090 |
Debye temperatures for crystallographically distinct atoms (θD,i) were calculated with isotropic atomic displacement parameters using the high temperature approximation:37,38
|
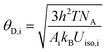 | (11) |
where i represents the atomic species K or Sr, and
Ai is the atomic weight of the atom. The
Uiso,i values employed were the average of the individual atomic species in the unit cell, weighted by their Wyckoff multiplicities. Then, according to the stoichiometric coefficients of each atom in the formula KSS
1−yC
yS, the Debye temperature is roughly calculated. It can be seen from the formula that Debye temperature is inversely proportional to atomic displacement parameter (
Uiso). Relevant parameters can be obtained by using the previous refinement data. The corresponding
Uiso of K site and Sr site before and after Ca replacement is 0.0397, 0.0419 and 0.0242, 0.0121, respectively. Relevant data are listed in Table S1.
† Obviously, when Ca ions replaces Sr
2+ ions, the average atomic displacement of K site and Sr site decreases, and the reduction of Sr site is particularly significant. The smaller
Uiso corresponds to the higher Debye temperature. Furthermore, it is proved that when Bi
3+ ions is transferred from K site to Sr site, the local environment of around Bi
3+ ions becomes more symmetric and more rigid, which further proves the cause of narrow-band emission after replacement.
Generally, the enhancement of crystal structure rigidity tends to improve the stability of phosphors to a certain extent. To further verify that the introduction of Ca ions increases the structural rigidity of the overall lattice, we measured the temperature spectra of KSSS:0.07Bi3+ and KSS0.6C0.4S:0.07Bi3+. Fig. 4(c) shows the temperature dependent PL spectra of representative KSS0.6C0.4S:0.07Bi3+ measured from 300 K to 500 K with a step of 25 K, and the temperature dependent PL spectra of KSSS:0.07Bi3+ is shown in Fig. S4.† It is observed that the emission intensity gradually decreases with increasing temperatures due to thermal quenching. The temperature stability of phosphor after Ca2+ substitution increased by 12% at a high temperature of 423 K shown in Fig. 4(c). The PL intensity can be fitted by the Arrhenius equation:39,40
|
ln(I0/I) = ln A − Ea/kT
| (12) |
where,
I0 and
I are emission intensities of the sample at 298 K and other test temperatures, respectively.
A is a constant,
k is Boltzmann's constant,
T is the thermodynamic temperature. When taking 1/
kT as the
x-coordinate and ln(
I0/
I) as the
y-coordinate, linear fitting was performed on the obtained data. The slope of the fitted line is the activation energy
Ea. As is shown in an illustrated of
Fig. 4(d), the activation energy
Ea of the activated ions in KSSS:0.07Bi
3+ and KSS
0.6C
0.4S:0.07Bi
3+ were 0.203 eV and 0.270 eV respectively, which indicated that the phosphors with Ca
2+ introduction had better thermal stability. It is also further proved that the rigidity of the structure is improved after the introduction of Ca ions, which creates a high rigidity environment for the activated ions.
The luminescence properties of phosphors (e.g. peak emission wavelength and full-width at half-maximum (FWHM)) directly affect the color gamut space.41 As a result, phosphors with narrow half-peak width tend to produce higher color purity, which will be more suitable for high-power pc-LEDs and wide-color backlight applications.36 The color purity of the phosphor can be defined by the percentage of the chromaticity coordinates of the measured light source and the linear distance between the white light source, which can be calculated by the following formula (13):42,43
|
 | (13) |
where (
x,
y) represents the color coordinates of phosphor. C(
xC,
yC) represents the white light source whose CIE color coordinate is (0.3333, 0.3333), as shown in
Fig. 5(a), D(
xD,
yD) is the color coordinate of the main wavelength corresponding to the measured light source. It connects the measured light source S
1 (KSSS:0.07Bi
3+) with white light source C and extends the intersection with the spectral trajectory line at point D. Main wavelength of 465 nm, the comparison wavelength and CIE1931 color coordinates table (
xD,
yD) for (0.1355, 0.0399), S
2 is the color coordinates of KSSCS:0.07Bi
3+. As a result, the color purity of KSSS:0.07Bi
3+ and KSS
0.6C
0.4S:0.07Bi
3+ are 78% and 88%, respectively. Obviously, the KSS
0.6C
0.4S:0.07Bi
3+ phosphors showed better color purity after controlling the cationic component, which indicates that the color purity of the phosphor has been greatly improved by the substitution of Ca
2+ for Sr
2+.
Table 4 presents the comparison of luminescent specialties between some phosphors reported previously and KSS
0.6C
0.4S:0.07Bi
3+. It is obvious that the color purity of KSS
0.6C
0.4S:0.07Bi
3+ is at a medium level. However, comprehensively comparing data such as excitation and emission wavelength, FWHM, color purity and thermal activation energy, it can be found that the performance of KSS
0.6C
0.4S:0.07Bi
3+ is at an advantage.
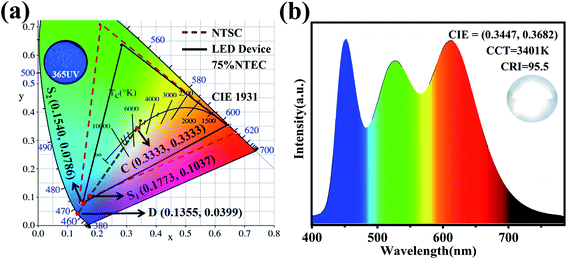 |
| Fig. 5 (a) CIE 1931 color coordinates of main wavelength positions of KSSS:0.07Bi3+ and KSS0.6C0.4S:0.07Bi3+, color space of NTSC standard (red dotted line) and white LED device (black line), and luminescence photograph of KSS0.6C0.4S:0.07Bi3+ phosphors under 365 nm UV lamp. (b) The EL spectrum of LED device fabricated with KSS0.6C0.4S:0.07Bi3+ and the commercial phosphors on an UV LED chip (370 nm) under a voltage of 3.2 V and current of 5 mA. | |
Table 4 Summary of excitation wavelength, emission wavelength, FWHM, color purity and activation energy (ΔE) of KSS0.6C0.4S:0.07Bi3+, some blue narrow-band phosphors and Bi3+ doped phosphors previously reported
Samples |
λex (nm) |
λem (nm) |
FWHM (nm) |
Color purity (%) |
ΔE (eV) |
Ref. |
Na3Sc2(PO4)3:Eu2+ |
370 |
458 |
50 |
89 |
— |
44 |
Ba9Lu1.5Al0.5Si6O24:Ce3+ |
400 |
488 |
117 |
— |
0.3000 |
45 |
NaHfSi2O7:Eu2+ |
397 |
460 |
54 |
90 |
0.1394 |
46 |
RbNa3(Li3SiO4)4:Eu2+ |
400 |
471 |
22.4 |
83.3 |
— |
47 |
Ba5SiO4Cl6:Eu2+ |
345 |
440 |
32 |
93 |
0.1425 |
48 |
BaMgAl10O17:Eu2+ |
350 |
450 |
52 |
91.3 |
— |
49 |
Sr4OCl6:Eu2+ |
370 |
446 |
46 |
— |
0.1378 |
50 |
Ca5(BO3)3F:Bi3+ |
322 |
459 |
100 |
— |
— |
51 |
Cs2AgInCl6:Bi3+ |
380 |
560 |
160 |
— |
— |
52 |
KScSr0.6Ca0.4Si2O7:Bi3+ |
370 |
455 |
40 |
88 |
0.2700 |
— |
3.4 Applications in white LED backlight display
The wide excitation, narrow emission, and excellent thermal stability make KSS0.6C0.4S:0.07Bi3+ a potential candidate for backlight display applications. A white LED was fabricated with KSS0.6C0.4S:0.07Bi3+ and the commercial green and red phosphor on an UV (370 nm) chip under a voltage of 3.2 V and current of 5 mA, whose EL spectrum and photograph are shown in Fig. 5(b). It can be seen a warm LED with low CCT = 3401 K, CRI = 95.5, and CIE color coordinate of (0.3447, 0.3682) has been obtained. For a comparison, the EL spectrum and photograph of the commercial blue phosphor BaMgAl10O17:Eu2+combined with UV (370 nm) chip are given in Fig. S5.† It is noticeable that the CCT of LED with KSS0.6C0.4S:0.07Bi3+ (3401 K) is lower than that of BaMgAl10O17:Eu2+ (5064 K), CRI up to 95.5% and CIE color coordinate of (0.4165, 0.4074) had more application potential. The color gamut of tour white LED device can cover the color space of 75% NTSC in CIE 1931 (as shown in Fig. 5(a)), which belongs to the broad gamut category. All these results indicate that KSSCS:0.07Bi3+ is a promising narrow-band blue phosphor for display backlight.
4. Conclusions
A series of blue narrow-band phosphors KScSr1−yCaySi2O7:0.07Bi3+ were obtained by introducing Ca2+ ions into KScSrSi2O7:Bi3+. With Ca2+ concentration increasing, the original crystal field environment changed, which lead to the migration of Bi3+ from K site to Sr site. Consequently, the main emission peak wavelength changed from 410 nm to 455 nm, and the luminescence color turned from purple to blue. Importantly, the distortion of the polyhedron in the crystal was reduced, the rigidity of the crystal structure was increased, and the symmetry was improved. As a result, under the excitation of 370 nm, KScSr0.6Ca0.4Si2O7:0.07Bi3+ phosphor presented a blue narrow band emission with a FWHM of 40 nm, and the color purity increased from the original 78% to 88%. And moreover, the LED device using KScSr0.6Ca0.4Si2O7:0.07Bi3+ phosphor performs a low CCT of 3401 K and a Ra of 95.5. The results demonstrate that the blue narrow-band phosphor KScSr0.6Ca0.4Si2O7:0.07Bi3+ has a bright prospect for application in pc-LEDs.
Conflicts of interest
The authors declare that they have no conflicts of interest.
Acknowledgements
The work is supported by the National Natural Science Foundation of China (No. 51672066, 51902080), the Funds for Distinguished Young Scientists of Hebei Province, China (No. A2018201101), and the Natural Science Foundation of Hebei Province, China (No. E2019201223), the personnel training project of Hebei Province, China (No. A201902005).
References
- A. Tücks, A. S. Wochnik, A. K. Henß, C. Scheu, C. Hecht, D. Wiechert, P. J. Schmidt, P. Pust, V. Weiler and W. Schnick, Narrow-band red-emitting Sr[LiAl3N4]:Eu2+ as a next-generation LED-phosphor material, Nat. Mater., 2014, 13, 891–896 CrossRef PubMed.
- P. Pust, P. J. Schmidt and W. Schnick, A revolution in lighting, Nat. Mater., 2015, 14, 454–458 CrossRef CAS PubMed.
- Z. G. Xia and Q. L. Liu, Progress in discovery and structural design of color conversion phosphors for LEDs, Prog. Mater. Sci., 2016, 84, 59–117 CrossRef CAS.
- B. Wang, H. Lin, F. Huang, J. Xu, H. Chen, Z. Lin and Y. Wang, Non-Rare-Earth BaMgAl10−2xO17:xMn4+, xMg2+: A Narrow-Band Red Phosphor for Use as a High-Power Warm w-LED, Chem. Mater., 2016, 28, 3515–3524 CrossRef CAS.
- J. S. Steckel, J. Ho, C. Hamilton, J. Xi, C. Breen, W. Liu, P. Allen and S. Coe-Sullivan, Ultra-Bright, Highly Efficient, Low Roll-Off Inverted Quantum-Dot Light Emitting Devices (QLEDs), J. Soc. Inf. Disp., 2015, 23, 294–305 CrossRef CAS.
- L. Wang, X. Wang, T. Kohsei, K. Yoshimura, M. Izumi, N. Hirosaki and R. J. Xie, High-Uniformity Planar Mini-Chip-Scale Packaged LEDs with Quantum Dot Converter for White Light Source, Opt. Express, 2015, 23, 28707–28717 CrossRef CAS PubMed.
- G. J. Hoerder, M. Seibald, D. Baumann, T. Schroder, S. Peschke, P. C. Schmid, T. Tyborski, P. Pust, I. Stoll, M. Bergler, C. Patzig, S. Reissaus, M. Krause, L. Berthold, T. Hoche, D. Johrendt and H. Huppertz, Sr[Li2Al2O2N2]:Eu2+ A High Performance Red Phosphor to Brighten the Future, Nat. Commun., 2019, 10, 1824 CrossRef PubMed.
- D. Dutzler, M. Seibald, D. Baumann and H. Huppertz, Alkali Lithosilicates: Renaissance of a Reputable Substance Class with Surprising Luminescence Properties, Angew. Chem., Int. Ed., 2018, 57, 13676–13680 CrossRef CAS PubMed.
- M. Zhao, H. X. Liao, L. X. Ning, Q. Y. Zhang, Q. L. Liu and Z. G. Xia, Next-Generation Narrow-Band Green-Emitting RbLi(Li3SiO4)2:Eu2+ Phosphor for Backlight Display Application, Adv. Mater., 2018, 30, 1802489 CrossRef PubMed.
- D. Dutzler, M. Seibald, D. Baumann and H. Huppertz, Renaissance of a Reputable Substance Class with Surprising Luminescence Properties, Angew. Chem., Int. Ed., 2018, 57, 13676 CrossRef CAS PubMed.
- L. L. Wang, Q. Sun, Q. Z. Liu and J. S. Shi, Investigation and application of quantitative relationship between sp energy levels of Bi3+ ion and host lattice, J. Solid State Chem., 2012, 191, 142–146 CrossRef CAS.
- M. Amer and P. Boutinaud, On the character of the optical transitions in closed-shell transition metal oxides doped with Bi3+, Phys. Chem. Chem. Phys., 2013, 19(3), 2591–2596 RSC.
- F. W. Kang, G. H. Sun, P. Boutinaud, F. Gao, Z. H. Wang, J. Lu, Y. Y. Li and S. S. Xiao, Tuning the Bi3+ photo-emission color over the entire visible region by manipulating secondary cations modulation in the ScVxP1−xO4:Bi3+(0 ≤ x≤ 1) solid solution, J. Mater. Chem. C, 2019, 7(32), 9865–9877 RSC.
- F. W. Kang, M. Y. Peng, S. H. Xu, Z. J. Ma, G. P. Dong and J. R. Qiu, Broadly Tunable Emission from CaMoO4:Bi Phosphor Based on Locally Modifying the Micro environment Around Bi3+ Ions, Inorg. Chem., 2014, 1373–1380 CAS.
- F. W. Kang, H. S. Zhang, W. Lothar, X. B. Yang, Y. Zhang, D. Y. Lei and M. Y. Peng, Band-Gap Modulation in Single Bi3+-Doped Yttrium-Scandium-Niobium Vana dates for Color Tuning over the Whole Visible Spectrum, Chem. Mater., 2016, 28, 2692–2703 CrossRef CAS.
- S. Ray, P. Tadge, S. Dutta, T. M. Chen, B. N. Govind and S. J. Dhoble, Luminescence and application of BaKYSi2O7:Eu2+: A new blue-emitting phosphor for near-UV white-light LED, Ceram. Int., 2018, 44, 8334–8343 CrossRef CAS.
- Y. Wang, J. Ding and Y. Wang, Preparation and photo luminescence properties with the site-selected excitation of Bi3+ activated Ba3Sc4O9 phosphors, J. Am. Ceram. Soc., 2017, 100, 2612–2620 CrossRef CAS.
- B. Philippe, On the spectroscopy of Bi3+ in d10 post-transition metal oxides, J. Lumin., 2020, 223, 117219 CrossRef.
- P. Chen, F. Mo, A. Guan, R. Wang, G. Wang, S. Xia and L. Zhou, Luminescence and energy transfer of the color-tunable phosphor Li6Gd(BO3)3: Tb3+, Bi3+, Eu3+, Appl. Radiat. Isot., 2016, 108, 148–153 CrossRef CAS PubMed.
- X. Zhang, J. Xu, Z. Guo and M. Gong, Luminescence and energy transfer of dual-emitting solid solution phosphors (Ca, Sr)10Li(PO4)7:Ce3+, Mn2+ for ratiometric temperature sensing, Ind. Eng. Chem. Res., 2017, 56, 890–898 CrossRef CAS.
- M. Li, J. Zhang, J. Han, Z. Qiu, W. Zhou, L. Yu, S. Lian and Z. Li, Changing Ce3+ Content and Codoping Mn2+ Induced Tunable Emission and Energy Transfer in Ca2.5Sr0.5 Al2O6: Ce3+, Mn2+, Inorg. Chem., 2017, 56, 241–251 CrossRef CAS PubMed.
- K. Li, H. Lian, M. Shang and J. Lin, A novel greenish yellow-orange red Ba3Y4O9:Bi3+, Eu3+ phosphor with efficient energy transfer for UV-LEDs, Dalton Trans., 2015, 44, 2054–20550 Search PubMed.
- L. G. Van Uitert, An empirical relation fitting the position in energy of the lower d band edge for Eu2+ or Ce3+ in various compounds, J. Lumin., 1984, 9, 1 CrossRef.
- P. Dorenbos, 5d-level energies of Ce3+ and the crystalline environment. III. Oxides containing ionic complexes, Phys. Rev. B: Condens. Matter Mater. Phys., 2001, 64, 125117 CrossRef.
- F. Wang, W. Wang and L. Zhang, et al. Luminescence properties and its red shift of blue-emitting phosphor Na3YSi3O9:Ce3+ for UV LED, RSC Adv., 2017, 7, 27422–27430 RSC.
- P. Dorenbos, Calculation of the energy of the 5d barycenter of La3F3[Si3O9]:Ce3+, J. Lumin., 2003, 105, 117–119 CrossRef CAS.
- P. Dorenbos, 5d-level energies of Ce3+ and the crystalline environment. I. Fluoride compounds, Phys. Rev. B: Condens. Matter Mater. Phys., 2000, 62, 15640–15649 CrossRef CAS.
- F. Kang, M. Peng, S. Xu, Z. Ma, G. Dong and J. Qiu, Broadly tunable emission from CaMoO4:Bi3+ phosphor based on locally modifying the micro environment around Bi3+ ions, Eur. J. Inorg. Chem., 2014, 1373–1380 CrossRef CAS.
- F. Kang, M. Peng, X. Yang, G. Dong, G. Nie, W. Liang, S. Xu and J. Qiu, Broadly tuning Bi3+ emission via crystal field modulation in solid solution compounds (Y, Lu, Sc)VO4:Bi3+ for ultraviolet converted white LEDs, J. Mater. Chem. C, 2014, 2, 6068–6076 RSC.
- H. Zhu, Z. Xia and H. Liu, Luminescence properties and energy transfer of Bi3+/Eu2+- codoped Ca10(PO4)6F2 phosphors, Mater. Res. Bull., 2013, 48(9), 3513–3517 CrossRef CAS.
- M. Zhao, Y. Zhou, M. S. Molokeev, Q. Zhang, Q. Liu and Z. G. Xia, Discovery of New Narrow-Band Phosphors with the UCr4C4-Related Type Structure by Alkali Cation Effect, Adv. Opt. Mater., 2019, 7, 1801631 CrossRef.
- Q. Wei, X. Zhou and Z. Tang, A novel blue-emitting Eu2+-doped Chlorine silicate phosphor with narrow-band for illumination and displays: structure and luminescence properties, CrystEngComm, 2019, 21, 3660–3667 RSC.
- C. Li, X. Wang and F. Chi, Narrow-Band Blue Emitting Phosphor Ca8Mg7Si9N22:Eu2+ for White pc-LEDs, J. Mater. Chem. C, 2019, 7, 3730–3734 RSC.
- J. Ruan, R. J. Xie and N. Hirosaki, Nitrogen gas pressure synthesis and photo luminescent properties of orange-red SrAlSi4N7:Eu2+ phosphors for white light-emitting diodes, J. Am. Ceram. Soc., 2011, 94(2), 536–542 CrossRef CAS.
- Z. G. Xia, S. Mia and M. Chen, Crystallographic Sites, and Tunable Luminescence Properties of Eu2+ and Ce3+/Li+-Activated Ca1.65Sr0.35SiO4 Phosphors, Inorg. Chem., 2015, 54(16), 7684–7691 CrossRef CAS PubMed.
- P. Dai, X. Zhang and L. Bian, Color tuning of (K1−xNax)SrPO4: 0.005Eu2+, yTb3+ blue-emitting phosphors via crystal field modulation and energy transfer, J. Mater. Chem. C, 2013, 1(2), 4570–4576 RSC.
- N. C. George, A. Birkel and J. Brgoch, Average and Local Structural Origins of the Optical Properties of the Nitride Phosphor La3−xCexSi6N11(0< x ≤ 3), Inorg. Chem., 2013, 52(23), 1373–13741 CrossRef PubMed.
- N. George, A. Pell and G. Dantelle, Local Environments of Dilute Activator Ions in the Solid-State Lighting Phosphor Y3−xCexAl5O12, ChemInform, 2014, 45(2), 3979–3995 CrossRef.
- J. Y. Han, I. W. Bin and D. Kim, New full-color-emitting phosphor, Eu2+-doped Na2−xAl2−xSixO4 (0 ≤ x ≤ 1) obtained using phase transitions for solid-state white lighting, J. Mater. Chem., 2012, 22(12), 5374–5381 RSC.
- J. Ruan, R. J. Xie and N. Hirosaki, Nitrogen gas pressure synthesis and photo luminescent properties of orange-red SrAlSi4N7:Eu2+ phosphors for white light-emitting diodes, J. Am. Ceram. Soc., 2011, 94(2), 536–542 CrossRef CAS.
- P. Strobel, S. Schmiechen, M. Siegert, A. Tücks, P. J. Schmidt and W. Schnick, Narrow-Band Green Emitting Nitridolithoalumosilicate Ba[Li2(Al2Si2)N6]:Eu2+ with Framework Topology for LED/LCD Back lighting Applications, Chem. Mater., 2015, 27, 6109–6115 CrossRef CAS.
- P. Du and J. S. Yu, Photo luminescence and Cathodoluminescence Properties of Eu3+ Ions Activated AMoO4 (A = Mg, Ca, Sr, Ba) Phosphors, Mater. Res. Bull., 2015, 70, 553–558 CrossRef CAS.
- Y. F. Wu, Y. T. Nien, Y. J. Wang, I. G. Chen and J. McKittrick, Enhancement of Photo luminescence and Color Purity of CaTi3:Eu3+ Phosphor by Li Doping, J. Am. Ceram. Soc., 2012, 95, 1360–1366 CrossRef CAS.
- X. Wang, Z. Zhao, Q. Wu, C. Wang, Q. L. Wang, Y. Yan and Y. Wang, Structure, photo luminescence and abnormal thermal quenching behavior of Eu2+-doped Na3Sc2(PO4)3: a novel blue-emitting phosphor for n-UV LEDs, J. Mater. Chem. C, 2016, 4, 8795–8801 RSC.
- L. Wang, C. Yuan, P. Hu, R. Li, Y. Liu, P. Sun, R. Dong, X. Qi, J. Jiang and H. Jiang, A low cost and high efficient Ba9(Lu2−x−yAlx)Si6O24:yCe3+ cyan-emitting phosphor, Ceram. Int., 2020, 46, 11466–11473 CrossRef CAS.
- Q. Wei, J. Ding and Y. Wang, A novel wide-excitation and narrow-band blue-emitting phosphor with hafnium silicon multiple rings structure for photo luminescence and cathodoluminescence, J. Alloys Compd., 2020, 831, 154825 CrossRef CAS.
- H. Liao, M. Zhao, M. S. Molokeev, Q. Liu and Z. G. Xia, Learning from a Mineral Structure toward an Ultra-Narrow-Band Blue-Emitting Silicate Phosphor RbNa3(Li3SiO4)4:Eu2+, Angew. Chem., Int. Ed. Engl., 2018, 57, 11728–11731 CrossRef CAS PubMed.
- Q. Wei, X. Zhou, Z. Tang, X. Wang and Y. Wang, A novel blue-emitting Eu2+-doped Chlorine silicate phosphor with narrow-band for illumination and displays: structure and luminescence properties, CrystEngComm, 2019, 21, 3660–3667 RSC.
- S. Kim and Y. Kim, Investigation of the degradation mechanisms in BaMgAl10O17:Eu2+ phosphor: on the influence of thermal process on operational durability, Appl. Phys. B, 2010, 98, 429–434 CrossRef.
- S. J. Gwak, P. Arunkumar and W. B. Im, A New Blue-Emitting Oxohalide Phosphor Sr4OCl6:Eu2+ for Thermally Stable, Efficient White-Light-Emitting Devices under Near-UV, J. Phys. Chem. C, 2014, 118, 2686–2692 CrossRef CAS.
- X. Li, P. L Li, Z. J. Wang, S. M. Liu, Q. Bao, X. Y. Meng and K. L. Qiu, Color-Tunable Luminescence Properties of Bi3+ in Ca5(BO3)3F via Changing Site Occupation and Energy Transfer, Chem. Mater., 2017, 29, 8792–8803 CrossRef CAS.
- M. B. Gray, J. D. Majher, T. A. Strom and P. M. Woodward, Broadband White Emission in Cs2AgIn1−xBixCl6 Phosphors, J. Inorg. Chem., 2019, 58, 13403–13410 CrossRef CAS PubMed.
Footnote |
† Electronic supplementary information (ESI) available: Table S1 and Fig. S1–S5. See DOI: 10.1039/d1ra01375k |
|
This journal is © The Royal Society of Chemistry 2021 |
Click here to see how this site uses Cookies. View our privacy policy here.