DOI:
10.1039/D1RA01365C
(Paper)
RSC Adv., 2021,
11, 18974-18983
Polysaccharide from rubescens: extraction, optimization, characterization and antioxidant activities
Received
19th February 2021
, Accepted 10th May 2021
First published on 25th May 2021
Abstract
In this work, rubescens polysaccharide was extracted and extraction conditions were optimized with the response surface method (RSM). The extracted polysaccharide was structurally elucidated and its antioxidant activity was investigated. The best extraction conditions were as follows: the solvent-solid ratio was 33.33
:
1, the extraction temperature was 87.70 °C and the extraction time was 2.59 h. Under optimum conditions, the predicted yield of the polysaccharide was 11.92%. The preliminary structural characteristics were analyzed by HPLC, X-ray, and FT-IR. Results showed that the polysaccharide composition was mannose
:
glucose
:
galactose
:
xylose
:
arabinose = 2.27
:
4.65
:
2.57
:
1
:
1.85. In addition, the rubescens polysaccharide possessed typical characteristic absorption peaks of polysaccharides. During the antioxidant experiments, the rubescens polysaccharide showed great reducing capacity and strong antioxidant activities on DPPH, ABTS, and hydroxyl radicals. This proved that rubescens polysaccharide has the potential to be a resource for the development of natural antioxidants. Rubescens has become a research object highly valued by the international oncology community and by western institutions for the research and development of new drugs due to its excellent anti-tumor activity. The research on the extraction of polysaccharide from rubescens provides a technical reference for the further utilization of rubescens resources. Hot-water extraction has the advantages of simple operation, easy access to materials and mild extraction conditions, and it can better preserve the original biological activity of the polysaccharide. The research on the antioxidant activity of rubescens polysaccharide provides theoretical support for its use as a natural antioxidant.
1. Introduction
Rubescens, also known as bingling grass, is a famous traditional medicine. Modern research shows that rubescens has efficacy in clearing heat and detoxicating, reducing inflammation, promoting blood circulation and dissipating swelling, and anti-tumor activity.1,2 Jiyuan has rich resources and unique population of rubescens. As early as 2006, Jiyuan rubescens was identified as a geographical indication protected product. Rubescens contains oridonin, ponicidin, flavonoids, and polysaccharides. Modern pharmacological and toxicological studies have proved that rubescens polysaccharide (RP) has many pharmacological activities, such as immune regulation and anti-tumor activity.3,4 However, the current research on rubescens is mostly focused on its fat-soluble components, such as diterpenoids,5–7 and there are few studies on RP.
Currently, there are many methods of extracting polysaccharides, such as heating, enzyme or ultrasonic-assisted methods. However, as is known, solvents are not environmentally friendly and ultrasonic or enzyme-assisted extraction is uneconomical, which limits the usage of these techniques. Hot-water extraction has the advantages of simple operation, easy access to materials and mild extraction conditions, and it can better preserve the original biological activity of polysaccharides.8,9 Therefore, the purpose of this work was to apply response surface methodology (RSM) to optimize the extraction conditions of RP. RSM has been used to optimize extraction processes of many natural active ingredients in a wide range of fields, such as pharmaceutical and food industries.10–12 Box–Behnken design (BBD) is one type of RSM; it is widely used because it is effective, and easy to arrange and explain experiments.13 Therefore, we chose the BBD method.
Plant polysaccharides have certain biological and antioxidant activities,14,15 and they have always been an active area of research. It is expected that polysaccharides can be applied to food and medicine to promote human health. Because polysaccharides have good antioxidant properties, they can be developed as natural antioxidants to prevent the occurrence of chronic diseases.16,17 Herein, we studied the antioxidant activity of RP from four aspects: reducing ability, DPPH radical scavenging ability, hydroxyl radical scavenging ability, and ABTS radical scavenging ability. It is hoped that the use of RP will provide some theoretical basis for the development of medicines and functional foods in the future.
2. Materials and methods
The rubescens sample was purchased from Henan Jiyuan Research Institute, crushed and screened with a 20 mesh screen to obtain fractions. All other chemicals used in the experiment were of analytical grade.
2.1 Extraction of crude polysaccharides
Rubescens (100 g) was extracted twice with petroleum ether at 80 °C for 2 h each time in order to remove some colored materials and lipids. After being dried at 60 °C for 12 h, the defatted sample (3 g) was extracted under the conditions of liquid–solid ratio (10–50), extraction time (1–5 h) and extraction temperature (60–100 °C). The filtrate was measured in a 250 mL volumetric flask. D-Glucose was used as the standard, and the content of polysaccharide was determined by the phenol-sulfuric acid method.18
2.2 Experimental design and statistical analysis
Based on single factor research, three factors, namely X1 (liquid–solid ratio, mL g−1), X2 (extraction temperature, °C) and X3 (extraction time, h) were studied to determine their influence on the polysaccharide yield. According to the BBD method, three levels were designed, namely X1 (20
:
1, 30
:
1, 40
:
1), X2 (70 °C, 80 °C, 90 °C) and X3 (1 h, 2 h, 3 h). The design of each factor and level is shown in Table 1; the whole design consisted of the group of 17 experiments in Table 2.
Table 1 Factors and level design in the Box–Behnken design
Factor |
Levels |
−1 |
0 |
1 |
Liquid/solid ratio (mL g−1) |
20 |
30 |
40 |
Extraction temperature (°C) |
70 |
80 |
90 |
Extraction time (h) |
1 |
2 |
3 |
Table 2 BBD with three variables and results for the yield of RP
Run order |
X1 (liquid–solid ratio, mL g−1) |
X2 (extraction temperature, °C) |
X3 (extraction time, h) |
Y (polysaccharide yield, %) |
Observed |
Predicted |
1 |
0 |
−1 |
−1 |
5.95 |
5.86 |
2 |
1 |
0 |
−1 |
6.39 |
6.66 |
3 |
0 |
1 |
−1 |
6.82 |
6.77 |
4 |
−1 |
−1 |
0 |
7.41 |
7.63 |
5 |
0 |
0 |
0 |
11.06 |
10.92 |
6 |
0 |
0 |
0 |
10.92 |
10.92 |
7 |
1 |
−1 |
0 |
7.85 |
7.67 |
8 |
1 |
0 |
1 |
9.89 |
10.02 |
9 |
0 |
0 |
0 |
10.92 |
10.92 |
10 |
−1 |
0 |
−1 |
5.66 |
5.53 |
11 |
−1 |
1 |
0 |
8.58 |
8.76 |
12 |
0 |
1 |
1 |
11.36 |
10.45 |
13 |
1 |
1 |
0 |
10.92 |
10.70 |
14 |
0 |
0 |
0 |
11.06 |
10.92 |
15 |
−1 |
0 |
1 |
9.46 |
9.19 |
16 |
0 |
0 |
0 |
10.62 |
10.92 |
17 |
0 |
−1 |
1 |
8.14 |
8.193 |
A second-order polynomial model was proposed for the response (Yi), and the regression coefficients were as follows:
|
 | (1) |
where
Yi is the predicted response value,
β0 is the model constant,
βi represents the linear effect terms,
βii is the quadratic effects and
βij is the interaction effects of the variables. Subsequently, three groups of confirmation experiments were carried out in order to verify the availability of the experimental design.
2.3 Physical and chemical properties
The chemical properties were determined by α-naphthol reaction, phenol-sulfuric acid reaction, FeCl3 reaction, Fehling's test, iodination reaction, carbazole reaction and ninhydrin test.
2.4 Preliminary analysis of polysaccharides
2.4.1 Analysis of monosaccharide composition. The monosaccharide composition of the polysaccharides was analyzed by HPLC with pre-column derivatization.19 RP was hydrolyzed with 4 M trifluoroacetic acid (TFA) at 110 °C for 4 h. After the reaction, the TFA was removed by co-distillation with methanol to afford the dry hydrolysate. NaOH (0.3 M, 100 μL) and PMP solution (0.5 M, 200 μL) were added to the dry hydrolysate. The solution was mixed and reacted for 2 hours at 70 °C. After incubation, the mixture was cooled to room temperature, followed by adjustment of the pH to neutral with HCl (0.3 M). Finally, the mixture was extracted with CHCl3 three times, and the aqueous phase was filtered through a 0.22 μm nylon membrane and analyzed by HPLC.The HPLC system was equipped with a C18 column (250 mm × 4.6 mm) and a DAD detector (250 nm). The mobile phase was 0.1 mol L−1 phosphate buffer (pH 6.7)–acetonitrile (82
:
18) at a flow rate of 1.0 mL min−1. The column temperature was 35 °C, and 10 μL of the resulting solution was injected each time.
2.4.2 FT-IR spectroscopy. FT-IR analysis was recorded with a Nicolet IS5 infrared spectrometer (Thermo Nicolet Co. USA) between wavelengths of 4000 and 400 cm−1 using the KBr disk method.20 Spectral resolution: 4 cm−1; scan number: 16.
2.4.3 Thermogravimetric analysis. A thermal analyzer was used to perform thermogravimetric scanning. The heating rate was 10 °C min−1, the heating range was 25–550 °C, the atmosphere was air and the flow rate was 30 mL min−1.
2.4.4 X-ray diffraction analysis. An X-ray powder diffractometer was used to determine the crystallization properties of the polysaccharides. The conditions of X-ray diffraction were Cu-Kα radiation, a 2θ test range of 5–80°, and a scan rate of 1.5° min−1.
2.5 Antioxidant activities in vitro
2.5.1 DPPH radical scavenging activity. The activity of RP to scavenge DPPH hydroxyl radical was measured according to the method of Shimada with a slight modification.21 First, 2 mL of DPPH solution (0.1 mM) and 2.0 mL deionized water were added to 2 mL of a different concentration of the sample solution in a test tube. The reaction solution was reacted in the dark at room temperature for 30 min, and the absorbance at 517 nm was measured. Ascorbic acid was used as a positive control. |
 | (2) |
where Ai is the absorbance of the sample, Aj is the absorbance of the sample only (without free radicals), and A0 is the absorbance of water instead of the sample.
2.5.2 ABTS radical scavenging activity. The ABTS scavenging activity was determined according to the literature.22 ABTS solution (7.4 mM) and potassium persulfate solution (2.6 mM) of the same volume were mixed and placed in the dark for 12 h. Before use, the ABTS solution was diluted with phosphate buffer solution (pH 7.4, 0.2 M) until the absorbance was 0.70 ± 0.02 under 734 nm. The polysaccharide solutions (1.0 mL) of different concentrations and the diluted ABTS solution (2.0 mL) were mixed and reacted for 6 min at room temperature; then, the absorbance was measured at 734 nm. The scavenging rate was calculated by eqn (2) as mentioned above.
2.5.3 Hydroxyl radical scavenging activity. Referring to Fenton's method, the hydroxyl radical scavenging activity of the polysaccharide was evaluated.23 Different concentrations of polysaccharide solution (2 mL) were mixed with FeSO4 (2 mL, 9 mM), salicylic acid (2 mL, 9 mM), and H2O2 (2 mL, 9 mM), respectively. The mixture was placed in a water bath at 37 °C for 30 minutes. Finally, the absorbance of the mixture was measured at 510 nm. The scavenging rate was calculated by eqn (2) as mentioned above.
2.5.4 Determination of reducing power. The reduction ability test was performed according to Pan Yingming's literature report with some modifications.24 Briefly, the sample solution (1.0 mL) of different concentrations, K3[Fe(CN)6] (1.0 mL, 1% w/v), and phosphate buffer (2.5 mL, 0.2 M, pH 6.6) were taken into a test tube and placed in a water bath at 50 °C for 20 min. Subsequently, TCA (2.5 mL, 10% w/v) was added to stop the reaction. Finally, the supernatant (2.5 mL) was mixed with distilled water (2.5 mL) and FeCl3 solution (0.5 mL, 1% w/v) in sequence. The absorbance was detected at 700 nm after 10 min.
2.6 Statistical analyses
Each experiment was repeated three times, and the average was taken as the observed response value. All the data are shown as the mean ± standard deviation (SD); analysis of variance (ANOVA) and P values were obtained by employing statistical software (SPSS 13.0.) P values below 0.05 were regarded as statistically significant.
3. Results and discussion
3.1 Optimization of polysaccharide extraction
3.1.1 Effect of the extraction temperature on the yield of polysaccharides. The experimental conditions for the single factor change of the extraction temperature were an extraction time of 3 h, extraction cycle number of 2 and material-to-liquid ratio of 1
:
40. As shown in Fig. 1(a), the extraction rate of polysaccharides rose sharply, but when the temperature exceeded 80 °C, the curve gradually became flat. The reason was that the increase in temperature was beneficial to the mass transfer rate of polysaccharide molecules and increased the solubility of the polysaccharides at the same time.25,26 When the temperature reached 80 °C, the polysaccharides in the solution almost reached a saturated state. Therefore, 80 °C was considered as the optimal extraction temperature.
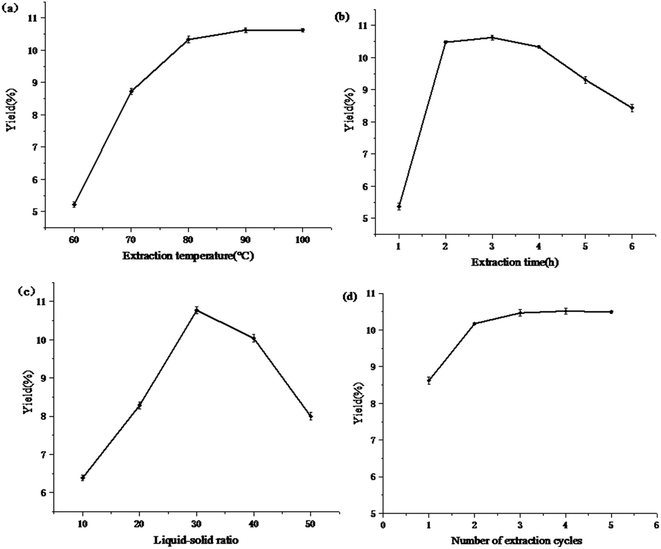 |
| Fig. 1 Effects of different (a) temperatures, (b) extraction times, (c) liquid–solid ratios and (d) extraction cycles on the extraction yield of RP. | |
3.1.2 Effect of the extraction time on the yield of polysaccharides. The experimental conditions for the time single factor change of the extraction time were an extraction temperature of 80 °C, a ratio of material to liquid of 1
:
40 and a number of extraction cycles of 2. As the extraction time increases, the extraction rate of polysaccharides gradually increases, as shown in Fig. 1(b). This may be because the polysaccharides were fully leached in the early stage of extraction, but the long-term extraction process may cause some hydrolysis and oxidation of polysaccharides and some polysaccharides may adhere to the macromolecular proteins to form precipitates.26,27 From an economical point of view, the optimized extraction time was determined to be 2 h.
3.1.3 Effect of the material liquid ratio on the yield of polysaccharides. The single-factor experiment conditions for the single factor change of the material liquid ratio were an extraction temperature of 80 °C, extraction time of 2 h and number of extraction cycles of 2. Fig. 1(c) shows that the polysaccharide yield increased first and then decreased. The reason may be that there was less solvent at the beginning of the extraction, and the polysaccharides were easily saturated after dissolution. Too much solvent caused the diffusion speed to decrease, and the polysaccharides were not completely leached.28 Therefore, an extraction ratio of 1
:
30 was favorable for polysaccharide extraction.
3.1.5 Model building and statistical analysis. The corresponding results of the RSM experiments are shown in Table 3. By applying multiple regression analysis to the experimental data, the prediction model was obtained through the following second-order polynomial equation according to the coded values: |
Y = 10.92 + 0.49X1 + 1.04X2 + 1.75X3 + 0.48X1X2 − 0.075X1X3 + 0.59X2X3 − 1.22X12 − 1.00X22 − 1.84X32
| (3) |
Table 3 ANOVA for the response surface quadratic modela
Sources |
Sum of squares |
Degrees of freedom |
Mean square |
F Value |
P Value |
R2 = 0.9924; Radj2 = 0.9825; Rpred2 = 0.9026; C.V.% = 2.97. |
Model |
65.03 |
9 |
7.23 |
101.04 |
<0.0001 |
X1 |
1.94 |
1 |
1.94 |
27.13 |
0.0012 |
X2 |
8.67 |
1 |
8.67 |
121.29 |
<0.0001 |
X3 |
24.61 |
1 |
24.61 |
344.06 |
<0.0001 |
X1X2 |
0.90 |
1 |
0.90 |
12.62 |
0.0093 |
X1X3 |
0.022 |
1 |
0.022 |
0.31 |
0.5923 |
X2X3 |
1.38 |
1 |
1.38 |
19.31 |
0.0032 |
X12 |
6.28 |
1 |
6.28 |
87.88 |
<0.0001 |
X22 |
4.25 |
1 |
4.25 |
59.38 |
0.0001 |
X32 |
14.32 |
1 |
14.32 |
200.26 |
<0.0001 |
Residual |
0.50 |
7 |
0.072 |
|
|
Lack of fit |
0.37 |
3 |
0.12 |
3.84 |
0.1134 |
Pure error |
0.13 |
4 |
0.032 |
|
|
Cor. total |
65.53 |
16 |
|
|
|
The values of R2 represent the fit ability of the model. For the quadratic regression model, the value of R2 and the predicted R2 were 0.9924 and 0.9062, respectively, which indicates that the experimental value has high accuracy and good reliability. In addition, the adjusted coefficient of determination (Radj2 = 0.9825) showed that the model was of great significance, and the coefficient of variation (C.V.% = 2.97) clearly indicated a very high degree of the accuracy and reliability of the polynomial model.29 Compared with the pure error, the lack of fit term of the equation was 0.11, with a p-value less than 0.05. The lack of fit was not significant, indicating that the model was stable and could be applied to predict the extraction rate within the experimental range adequately. As shown in Table 3, the linear coefficients (X1, X2 and X3), quadratic coefficients (X12, X22 and X32) and cross product coefficients (X1X2, X2X3) of the model were of great significance, and their p-values were less than 0.05. At the same time, other coefficients (X1X3) had no great effect on the extraction rate of RP. A conclusion could be obtained: the order of factors that had great effects on the response value of the extraction ratio of RP by observing the quadratic and linear coefficients was extraction time > extraction temperature > liquid–solid ratio.
As shown in Fig. 2, the observed value was compared to the predicted value calculated from the regression model, and the predicted value well matched the experimental value. This result suggests that the model was able to identify the operating conditions for the extraction of RP.
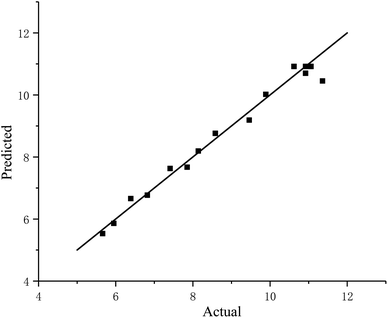 |
| Fig. 2 Comparison between the predicted and actual polysaccharide yields. | |
There are few studies on the extraction of rubescens; however, there are more similar studies using other materials. From previous studies, the effects of the linear terms and interaction terms were diverse between different species and different materials.30 Song et al.31 studied the extraction of defatted peanut cake polysaccharide and drew a conclusion that the best extraction conditions were extraction temperature 48.7 °C, extraction time 1.52 h, and ethanol concentration 61.9% (v/v), respectively. Luo et al.32 worked on dioscorea nipponica makino polysaccharide and found that the optimum conditions were liquid–solid ratio 33
:
1, extraction time 134 min, and extraction temperature 95 °C; they also found that the extraction temperature has a significant effect on the yield of polysaccharides. Dried materials compared with fresh materials take more energy to extract the polysaccharide, and a higher extraction temperature and a longer extraction time are needed.33 Different types of polysaccharides have different physical and chemical properties and structural characteristics; therefore, different types of polysaccharides have different solubilities in water and different extraction conditions.
3.1.6 Process optimization. According to the response surface method analysis data, the response surface and its contour map could be drawn, which could directly reflect the influence of the liquid-to-material ratio, the extraction temperature, the extraction time and their interaction on the yield of RP.34 All the drawings in Fig. 3 can be explained similarly. The results conformed to single factor test and ANOVA analysis. The applicability of the model equation to predict the optimal response value was tested using the recommended optimal conditions. When the optimal value of the independent variable (extraction temperature 87.70 °C, extraction time 2.59 h, material–liquid ratio 1
:
33.33) was incorporated into the regression equation, a yield of 11.91% of the polysaccharides could be obtained.
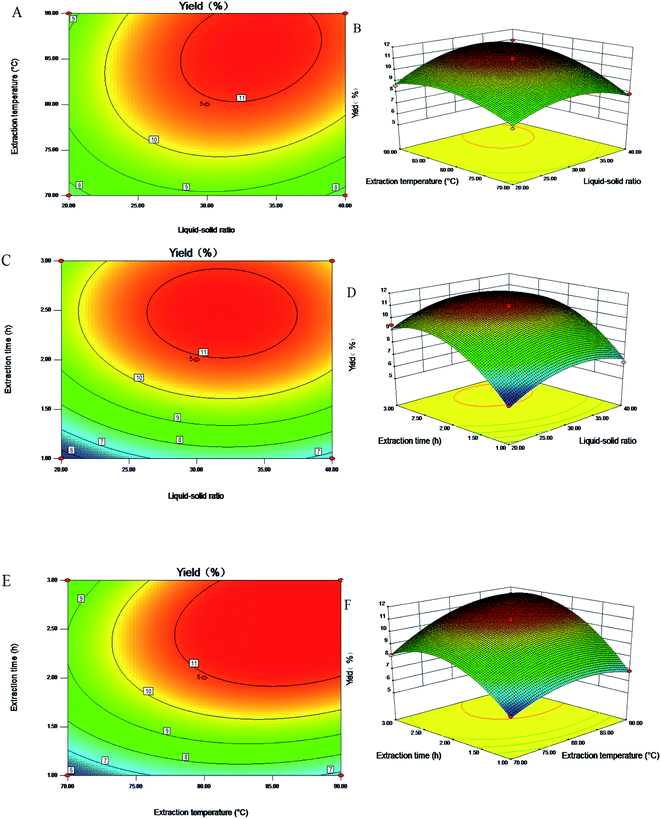 |
| Fig. 3 Response surface plots and contour plots showing the effects of the extraction time, extraction temperature and ratio of water to raw material on the response yield. | |
3.1.7 Confirmatory test. The suitability of the model equation for predicting the optimum response value was tested using the following conditions: extraction temperature 84.92 °C, material–liquid ratio 1
:
33, and extraction time 2.06 h. Taking into account the feasibility of the actual operation, the extraction conditions of the polysaccharides were modified to extraction temperature 85 °C, material–liquid ratio 1
:
33, and extraction time 2 h. Under these conditions, three parallel experiments were performed, and the average value was taken to obtain an RP extraction rate of 11.16% with an error of 0.11%. This value was close to the theoretical prediction value, indicating that the use of this model to optimize the process of extracting polysaccharides has certain practical significance.
3.2 Physical and chemical properties analysis
The RP content was 74.3 ± 1.2% measured by the phenol-sulfuric acid method after deproteinization and decolorization. Additionally, it was free of amino acids, starch, and proteins.
3.3 Preliminary structural analysis
The monosaccharide composition of the RP is illustrated in Fig. 4. The sugar compositional analysis of RP was mannose
:
glucose
:
galactose
:
xylose
:
arabinose = 2.27
:
4.65
:
2.57
:
1
:
1.85. The molar ratio is illustrated in Table 4.
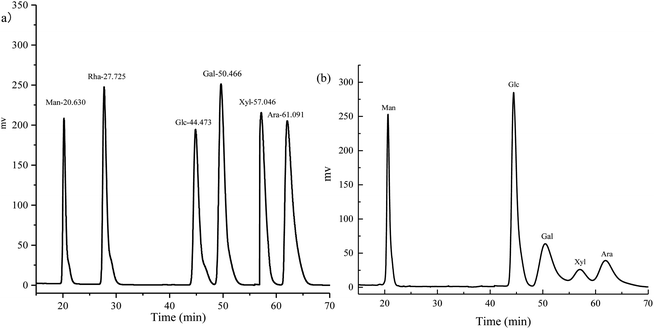 |
| Fig. 4 HPLC traces of the standard monosaccharide (a) and RP (b). | |
Table 4 Monosaccharide molar ratio of RP
Compound |
Molar ratio (%) |
Mannose |
18.4 |
Glucose |
37.7 |
Galactose |
20.8 |
Xylose |
8.1 |
Arabinose |
15.0 |
The FT-IR spectrum is shown in Fig. 5. Three strong absorption bands at 1152, 1074, and 1039 cm−1 in the range of 1200–1000 cm−1 indicated that the monosaccharide in RP had a pyranose ring.31 The characteristic strong peak at 3404 cm−1 was due to O–H stretching vibrations, and the vibration at 2930 cm−1 exhibited C–H stretching vibrations. The absorption peak at 1583 cm−1 was due to associated water.35 The absorption peak at 1414 cm−1 was dominated by C–O stretching vibrations, and the peak at 1070 cm−1 indicated that RP contains the ether bond (C–O–C) and the hydroxyl of the pyranose ring. The absorption at 896 cm−1 revealed that the polysaccharide contained a β-glycosidic linkage.36,37
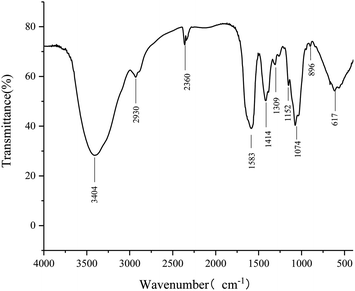 |
| Fig. 5 The FT-IR spectrum of RP. | |
As shown in Fig. 6, when 2θ was close to 20°, the characteristic diffraction curve of the polysaccharides was shown. This indicated that the polysaccharides existed in both crystalline and amorphous states and were semi-crystalline polymers.
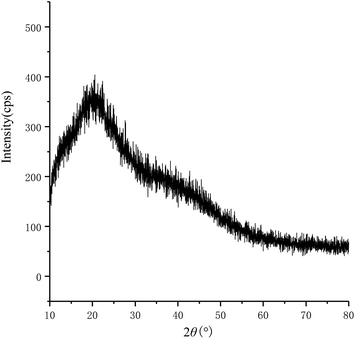 |
| Fig. 6 X-Ray diffraction analysis of RP. | |
As shown in Fig. 7, the TG curve was divided into three different stages. The first stage weight loss range was 25–205 °C, mainly due to the loss of water, and the mass loss rate was about 15.5%. The second rapid weight loss stage occurred at 205–433 °C, and the weight loss was 56.5%, which may be related to the thermal decomposition of the polysaccharides. When the third weight loss was in the range of 433–528 °C, the quality of the carbon was gradually reduced due to the thermal decomposition of carbon.
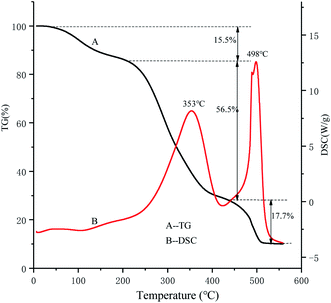 |
| Fig. 7 TG and DSC of RP. | |
3.4 Antioxidant activity assays
3.4.1 Scavenging effects of RP on DPPH radicals. DPPH radical is a free radical with a single electron but a relatively stable structure.38 When it interacts with a scavenger, the color of the solution changes. As shown in Fig. 8(a), the scavenging effect of RP on DPPH radical was positively correlated with the polysaccharide concentration from 0.1 to 1.6 mg mL−1. When the concentration was 1.6 mg mL−1, the DPPH radical scavenging rate was 54.1%. The activity may be caused by the hydrogen donation of the hydroxyl group of the polysaccharide or by the reduction of DPPH radical.39,40 Although there was a certain gap with Vc, the results also showed that RP could have stronger ability to donate electrons or hydrogen.
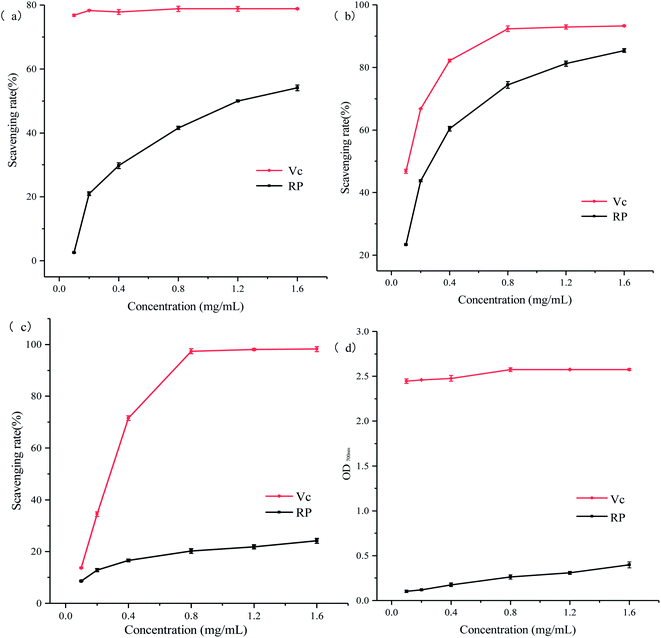 |
| Fig. 8 Antioxidant activity of RP: (a) scavenging activity to DPPH radicals; (b) scavenging activity to ABTS radicals; (c) scavenging activity to hydroxyl radicals; (d) reducing power. | |
3.4.2 Scavenging effects of RP on ABTS radicals. ABTS reacts with potassium persulfate to generate blue-green cationic radical ABTS radicals, and the antioxidant components will react with ABTS radicals to cause fading of the system, which reflects the antioxidant capacity of the substance.41 As shown in Fig. 8(b), the ABTS radical scavenging capacity of RP increased in a dose-dependent manner. When the concentration was 1.6 mg mL−1, the scavenging rate was 85.4%. The IC50 of the scavenging ability of RP on ABTS radicals was 0.27 mg mL−1. These results showed that RP has a very good scavenging effect on ABTS radicals.
3.4.3 Scavenging effects of RP on hydroxyl radicals. Hydroxyl free radicals are reactive oxygen species. The production of reactive oxygen species can cause oxidative damage to cell tissues and then cause disease.42 As shown in Fig. 8(c), the scavenging effect of RP on hydroxyl radicals showed an upward trend with the increase in concentration. At a concentration of 1.6 mg mL−1, its hydroxyl radical scavenging rate reached 24.16%. RP had weak hydroxyl radical scavenging ability on hydroxyl radicals. According to reports, the mechanism by which polysaccharides scavenge hydroxyl radicals involves the interaction of hydrogen and free radicals.43 However, the details of this mechanism have not been clarified.
3.4.4 Reducing power of RP. Reducing ability is an important indicator of the antioxidant performance of natural products. The electrons provided by antioxidants undergo a reduction reaction to achieve the purpose of scavenging free radicals.44 As shown in Fig. 8(d), when the concentration was 1.6 mg mL−1, the reducing ability of RP was 0.398. As the concentration of polysaccharides increased, there was a clear upward trend. The reducing ability of RP may be higher at a certain concentration.
4. Conclusions
RSM was applied to optimize the extraction parameters in this study. The experimental value of the polysaccharide yield varied from 5.66% to 11.36%. The variable with the largest effect was extraction time. The optimal conditions for the extraction of polysaccharide were as follows: extraction time 2 h, material–liquid ratio 1
:
33 and extraction temperature 85 °C. The preliminary characterization of RP was mainly mannose
:
glucose
:
galactose
:
xylose
:
arabinose = 2.27
:
4.65
:
2.57
:
1
:
1.85 by HPLC. Regarding antioxidant activity, the antioxidant capacity of RP was generally lower than that of ascorbic acid. However, RP performed well in the scavenging ability of ABTS free radicals. RP had a certain scavenging ability for DPPH and hydroxyl radicals. This result proved that RP can be used as a potential natural antioxidant for development in the future. In addition, further studies on the exact chemical structure of RP and the mechanism of its antioxidant activity are ongoing.
Conflicts of interest
There are no conflicts to declare.
References
- N. Bai, K. He, Z. Zhou, M.-L. Tsai and L. Zhang, Ent-Kaurane Diterpenoids from Rabdosia rubescens and Their Cytotoxic Effects on Human Cancer Cell Lines, Planta Med., 2010, 76(2), 140–145 CrossRef CAS PubMed.
- N. Bai, H. Kan, Z. Zhou, C. S. Lai, Z. Li, Z. Quan, X. Shao, M. H. Pan and C. T. Ho, Flavonoids from Rabdosia rubescens exert anti-inflammatory and growth inhibitory effect against human leukemia HL-60 cells, Food Chem., 2010, 122(3), 831–835 CrossRef CAS.
- Y. Ding, C. Y. Ding, N. Ye, Z. Q. Liu, E. A. Wold, H. Y. Chen, C. Wild, Q. Shen and J. Zhou, Discovery and development of natural product oridonin-inspired anticancer agents, Eur. J. Med. Chem., 2016, 47, 102–117 CrossRef PubMed.
- S. X. Huang, Y. Zhou, J. X. Pu, R. T. Li and H. D. Sun, Cytotoxic ent-kauranoid derivatives from Isodon rubescens, Tetrahedron, 2006, 62(20), 4941–4947 CrossRef CAS.
- H. D. Sun, Q. Z. Zhou and T. Fujita, Rubescensin D, a diterpenoid from Rabdosia rubescens, Phytochemistry, 1992, 31, 1418–1419 CrossRef CAS.
- Q. B. Han, J. X. Zhang, A. H. Zhao, H. D. Sun, Y. Lu, Y. S. Wu and Q. T. Zheng, Two novel tricyclic diterpenoids from Isodon rubescens var. taihangensis, Tetrahedron, 2004, 60(10), 2373–2377 CrossRef CAS.
- J. Yang, W. G. Wang, H. Y. Wu, M. Liu, H. Y. Jiang, X. Du, Y. Li, J. X. Pu and H. D. Sun, Ent-Kaurene diterpenoids from Isodon phyllostachys, Tetrahedron Lett., 2016, 58(4), 349–351 CrossRef.
- P. Gong, S. Y. Wang, M. Liu, F. X. Chen and X. F. Chen, Extraction methods, chemical characterizations and biological activities of mushroom polysaccharides: a mini-review, Carbohydr. Res., 2020, 494, 108037 CrossRef CAS PubMed.
- S. Lei, Bioactivities, isolation and purification methods of polysaccharides from natural products: a review, Int. J. Biol. Macromol., 2016, 37–48 Search PubMed.
- S. S. J. Chelladurai, K. Murugan, A. P. Ray, M. Upadhyaya, V. Narasimharaj and S. Gnanasekaran, Optimization of process parameters using response surface methodology: a review, Mater. Today: Proc., 2020, 1301–1304 Search PubMed.
- Y. X. Sun, T. B. Li, J. W. Yan and J. C. Liu, Technology optimization for polysaccharides (POP) extraction from the fruiting bodies of Pleurotus ostreatus by Box–Behnken statistical design, Carbohydr. Polym., 2010, 80(1), 242–247 CrossRef CAS.
- H. Zhao, J. Wang and Z. Lu, Optimization of process parameters of the Pholiota squarrosa extracellular polysaccharide by Box–Behnken statistical design, Carbohydr. Polym., 2009, 77(3), 677–680 CrossRef CAS.
- J. P. Maran, S. Manikandan, K. Thirugnanasambandham, C. V. Nivetha and R. Dinesh, Box-Behnken design based statistical modeling for ultrasound-assisted extraction of corn silk polysaccharide, Carbohydr. Polym., 2013, 92(1), 604–611 CrossRef PubMed.
- J. Fan, H. Feng, Y. Yu, M. Sun, Y. Liu, T. Li, X. Sun, S. Liu and M. Sun, Antioxidant activities of the polysaccharides of Chuanminshen violaceum, Carbohydr. Polym., 2017, 157, 629–636 CrossRef CAS PubMed.
- S. Y. Tian, C. C. Hao, G. K. Xu, J. J. Yang and R. G. Sun, Optimization conditions for extracting polysaccharide from Angelica sinensis and its? antioxidant activities, J. Food Drug Anal., 2017, 766–775 CrossRef CAS PubMed.
- Y. Zhang, D. Ling, X. Kong and L. Chen, Characterization and in vitro antioxidant activities of polysaccharides
from Pleurotus ostreatus[J]., Int. J. Biol. Macromol, 2012, 51(3), 259–265 CrossRef CAS PubMed.
- J. Fang, Z. Wang, P. Wang and M. Wang, Extraction, structure and bioactivities of the polysaccharides from Ginkgo biloba: a review, Int. J. Biol. Macromol, 2020, 162, 1897–1805 CrossRef CAS PubMed.
- M. Dubois, K. A. Gilles, J. K. Hamilton, P. A. Rebers and F. Smith, Colorimetric Method for Determination of Sugars and Related Substances, Anal. Chem., 1956, 28(3), 350–356 CrossRef CAS.
- J. J. Zhang, Q. B. Zhang, J. Wang, X. L. Shi and Z. S. Zhang, Analysis of the monosaccharide composition of fucoidan by precolumn derivation HPLC, Chin. J. Oceanol. Limnol., 2009, 27(3), 578–582 CrossRef CAS.
- L. Nenad, S. Vojin, N. Stevo, P. Milenko and U. Dragan, A study of HAp/PLLA composite as a substitute for bone powder, using FT-IR spectroscopy, Biomaterials, 2001, 22(6), 571–575 CrossRef.
- S. Kazuko, F. Kuniko, Y. Keiko and N. Takashi, Antioxidative properties of xanthan on the autoxidation of soybean oil in cyclodextrin emulsion, J. Agric. Food Chem., 1992, 40(6), 945–948 CrossRef.
- C. J. Yue, J. Chen, R. R. Hou, J. Liu and X. P. Li, Effects of Selenylation Modification on Antioxidative Activities of Schisandra chinensis Polysaccharide, PLoS One, 2015, e0134363 CrossRef PubMed.
- J. Tang, J. Nie, D. P. Li, W. J. Zhu, S. P. Zhang, F. Ma, Q. Sun, J. Song, Y. L. Zheng and P. Chen, Characterization and antioxidant activities of degraded polysaccharides from Poria cocos sclerotium, Carbohydr. Polym., 2014, 105, 121–126 CrossRef CAS PubMed.
- Y. M. Pan, K. Wang, S. Q. Huang, H. S. Wang and X. M. Mu, Antioxidant activity of microwave-assisted extract of longan (Dimocarpus Longan Lour.) peel, Food Chem., 2008, 106(3), 1264–1270 CrossRef CAS.
- E. V. Vinogradov, L. Brade and H. Brade, Structural and serological characterisation of the O-antigenic polysaccharide of the lipopolysaccharide from Acinetobacter baumannii strain 24, Carbohydr. Res., 2003, 2751–2756 CrossRef CAS PubMed.
- M. E. M. Braga, S. R. M. Moreschi and M. A. A. Meireles, Effects of supercritical fluid extraction on Curcuma longa L. and Zingiber officinale R. starches, Carbohydr. Polym., 2006, 63(3), 340–346 CrossRef CAS.
- E. V. Vinogradov, L. Brade, H. Brade and O. Holst, Structural and serological characterisation of the O-antigenic polysaccharide of the lipopolysaccharide from Acinetobacter baumannii strain 24, Carbohydr. Res., 2003, 338(23), 2751–2756 CrossRef CAS PubMed.
- M. B. Hu, W. Peng, Y. J. Liu, N. Wu, C. B. Zhao, D. S. Xie, D. Yan, X. F. Zhang, X. B. Tao and C. J. Wu, Optimum Extraction of Polysaccharide from Areca catechu Using Response Surface Methodology and its Antioxidant Activity, J. Food Process. Preserv, 2017, 41(1), e12798 CrossRef.
- W. Li, S. W. Cui and Y. Kakuda, Extraction, fractionation, structural and physical characterization of wheat β-D-glucans, Carbohydr. Polym., 2006, 63(3), 408–416 CrossRef CAS.
- X. Guo, X. Zou and M. Sun, Optimization of extraction process by response surface methodology and preliminary characterization of polysaccharides from Phellinus igniarius, Carbohydr. Polym., 2010, 80(2), 344–349 CrossRef CAS.
- Y. Song, B. J. Du, T. Zhou, B. Han, F. Yu, R. Yang, X. S. Hu, Y. Y. Ni and Q. H. Li, Optimization of extraction process by response surface methodology and preliminary structural analysis of polysaccharides from defatted peanut (Arachis hypogaea) cakes, Carbohydr. Res., 2011, 346(2), 305–310 CrossRef CAS PubMed.
- D. H. Luo, Optimization of total polysaccharide extraction from Dioscorea nipponica Makino using response surface methodology and uniform design, Carbohydr.
Polym., 2012, 90(1), 284–288 CrossRef CAS PubMed.
- Y. X. Sun, Y. J. Li, M. Q. Li, H. B. Tong, X. D. Yang and J. C. Liu, Optimization of extraction technology of the Anemone raddeana polysaccharides (ARP) by orthogonal test design and evaluation of its anti-tumor activity, Carbohydr. Polym., 2009, 75(4), 575–579 CrossRef CAS.
- R. Muralidhar, R. Chirumamila, R. Marchant and S. N. N. Poonam, A response surface approach for the comparison of lipase production by Candida cylindracea using two different carbon sources, Biochem. Eng. J., 2001, 9(1), 17–23 CrossRef CAS.
- W. Cao, X. Q. Li, L. Liu, M. Wang, H. T. Fan, C. Li, Z. Lv, X. Wang and Q. Mei, Structural analysis of water-soluble glucans from the root of Angelica sinensis (Oliv.) Diels, Carbohydr. Res., 2006, 341(11), 1870–1877 CrossRef CAS PubMed.
- W. Cao, X. Q. Li, L. Liu, M. C. Wang, H. T. Fan, C. Li, Z. G. Lv, X. J. Wang and Q. B. Mei, Structural analysis of water-soluble glucans from the root of Angelica sinensis (Oliv.) Diels, Carbohydr. Res., 2006, 341(11), 1870–1877 CrossRef CAS PubMed.
- M. J. Wu, D. Z. Jiang and T. M. Liu, Structural Analysis of Water-soluble Polysaccharide PIP1 Extracted from the Cultured Mycelium of Phellinus igniarius, Chem. Res. Chin. Univ., 2006, 22(6), 708–711 CrossRef CAS.
- M. Öztürk, F. Aydoğmuş-Öztürk, M. E. Duru and G. Topçu, Antioxidant activity of stem and root extracts of Rhubarb (Rheum ribes): An edible medicinal plant, Food Chem., 2007, 103(2), 623–630 CrossRef.
- J. H. Xie, M. Y. Shen, M. Y. Xie, S. P. Nie, Y. Chen, C. Li, D. F. Huang and Y. X. Wang, Ultrasonic-assisted extraction, antimicrobial and antioxidant activities of Cyclocarya paliurus (Batal.) Iljinskaja polysaccharides, Carbohydr. Polym., 2012, 89(1), 177–184 CrossRef CAS PubMed.
- R. Z. Chen, S. Z. Li, C. M. Liu, S. M. Yang and X. L. Li, Ultrasound complex enzymes assisted extraction and biochemical activities of polysaccharides from Epimedium leaves, Process Biochem., 2012, 47(12), 2040–2050 CrossRef CAS.
- K. Durgo, M. Koncar, D. Komes, A. Belscak-Cvitanovic, J. Franekic, I. Jakopovich, N. Jakopovich and B. Jakopovich, Cytotoxicity of blended versus single medicinal mushroom extracts on human cancer cell lines: contribution of polyphenol and polysaccharide content, Int. J. Med. Mushrooms, 2013, 15(5), 435–448 CrossRef CAS PubMed.
- X. H. Zhang, L. Liu and C. W. Lin, Isolation, structural characterization and antioxidant activity of a neutral polysaccharide from Sisal waste, Food Hydrocolloids, 2014, 39, 10–18 CrossRef CAS.
- E. Tsiapali, S. Whaley, J. Kalbfleisch, H. E. Ensley, L. W. Browder and D. L. Williams, Glucans exhibit weak antioxidant activity, but stimulate macrophage free radical activity, Free Radicals Biol. Med., 2001, 30(4), 393–402 CrossRef CAS PubMed.
- S. Q. Li and N. P. Shah, Characterization, antioxidative and bifidogenic effects of polysaccharides from Pleurotus eryngii after heat treatments, Food Chem., 2016, 197, 240–249 CrossRef CAS PubMed.
|
This journal is © The Royal Society of Chemistry 2021 |
Click here to see how this site uses Cookies. View our privacy policy here.