DOI:
10.1039/D1RA01088C
(Paper)
RSC Adv., 2021,
11, 9426-9432
Total synthesis of landomycins Q and R and related core structures for exploration of the cytotoxicity and antibacterial properties†
Received
9th February 2021
, Accepted 23rd February 2021
First published on 2nd March 2021
Abstract
Herein, we report the total synthesis of landomycins Q and R as well as the aglycone core, namely anhydrolandomycinone and a related core analogue. The synthesis features an acetate-assisted arylation method for construction of the hindered B-ring in the core component and a one-pot aromatization–deiodination–denbenzylation procedure to streamline the global functional and protecting group manuipulation. Subsequent cytotoxicity and antibacterial studies revealed that the landomycin R is a potential antibacterial agent against methicillin-resistant Staphylococcus aureus.
Introduction
Over the past few decades, the emergence of multidrug-resistant nosocomial pathogens has become a worldwide issue that is of concern for scientists and clinical practitioners.1,2 Consequently, new antibiotics and antibacterial strategies are urgently needed.3,4 However, the development of a new antibiotic is a slow process that is associated with a high risk of failure. Compounds that exhibit antimicrobial capacity in vitro may be unable to combat the infection in vivo or may be highly toxic to the host cells.5
Staphylococcus aureus (S. aureus) is a class of Gram-positive bacteria that is present on the skin and mucous membranes as a part of normal flora.6 If by chance S. aureus bacteria enter the bloodstream, they may cause severe infections including meningitides, endocarditis, and urinary tract infections.7 Staphylococcal infections are common in community and hospital-acquired settings and treatment of these infections has been complicated because of the rising incidence of methicillin resistant S. aureus (MRSA) infections.8 Although vancomycin is the antibiotic of choice, it may cause nephrotoxicity at high doses.9,10 Therefore, a less or non-toxic antibiotic is desirable.11–13
The landomycins (LAs) are a class of angucyclines produced from Streptomyces species.14 The first report on LA described the isolation of LA A to D from S. cyanogenus S136.15 Through the use of the modern molecular biology techniques, the LA family has now grown to include more than 100 members.16,17 The general structure of LA compounds comprises a tetracyclic core, which is substituted with some hydroxyl groups and a 2-deoxysaccharide chain at the C8 position (Fig. 1a).14–17 Based on the B-ring structure and hydroxyl substitution pattern, four different core structures have been identified: tetrangulol, 5,6-anhydrolandomycinone, landomycinone, and 11-deoxy-landomycinone. The combinations of different core structures and 2-deoxysaccharide chains provide a rich pool of natural products for structure and activity relationship studies.
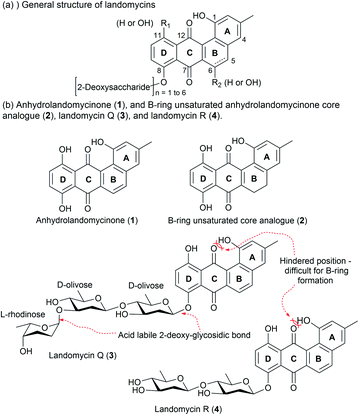 |
| Fig. 1 (a) General Structure of LAs. (b) Structures of anhydrolandomycinone aglycone core (1), and B-ring unsaturated core analogue (2), LA R (3) and LA Q (4). | |
Previous studies of LAs have mainly focused on the anti-cancer activity, and their potential antibacterial property has received far less attention.18 Given that the demand for non-toxic antibiotics is on the rise, we sought to establish a versatile synthetic route to procure the anhydrolandomycinone core (1), the B-ring unsaturated core analogue (2), the LA Q (3), and LA R (4) for exploration of the cytotoxic and antibacterial properties (Fig. 1b).
Results and discussion
Synthesis of anhydrolandomycinone (1), B-ring unsaturated core analogue (2), LA Q (3), and LA R (4)
The total synthesis of LA A and LA D has been reported by Yu and Yang, but the synthesis of LA Q (3) and LA R (4) with a different core structure has not yet been realized.19 From structural perspective, the synthesis of this class of compounds is non-trivial and yet challenging. Currently available protocols toward their aglycone core structure and glycone component alone are inadequate for procurement of a complete LA scaffold.20,21 Some modifications are required for selective removal of protecting groups and subsequent coupling reactions; but such modifications may not be tolerable to the original protocols. Thereby, we envisaged some synthetic challenges to realize the structures of our targets. These include: (i) the construction of a highly hindered B-ring; (ii) stereocontrol of the formation of the 2-deoxy-β-glycosidic bonds; and (iii) the global deprotection in conditions tolerated by the rather fragile trideoxy- and dideoxy-glycosidic bonds (Fig. 1b).22
To tackle these challenges, new synthetic routes were designed, which was based on the modifications of literature protocols.19–21 To access anhydrolandomycinone core (1) and its B-ring unsaturated analogue (2), the D-ring and A-ring building blocks 5 and 6 were prepared from available hydroquinone 7 and dimethylanisole 8, respectively. Based on the building blocks 5 and 6, the C and B-rings of the core scaffold were constructed.
In the preparation of the D-ring building block 5, regio-selective acetylation of hydroquinone 7 with Zhang's procedure23 followed by sequential benzylation and bromination gave the known 3-bromo-4-hydroxyphenyl acetate 9.24 Then, 9 was converted to 1-(benzyloxy)-2-bromo-4-(methoxy-methoxy)-benzene 10 through deacetylation and methoxymethyl ether (MOM) protection (Scheme 1a). Subsequent lithiation of 10 in couple with Meerwein's salt methylation completed the preparation of building block 5 in 37% yield over seven steps.25 Because of the poor stability of 5, it was freshly prepared prior to the Dötz benzannulation shown in Scheme 1c.
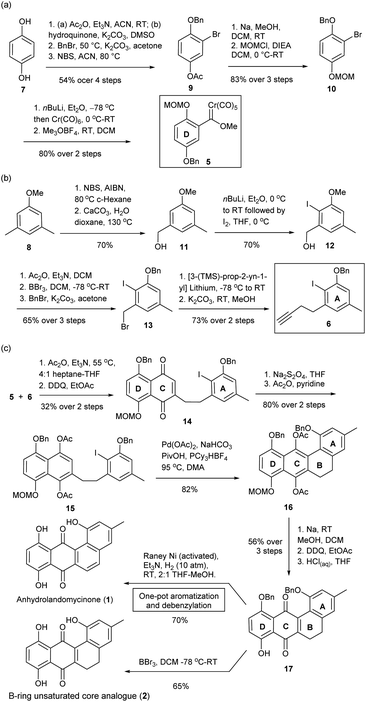 |
| Scheme 1 Preparation of (a) D-ring building block 5, (b) A-ring alkyne building block 6, (c) anhydrolandomycinone (1), and corresponding B-ring unsaturated core analogue (2). | |
The preparation of the A-ring building block 6 started with readily available 3,5-dimethylaniosle 8 (ref. 20b) instead of the more expensive 3-bromo-5-methylphenol as was the case in our previous synthesis.20d Thus, bromination of 3,5-dimethylaniosle 8 with Wohl–Ziegler's procedure26 followed by hydroxyl substitution afforded known (3-methoxy-5-methylphenyl)-methanol 11 (Scheme 1b).20b Subsequent directed-ortho lithiation of 11 and regioselective iodination gave (2-iodo-3-methoxy-5-methylphenyl)methanol 12 as the sole isomer.20b,27 Acetylation of 12 followed by replacement of the methoxy group with benzyl ether protection provided 1-(benzyloxy)-3-(bromomethyl)-2-iodo-5-methylbenzene 13.20d Finally, the substitution of 13 with in situ prepared [3-TMS-prop-2-yn-1-yl] lithium and desilylation completed the preparation of building block 6 in 23% yield over eight steps.
With the D- and A-ring building blocks 5 and 6 in hand, we embarked on the C-ring construction via Dötz benzannulation (Scheme 1c).28 The annulation was followed by 2,3-dichloro-5,6-dicyano-benzoquinone (DDQ) oxidation to give the A-ring tethered naphthalene-1,4-dione 14; which through Na2S2O4 reduction and acetylation was converted to the A-ring tethered naphthalene-1,4-diacetate 15. Subsequent acetate-assisted intramolecular directed arylation enabled construction of the B-ring in a highly congested environment.29 The desired 5,6-dihydrotetraphene-7,12-diyl-diacetate 16 was procured in a good 82% yield along with just a trace amount of the deiodination product of 15. Removal of the acetyl groups of 16 followed by DDQ oxidation and acidic cleavage of the MOM protecting group gave 5,6-dihydrotetraphene-7,12-dione 17. In subsequent hydrogenolytic debenzylation, the modified RANEY®Ni catalyzed hydrogenation conditions were applied; whereas, the RANEY®Ni catalyst was freshly activated with NaOH(aq) and triethylamine (Et3N) was added as an acid scavenger.30 Under the new conditions, no reduction of the carbonyl groups at C-ring occurred, while the B-ring was aromatized to give desired anhydrolandomycinone (1) in a one-pot fashion. In previous hydrogenation conditions, the carbonyl groups at the C-ring were also reduced, which had to be recovered by the DDQ oxidation.19,20d In addition to the synthesis of (1), removal of the benzyl protecting groups of 17 with BBr3 furnished the B-ring unsaturated core analogue (2).
For the synthesis of LA Q (3) and R (4), partially protected anhydrolandomycinone 18 was initially employed as the aglycone acceptor, which could be derived from advanced intermediate 16 via four functional- and protecting-group manipulation steps (Scheme 2a). The 2-deoxytrisaccharide component of LA Q (3) was assembled from L-rhodinosyl acetates 19 (ref. 31) and, D-olivosyl acetates 20,19a and 21, while the 2-deoxy-disaccharide component of LA R (4) was built from the D-olivosyl acetates 21 and 22 (ref. 19a) (Scheme 2b). L-Rhodinosyl acetate 19 was derived from L-rhamnose (Scheme S1†), and D-olivosyl acetates 20 and 22 were derived from known glucal 23 according to literature procedures (Scheme S2†).32 6-Iodo-olivosyl acetate 21 was also prepared from 23 via intermediates 24 and 25 in 20% overall yield (Scheme 2c).
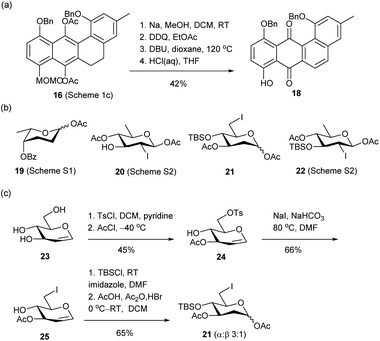 |
| Scheme 2 (a) Preparation of anhydrolandomycinone acceptor 18. (b) Structures of L-rhodinosyl acetate 19 and olivosyl acetates 20–22. (c) Preparation of D-olivosyl acetate 21. | |
In the construction of LA Q (3), an anhydrolandomycinonyl β-olivoside acceptor was prepared, which was subsequently coupled with a non-reducing-end disaccharide donor. In the preparation of the β-olivoside acceptor, 6-iodo-olivosyl acetate 21 was converted to an α-olivosyl iodide by treatment with trimethylsilyl iodide (TMSI) (Scheme 3a).33 After formation of the iodide intermediate, the DCM and residual TMSI were removed and the crude intermediate was reacted with the phenolate nucleophile, which was prepared in situ by deprotonation of 18 with potassium hexamethyldisilazane (KHMDS). Under the SN2 glycosylation conditions, the desired anhydrolandomycinonyl β-olivoside 26 was obtained in a satisfactory 68% yield with perfect selectivity. Assignment of the configuration of the O-aryl 2-deoxy-β-glycosidic bond was supported by the 3JH1-Hax coupling constant of 9.6 Hz. Notably, the above substitution occurred exclusively at C1 position despite the presence of the iodo substituent at the C6 position. Subsequent removal of the silyl protecting group afforded the desired β-olivoside acceptor 27 in 65% yield.
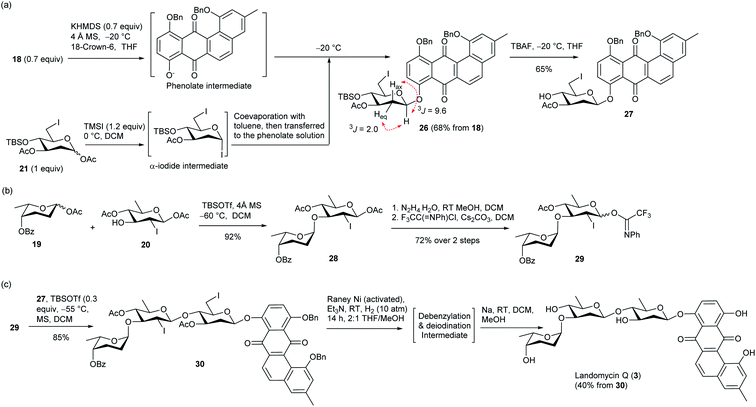 |
| Scheme 3 (a) Preparation of anhydrolandomycinonyl β-olivoside acceptor 27. (b) Preparation of disaccharide imidate donor 29. (c) Total synthesis of LA Q (3). | |
For the preparation of the disaccharide donor, olivosyl acetate acceptor 20 was coupled with rhodinosyl acetate donor 19 to give α-rhodinosyl-(1,3)-olivosyl acetate 28 (Scheme 3b). In the glycosylation, a low −60 °C temperature was applied to avoid the cleavage of the fragile 2,3,6-trideoxyglyosidic bond. Final replacement of the anomeric acetate group of 28 with N-phenyltrifluoroacetimidate completed the preparation of disaccharide imidate donor 29.
The availability of donor 29 set the stage for the assembly of the target (Scheme 3c). Glycosylation of acceptor 27 with donor 29 gave protected LA Q 30 in a high 85% yield with excellent β-selectivity. The selectivity of the glycosylation is attributed to the participatory effect of the 2-iodo substituent group.34 Subsequent one-pot deiodination and debenzylation of 30 could be achieved under the same RANEY®Ni hydrogenation conditions as shown in Scheme 1c. Hydrolysis of the acyl protecting groups of the deiodinated and debenzylated intermediate concluded the synthesis of LA Q (3) in 40% yield over two steps from 30. The identity and structure of 3 were confirmed with HRMS and NMR spectroscopy. The proton chemical shifts of (3) were in full agreement with the data given from an authentic sample (Table S1†).16c
Inspired by the one-pot debenzylation and aromatization in Scheme 1c, and the one-pot debenzylation and deiodination in Scheme 3c, we envisaged a simpler synthetic route for LA R (4), which would invoke a one-pot three-step, i.e. aromatization–debenzylation–deiodination, procedure. With this aim, 5,6-dihydrotetraphene-7,12-dione 17 in Scheme 1c was coupled with 6-iodo olivosyl acetate 21 according to the above SN2-glycoslation procedure to give 5,6-dihydrotetraphene-7,12-dione β-olivoside 31 in 68% yield along with ca. 5% of the inseparable aromatization product 26 (Scheme 4a). The crude β-olivoside 31 was subjected to desilylation to give the 5,6-dihydrotetraphene-7,12-dionyl β-olivoside acceptor 32 along with a small amount of olivoside 27.
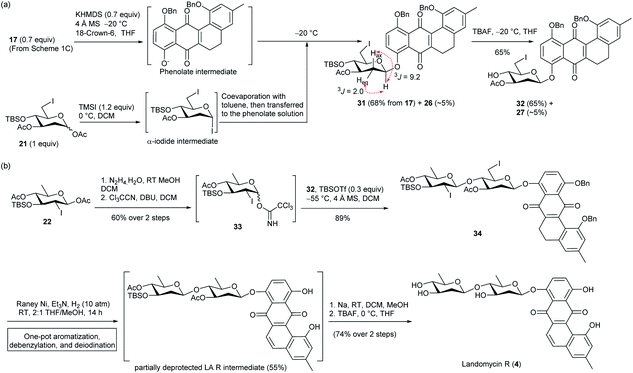 |
| Scheme 4 (a) Preparation of B-ring unsaturated anhydrolandomycinonyl core acceptor 32. (b) Total synthesis of LA R (4). | |
On the other hand, the non-reducing end olivosyl acetate 22 was converted to the trichloroacetimidate donor 33 by standard procedures (Scheme 4b). Subsequent glycosylation of β-olivoside acceptor 32 with donor 33 furnished 8-disaccharidyl-5,6-dihydrotetraphene-7,12-dione 34 in 89% yield, though a tiny amount of glycosylation product arising from contaminant olivoside 27 was also produced. Without separation, the crude β-glycoside 34 was subjected to the one-pot aromatization–debenzylation–deiodination procedure to afford a partially deprotected LA R intermediate in a satisfactory 55% yield. Final deprotection of the silyl and acetyl protecting groups concluded the total synthesis of LA R (4) in 74% yield over two steps.
Exploration of the cytotoxic and antibacterial properties
Having acquired anhydrolandomycinone (1), the B-ring unsaturated core analogue (2), LA Q (3) and LA R (4), we evaluated their cytotoxicity with the [3-(dimethylthiazol-2-yl)-5-(3-carboxymethoxyphenyl)-2-(4-sulfo-phenyl)-2H-tetrazolium] (MTS) assay (Table 1).35,36 Anhydrolandomycinone (1) was found to be cytotoxic against the cancer cell lines NCI-H460 and SF-268 with IC50 values at 7.00 ± 0.70 and 8.57 ± 0.34 μM, respectively; but no appreciable cytoxicity was noted for normal cell line Detroit 551 at 10 μM (Entry 1). Intriguingly, such a toxicity pattern was reversed for the B-ring unsaturated core analogue (2) (Entry 2). At 10 μM concentration of glycosylated LA Q (3) and R (4), no appreciable cytotoxic effect was detected for the normal cell line (Entries 3 and 4). Taken together, the unsaturated B ring and/or the 2-deoxysaccharide chain appear to play a role in the cytotoxicity of the LA compounds.
Table 1 Inhibitory effects on normal/cancer cell proliferation by anhydrolandomycinone (1), B-ring unsaturated core analogue (2), LA Q (3), and LA R (4)a
Entry |
Compound |
IC50 (μM) |
NCI-H460 |
SF-268 |
Detroit 551 |
Values represent the mean ± SD of three independent experiments. |
1 |
Core (1) |
7.00 ± 0.70 |
8.57 ± 0.34 |
>10.0 |
2 |
B-ring unsaturated core (2) |
>10.0 |
>10.0 |
9.58 ± 0.66 |
3 |
LA Q (3) |
>10.0 |
>10.0 |
>10.0 |
4 |
LA R (4) |
>10.0 |
9.82 ± 0.08 |
>10.0 |
To elucidate the extent of the cytotoxicity, we evaluated the survival rates of Detroit 551 cell line at 10 μM of the compounds, which were found to be 59.00% ± 3.26% for the core structure (1), 104.2% ± 20.67% for LA Q (3), and 93.56% ± 10.02% for LA R (4), implicating that the anhydrolandomycinone alone is more toxic than its glycosylated products.
After clarifying the cytotoxicity profiles of 1–4, we examined the antibacterial activity with MRSA 4N 216 and 7Y001 (Table 2).37,38 Among the compounds examined, only the disaccharide substituted LA R (4) exhibited inhibitory activity against MRSA 4N 216 and 7Y001 with the MIC values of 8 and 4 μg mL−1, respectively. Although, such a potency is inferior to that offered by vancomycin (VA), the non-toxic nature of LA R (4) renders it a potential candidate for further optimization.
Table 2 In vitro inhibitory assay with anhydrolandomycinone (1), B-ring unsaturated core analogue (2), LA Q (3), and LA R (4)a
MRSA strain |
MIC (μg mL−1) of compound |
Core (1) |
Analogue (2) |
LA Q (3) |
LA R (4) |
VA |
Values are derived from two independent MIC experiments. |
4N216 |
>8 |
>8 |
>8 |
8 |
1 |
7Y001 |
>8 |
>8 |
>8 |
4 |
1 |
Conclusion
In summary, a versatile strategy was established for the total synthesis of the anhydrolandomycinone core (1), the B-ring unsaturated anhydrolandomycinone analogue (2), landomycin Q (3), and landomycin R (4). The strategy employed the acetate-assisted intramolecular arylation for construction of the hindered B-ring of the anhydrolandomycin core and a one-pot aromatization, deiodination, and debenzylation procedure was established to simplify the end-stage functional group manipulations. In subsequent MTS study, the structure of the B-ring and the degree of glycosylation of the landomycins appear to play a role in the cytotoxicity. Further exploration of the antibacterial properties revealed the potential of landomycin R (4) for inhibition of MRSA 4N 216 and 7Y001.
Conflicts of interest
There are no conflicts to declare.
Acknowledgements
Thanks are given to the Ministry of Science and Technology in Taiwan (MOST) for financial support (grant no. MOST 109-2113-M-009-007).
Notes and references
-
(a) A. W. Robert, G. Robert and R. E. Jonathan, Overview of Nosocomial Infections Caused by Gram-Negative Bacilli, Clin. Infect. Dis., 2005, 41, 848–854 CrossRef;
(b) F. Perez, A. M. Hujer, K. M. Hujer, B. K. Decker, P. N. Rather and R. A. Bonomo, Global challenge of multidrug-resistant A. baumannii, Antimicrob. Agents Chemother., 2007, 51, 3471–3484 CrossRef CAS;
(c) E. M. D'Agata, Rapidly rising prevalence of nosocomial multidrug-resistant, Gram-negative bacilli: a 9-year surveillance study, Infect. Control Hosp. Epidemiol., 2004, 25, 842–846 CrossRef.
- M. M. D'Andrea, M. Fraziano, M. C. Thaller and G. M. Rossolini, The Urgent Need for Novel Antimicrobial Agents and Strategies to Fight Antibiotic Resistance, Antibiotics, 2019, 8, 254 CrossRef.
- I. Roca, et al., The global threat of antimicrobial resistance: science for intervention, New Microbes New Infect., 2015, 6, 22–29 CrossRef CAS.
- J. Bérdy, Thoughts and facts about antibiotics: where we are now and where we are heading, J. Antibiot., 2012, 65, 385–395 CrossRef.
-
(a) A. P. Tomaras, J. L. Crandon, C. J. McPherson, M. A. Banevicius, S. M. Finegan, R. L. Irvine, M. F. Brown, J. P. O'Donnell and D. P. Nicolau, Adaptation-Based Resistance to Siderophore-Conjugated Antibacterial Agents by Pseudomonas aeruginosa, Antimicrob. Agents Chemother., 2013, 57, 4197–4207 CrossRef CAS;
(b) M. Ghosh, P. A. Miller, U. Möllmann, W. D. Claypool, V. A. Schroeder, W. R. Wolter, M. Suckow, H. Yu, S. Li, W. Huang, J. Zajicek and M. J. Miller, Targeted Antibiotic Delivery: Selective Siderophore Conjugation with Daptomycin Confers Potent Activity against Multidrug Resistant Acinetobacter baumannii Both In Vitro and In Vivo, J. Med. Chem., 2017, 60, 4577–4583 CrossRef CAS;
(c) J.-K. Huang, T.-L. Yang Lauderdale, C.-C. Lin and K.-S. Shia, Total Synthesis of Tetarimycin A, (±)-Naphthacemycin A9, and (±)-Fasamycin A: Structure–Activity Relationship Studies against Drug-Resistant Bacteria, J. Org. Chem., 2018, 83(12), 6508–6523 CrossRef CAS.
- F. C. Henry, Community-Associated MRSA-Resistance and Virulence Converge, N. Engl. J. Med., 2005, 352, 1485–1487 CrossRef.
-
(a) F. D. Lowy, Staphylococcus aureus Infections, N. Engl. J. Med., 1998, 339, 520–532 CrossRef CAS;
(b) S. Y. Tong, J. S. Davis, E. Eichen-berger, T. L. Holland and V. G. Fowler Jr, Staphylococcus aureus Infections: Epidemiology, Pathophysiology, Clinical Manifestations, and Management, Clin. Microbiol. Rev., 2015, 28, 603–661 CrossRef CAS.
- H. W. Boucher and G. R. Corey, Epidemiology of Methicillin-Resistant Staphylococcus aureus, Clin. Infect. Dis., 2008, 46, S344–S349 CrossRef.
- B. Oluwatoyin, Review of Vancomycin-Induced Renal Toxicity: An Update, Ther. Adv. Endocrinol. Metab., 2016, 7, 136–147 CrossRef.
- S. W. Davies, C. A. Guidry, R. T. Petroze, T. Hranjec and R. G. Sawyer, Vancomycin and Nephrotoxicity; Just Another Myth?, J. Trauma Acute Care Surg., 2013, 75, 830–835 CrossRef CAS.
- R.-H. Song, B. Yu, D. Friedrich, J.-F. Li, H. Shen, H. Krautscheid, S.-D. Huang and M.-D. Kim, Naphthoquinone-derivative as a Synthetic Compound to Overcome the Antibiotic Resistance of Methicillin-resistant Staph. aureus, Commun. Biol., 2020, 3, 529 CrossRef CAS.
- J.-K. Huang, T.-L. Yang, C.-C. Lin and K.-S. Shia, Total Synthesis of Tetarimycin A, (±)-Naphthacemycin A9, and (±)-Fasamycin A: Structure-Activity Relationship Studies against Drug-Resistant Bacteria, J. Org. Chem., 2018, 83, 6508–6523 CrossRef CAS.
- H. Mohammad, N. S. Abutaleb and M. N. Seleem, Auranofin Rapidly Eradicates Methicillin-resistant Staphylococcus aureus (MRSA) in an Infected Pressure Ulcer Mouse Model, Sci. Rep., 2020, 10, 7251 CrossRef CAS.
-
(a) M. K. Kharel, P. Pahari, M. D. Shepherd, N. Tibrewal, S. E. Nybo, K. A. Shaaban and J. Rohr, Angucyclines: biosynthesis, mode-of-action, new natural products, and synthesis, Nat. Prod. Rep., 2012, 29, 264–325 RSC;
(b) B. Ostash, A. Korynevska, R. Stoika and V. Fedorenko, Chemistry and Biology of Landomycins, an Expanding Family of Polyketide Natural Products, Mini-Rev. Med. Chem., 2009, 9, 1040–1051 CrossRef CAS.
- T. Henkel, J. Rohr, J. Beale and L. Schwenen, Landomycins, new angucycline antibiotics from Streptomyces sp. I. Structural studies on Landomycins A-D, J. Antibiot., 1990, 43, 492–503 CrossRef CAS.
-
(a) L. Zhu, A. Luzhetskyy, M. Luzhetska, C. Mattingly, V. Adams, A. Bechthold and J. Rohr, Generation of New Landomycins with Altered Saccharide Patterns through Over-expression of the Glycosyltransferase Gene lanGT3 in the Biosynthetic Gene Cluster of Landomycin A in Streptomyces cyanogenus S-136, ChemBioChem, 2007, 8, 83–88 CrossRef CAS;
(b) B. Ostash, et al., Generation of New Landomycins by Combinatorial Biosynthetic Manipulation of the LndGT4 Gene of the Landomycin E Cluster in Strept. globisporus, Chem. Biol., 2004, 11, 547–555 CrossRef CAS;
(c) K. A. Shaaban, S. Srinivasan, R. Kumar, C. Damodaran and J. Rohr, Landomycins P-W, Cytotoxic Angucyclines from Streptomyces cyanogenus S136, J. Nat. Prod., 2011, 74, 2–11 CrossRef CAS.
- K. A. Shaaban, C. Stamatkin, C. Damodaran and J. Rohr, 11-Deoxylandomycinone and landomycins X-Z, new cytotoxic angucyclin(on)es from a Strept. cyanogenus K62 mutant strain, J. Antibiot., 2011, 64, 141–150 CrossRef CAS.
-
(a) R. Crow, B. Rosenbaum, R. Smith, Y. Guo, K. Ramos and G. Sulikowski, Landomycin A Inhibits DNA Synthesis and G1/S Cell Cycle Progression, Bioorg. Med. Chem. Lett., 1999, 9, 1663–1666 CrossRef CAS;
(b) A. Korynevska, P. Heffeter, B. Matselyukh, L. Elbling, M. Micksche, R. Stoika and W. Berger, Mechanisms Underlying the Anti-cancer Activities of the Angucycline Landomycin E, Biochem. Pharmacol., 2007, 74, 1713–1726 CrossRef CAS.
-
(a) X. Yang, B. Fu and B. Yu, Total Synthesis of Landomycin A, a Potent Antitumor Angucycline Antibiotic, J. Am. Chem. Soc., 2011, 133, 12433–12435 CrossRef CAS;
(b) X. Yang and B. Yu, Synthesis of landomycin D: studies on the saccharide assembly, Synthesis, 2016, 48, 1693–1699 CrossRef CAS.
- Synthesis of anhydrolandomycinone aglycone:
(a) D.-S. Hsu and J.-Y. Huang, Room-Temperature B(OAc)3-Promoted Diels–Alder Reaction of Juglone with Styrenes: Total Syntheses of Tetrangulol and Anhydrolandomycinone, J. Org. Chem., 2012, 77, 2659–2666 CrossRef CAS;
(b) S. Yamaguchi, H. Tanaka, R. Yamada, S. Kawauchi and T. Takahashi, Synthesis of a Landomycinone Skeleton via Masamune-Bergmann Cyclization, RSC Adv., 2014, 4, 32241–32248 RSC;
(c) D. G. Vanga and K. P. Kaliappan, A Unified Strategy for the Syntheses of Angucyclinone Antibiotics: Total Syntheses of Tetrangulol, Kanglemycin M, X-14881-E, and Anhydrolandomycinone, Eur. J. Org. Chem., 2012, 2250–2259 CrossRef CAS;
(d) C.-J. Sie, V. Patteti, Y.-R. Yang and T. K.-K. Mong, A General Strategy for Diverse Syntheses of Anhydrolandomycinone, Tetrangulol, and Landomycinone, Chem. Commun., 2018, 54, 1885–1888 RSC.
- Synthesis of 2-deoxysaccharide fragments of LAs:
(a) B. Yu and P. Wang, Efficient Synthesis of the Hexasaccharide Fragment of Landomycin A: Using Phenyl 2,3-O-Thionocarbonyl-1-thioglycosides as 2-Deoxy-β-glycoside Precursors, Org. Lett., 2002, 4, 1919–1922 CrossRef CAS;
(b) M. Zhou and G. A. O'Doherty, Enantioselective Synthesis of 2-Deoxy- and 2,3-Dideoxyhexoses, Org. Lett., 2008, 10, 2283–2286 CrossRef CAS;
(c) H. Tanaka, S. Yamaguchi, A. Yoshizawa, M. Takagi, K. Shinya and T. Takahashi, Combinatorial Synthesis of Deoxyhexasaccharides Related to the Landomycin A Sugar Moiety, Based on an Orthogonal Deprotection Strategy, Chem.–Asian J., 2010, 5, 1407–1424 CrossRef CAS;
(d) D. Zhu, K. N. Baryal, S. Adhikari and J. Zhu, Direct Synthesis of 2-Deoxy-β-Glycosides via Anomeric O-Alkylation with Secondary Electrophiles, J. Am. Chem. Soc., 2014, 136, 3172–3175 CrossRef CAS;
(e) J.-H. Ruei, P. Venukumar, A. B. Ingle and K.-K. T. Mong, C6 Picoloyl Protection: A Remote Stereodirecting Group for 2-Deoxy-β-Glycoside Formation, Chem. Commun., 2015, 51, 5394–5397 RSC;
(f) S. Yalamanchili, D. Lloyd and C. S. Bennett, Synthesis of the Hexasaccharide Fragment of Landomycin A Using a Mild, Reagent-Controlled Approach, Org. Lett., 2019, 21, 3674–3677 CrossRef CAS.
- X. Yang, P. Wang and B. Yu, Tackling the Challenges in the Total Synthesis of Landomycin A, Chem. Rec., 2013, 13, 70–84 CrossRef CAS.
- J.-C. Liu, J.-J. Fu, W.-L. Li, Y. Zou, Z.-J. Huang, J.-Y. Xu, S.-X. Peng and Y.-H. Zhang, Utilization of the Inherent Nucleophile for Regioselective O-Acylation of Polyphenols via an Intermolecular Cooperative Transesterification, Tetrahedron Lett., 2016, 72, 4103–4110 CrossRef CAS.
- For D-ring intermediate 9: F. Falchi, S. M. Bertozzi, G. Ottonello, G. F. Ruda, G. Colombano, C. Fiorelli, C. Martucci, R. Bertorelli, R. Scarpelli, A. Cavalli, T. Bandiera and A. Armirotti, A New Kernal-Based, Partial Least Squares, QSRR Model for UPLC Retention Time Prediction: A Useful Tool for Metabolite Identification, Anal. Chem., 2016, 88, 9510–9517 CrossRef CAS.
- S. Pulley and B. Czakó, Formal Total Synthesis of Shikonin via Dötz Benzannulation, Tetrahedron Lett., 2004, 45, 5511–5514 CrossRef CAS.
- A. Wohl, Bromination of Unsaturated Compounds with N-Bromosuccinimide, a Contribution to the Theory of the Course of Chemical Processes, Eur. J. Inorg. Chem., 1919, 51–63 CAS.
- V. Snieckus, Directed-ortho Metalation. Tertiary Amide and O-Carbamate Directors in Synthetic Strategies for Polysubstituted Aromatic, Chem. Rev., 1990, 90, 879–933 CrossRef CAS.
-
(a) K. H. Dötz, Synthesis of the Naphthol Skeleton from Pentacarbonyl-[methoxy(phenyl)carbene]chromium (O) and To-lan, Angew. Chem., Int. Ed., 1975, 14, 644–645 CrossRef;
(b) R. Fernandes and S. Mulay, Chiral Cups (Calixarenes) via Dötz Benzannulation, Synthesis, 2014, 46, 1836–1846 CrossRef;
(c) T. Bera, K. Pandey and R. Ali, The Dötz Benzannulation Reaction: A Booming Methodology for Natural Product Synthesis, ChemistrySelect, 2020, 5, 5239–5267 CrossRef CAS.
- L.-C. Campeau, M. Parisien, A. Jean and K. Fagnou, Catalytic Direct Arylation with Aryl Chlorides, Bromides, and Iodides: Intramolecular Studies Leading to New Intermolecular Reactions, J. Am. Chem. Soc., 2006, 128, 581–590 CrossRef CAS.
- H. R. Billica and H. Adkins, Catalyst, Raney Nickel, W-6, Org. Synth., 1949, 29, 24 CrossRef CAS and references cited therein.
- For building block 19: O. Calin, R. Pragani and P. H. Seeberger, De Novo Synthesis of L-Colitose and L-Rhodinose Building Blocks, J. Org. Chem., 2012, 77, 870–877 CrossRef CAS.
- For glucal 23: W. Roth and W. Pigman, D-Glucal and the Glycals. D-Glucal and 6-Deoxy-L-Dlucal, Methods Carbohydr. Chem., 1963, 405–408 CAS.
- S.-N. Lam and J. Gervay-Hague, Efficient Route to 2-Deoxy β-O-Aryl-D-glycosides via Direct Displacement of Glycosyl Iodides, Org. Lett., 2003, 5, 4219–4222 CrossRef CAS.
- W. R. Roush and C. E. Bennett, A Highly Stereoselective Synthesis of 2-Deoxy-β-Glycosides using 2-Deoxy-2-Iodo-Glucopyranosyl Acetate Donors, J. Am. Chem. Soc., 1999, 121, 3541–3542 CrossRef CAS.
- C. J. Goodwin, S. J. Holt, S. Downes and N. J. Marshall, Microculture Tetrazolium Assays: a Comparison between Two New Tetrazolium Salts, XTT and MTS, J. Immunol. Methods, 1995, 179, 95–103 CrossRef CAS.
- Promega, CellTiter96 AQueous Non-Radioactive Cell Proliferation Assay: Technical Bulletin No 169, Promega Corporation, Madison, USA, 2001 Search PubMed.
- CLSI, Methods for Dilution Antimicrobial Susceptibility Tests for Bacteria that Grow Aerobically, CLSI standard M07, Clinical and Laboratory Standards Institute, Wayne, PA, 11th edn, 2018 Search PubMed.
- F.-J. Chen, T.-L. Lauderdale, C.-H. Lee, Y.-C. Hsu, I.-W. Huang, P.-C. Hsu and C.-S. Yang, Effect of a Point Mutation in mprF on Susceptibility to Daptomycin, Vancomycin, and Oxacillin in an MRSA Clinical Strain, Front. Microbiol., 2018, 9, 1086 CrossRef.
Footnotes |
† Electronic supplementary information (ESI) available: Schemes S1 and S2 for preparation of 19, 20, and 22, Table S1 for comparison of NMR data, raw data of MTS, and MIC assays, experimental procedures, and NMR spectroscopic data and spectra were given. See DOI: 10.1039/d1ra01088c |
‡ These authors contributed equally. |
|
This journal is © The Royal Society of Chemistry 2021 |
Click here to see how this site uses Cookies. View our privacy policy here.