DOI:
10.1039/D1RA01004B
(Paper)
RSC Adv., 2021,
11, 10610-10614
Heteropolyacid ionic liquid heterogeneously catalyzed synthesis of isochromans via oxa-Pictet–Spengler cyclization in dimethyl carbonate†
Received
6th February 2021
, Accepted 2nd March 2021
First published on 11th March 2021
Abstract
A recyclable and efficient heterogeneous, green catalyst based on the synthesis of Keggin-type polyoxometalate (H3PMo12O40) and vitamin B1 analogue 3-ethyl-5-(2-hydroxyethyl)-4-methylthiazol-3-ium (HEMT), i.e., [HEMTH]H2[PMo12O40] was prepared. Oxa-Pictet–Spengler cyclization of arylethanols and aldehydes were catalyzed to afford various substituted isochromans in moderate conditions with excellent yields using dimethyl carbonate (DMC) as a green solvent. Furthermore, this protocol was applicable in a gram-scale reaction, and the catalyst could be recycled eight times without significant loss of activity.
Introduction
Green chemistry has been widely advocated for the reasons of economic development, environmental degradation, resource consumption and so on. In particular, green catalytic chemistry has received widespread attention. As part of our interest in the field of polyoxometalates (POMs) and green chemistry, POMs have played vital roles in organic synthesis as acid–base catalysts for the past few decades.1–7 Among those, the application of noncorrosive, inexpensive, and readily available Keggin-type heteropolyacids (HPAs) for organic transformations including condensation, cycloaddition and coupling reactions has been extensively studied.8–14 However, HPAs are generally homogeneous catalysts in most of the reported systems. Since homogeneous catalysts are difficult to recycle, there is an urgent need to develop a recyclable heterogeneous catalytic system based on HPAs from the perspective of environmental sustainability.15–21
Ionic liquids (ILs) have drawn considerable attention among various heterogeneous designs of HPAs due to their non-volatility, high thermal stability, and excellent catalytic activity. In the past few decades, the counter-cation precursors of some typical HPAs-based ionic liquids have been designed as pyridine, 1-methyl imidazole, and 1-methyl-2-pyrrolidinone, etc.22–27 Our group is committed to developing HPA-based ionic liquids for green synthesis. In the previous work, our group synthesized [HMTH]2H2[SiW12O40] using H4SiW12O40 and the analogue compound 5-(2-hydroxyethyl)-4-methylthiazole (HMT) of vitamin B1 as the counter-cation precursor.28 Various polysubstituted olefins were synthesized by the dehydration of alcohols with alcohols (or alkenes) in a green solvent dimethyl carbonate (DMC) catalyzed by [HMTH]2H2[SiW12O40]. We are making attempts to further develop this catalytic system for more valuable reactions, for example, the synthesis of isochromans from arylethanols and aldehydes via oxa-Pictet–Spengler cyclization (Scheme 1a).
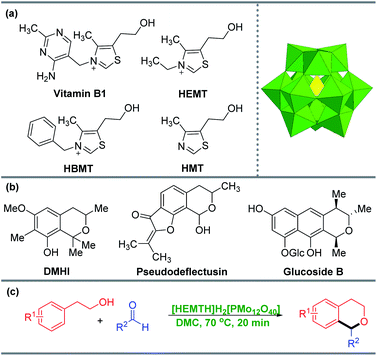 |
| Scheme 1 (a) Precursors for HPA-based ionic liquids; (b) representative examples of isochromans with biological activities; (c) synthesis of substituted isochromans via oxa-Pictet–Spengler cyclization (this work). | |
Isochroman is a prominent structure motif and is the core structure of numerous bioactive natural products, such as 6-methoxy-1,1,3,7-tetramethylisochroman-8-ol (DMHI), glucoside B and aspergillus pseudodeflectus (Scheme 1b), which are valuable biologically and pharmaceutically active organic molecules.29–31 Due to the importance of isochromans, the synthesis of isochromans have undoubtedly received considerable attention, and the synthetic methods of isochromans have achieved greatly development.32,33 There are two important methods for the synthesis of isochromans, including the cyclo-dehydration of homophthalyl alcohols and the cyclization of phenylethanol which is called oxa-Pictet–Spengler reaction.34,35 The catalysts are used in the latter are usually Fe(OTf)2, Bi(OTf)3, SnCl4 and other expensive Lewis acid, or HCl, TfOH and other corrosive Bronsted acid (TfOH = trifluoromethanesulfonic acid).33,36–40 And the solvents are toluene, benzene, dioxane and so on.41–45 These methods show disadvantages such as long reaction times, high temperature, and unrecyclable catalysts. Therefore, it is urgent to develop a green and efficient catalytic system for the synthesis of isochromans.
Based on our previous research on the application of HPAs-based ionic liquids in various reactions, we envisioned that the application of inexpensive, noncorrosive, acidic HPAs-based ionic liquids could be a green, practical and efficient strategy for the synthesis of isochromans via oxa-Pictet–Spengler reaction. Herein, we report a practical protocol to synthesize isochromans under the mild conditions catalysized by [HEMTH]H2[PMo12O40] (Scheme 1c). This catalyst could be easily recycled at least 8 times without significantly reducing activity or selectivity.
Results and discussion
To optimize the optimal reaction conditions, we carried out our study by the reaction of 2-(3,4-dimethoxyphenyl)ethan-1-ol 1a and benzaldehyde 2a in dichloroethane (DCE) at 50 °C for 10 min using different HPAs (3 mol%), i.e., H3PMo12O40, H3PW12O40, H4SiW12O40 as catalyst (Fig. 1A). First, the catalytic effects of HPAs was investigated. As shown in Fig. 1B, H3PMo12O40 was the best catalyst for the reaction and the yield of product 3a was 80%. 54% and 43% yields of 3a was obtained in the cases of H3PW12O40 and H4SiW12O40 as catalysts. No yield of 3a was observed when the reaction was performed in the absence of catalyst. Among those HPAs, H3PMo12O40 shows the weakest acidity but the softest heteropoly anion. Subsequently, H3PMo12O40 was applied as optimal catalyst to screen the solvent. Some common solvents were examined, and the effect of some green solvents on the reaction has been mainly studied including propylene carbonate (PC), ethylene carbonate (EC), cyclopentyl methyl ether (CPME), dimethyl carbonate (DMC), H2O. As shown in Fig. 1C, no yield was observed when the reaction was conducted in H2O. The reaction in PC and EC delivered 3a in 54% and 35% yield, respectively. While 3a can be obtained with good yields in DMC and CPME. In particular, 3a could be obtained in 89% yield when DMC was used as solvent (see ESI† for details). Subsequently, [HMTH]H2[PMo12O40] (ILs-1), [HEMTH]H2[PMo12O40] (ILs-2), and [HBMTH]H2[PMo12O40] (ILs-3) were prepared using 5-(2-hydroxyethyl)-4-methylthiazole (HMT), 3-ethyl-5-(2-hydroxyethyl)-4-methylthiazol-3-ium (HEMT), 3-benzyl-5-(2-hydroxyethyl)-4-methylthiazol-3-ium (HBMT) and H3PMo12O40 as the raw materials by a procedure similar to the literature.28 Accordingly, [HEMTH]2H[PMo12O40] (ILs-4) and [HEMTH]3[PMo12O40] (ILs-5) were prepared using 2 mmol and 3 mmol of HEMT and H3PMo12O40 (1 mmol), respectively (IR characterization is shown in Fig. S1†). All of the ionic liquids were used as catalysts in the condensation reaction. It is clear that ILs-2 exhibits better catalytic activity than other ionic liquids as shown in Fig. 1D.
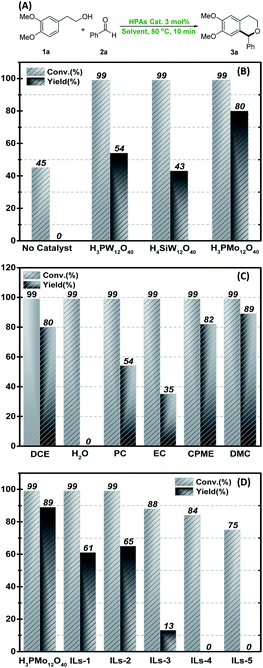 |
| Fig. 1 (A) Oxa-Pictet–Spengler reaction of 1a and 2a; (B) conversation and yield profile for the examination of HPAs catalysts (DCE as solvent, Table S1†); (C) conversation and yield profile for the optimization of the reaction solvents (H3PMo12O40 as catalyst, Table S2†); (D) conversation and yield profile for the examination of HPAs-based ionic liquids (DMC as solvent, Table S3†) (yields were determined by GC using biphenyl as an internal standard). | |
Then, we studied the influence of reaction temperature, time and catalyst loading on the reaction. As shown in Fig. 2, the conversion of the substrate could reach 99% at 30 °C, but the selectivity is relatively low. When the temperature was risen, the yield of the desired product 3a could be obtained 85% at 70 °C (Fig. 2A). As can be seen from Fig. 2B, with the increase of the reaction time, the yield of the desired product 3a gradually increased to 91% in 20 min. To further improve the yield of desired product 3a, the catalyst loading in the reaction was studied. It can be found that the yield of the product 3a gradually increased to 95% with the increase of the catalyst loading up to 4 mol% (Fig. 2C). Finally, the optimal condition for the oxa-Pictet–Spengler reaction of 2-(3,4-dimethoxyphenyl)ethan-1-ol 1a and benzaldehyde 2a was established when 4 mol% [HEMTH]H2[PMo12O40] was used as catalyst in DMC at 70 °C for 20 min.
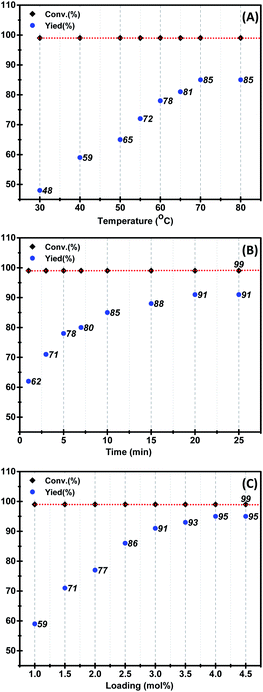 |
| Fig. 2 (A) Investigation of the reaction temperature ([HEMTH]H2PMo12O40 as catalyst, DMC as solvent, 10 min, Table S4†); (B) optimization of the reaction time (70 °C, Table S5†); (C) examination of the loading of [HEMTH]H2[PMo12O40] (DMC as solvent, 70 °C, 20 min, Table S6†) (yields were determined by GC using biphenyl as an internal standard). | |
With the optimized reaction conditions in hand, we examined the scope of the method and found that it proceeded well with various substituted aldehydes. This reaction system was not only suitable for aromatic aldehydes, but also for aliphatic aldehydes (Table 1). As can be seen from the table, 2-(3,4-dimethoxyphenyl)ethan-1-ol reacted with arylaldehydes bearing electron-withdrawing groups, such as –F, –Cl, –Br, –NO2, –CN, could afford isolated yield of the corresponding isochroman products over 92% (92–96%) 92% (92–96%) (3b–3i, 3o). While arylaldehydes with electron-donating groups, such as –CH3, –OCH3, –OCH2CH3, –CH(CH3)2, could provide isolated yield of the corresponding isochroman products in up to 95% (91–95%) (3j–3n, 3p). The reaction was carried out using ortho-, meta-, and para-substituted aromatic aldehydes as reaction substrate. As shown in Table 1, affected by steric hindrance, the yields of the desired products obtained from para-aldehydes was higher than that of meta-aldehydes, while the yields from meta-aldehydes was higher than the desired product obtained from ortho-aldehydes (3c > 3d > 3e, 3g > 3h > 3i, 3j > 3k > 3l). Particularly, aliphatic aldehydes were proved to be also equally applicable to this catalytic system and afford isochromans in high isolated yields (3r–3u), including butyraldehyde, pentanal, hexaldehyde and phenylacetaldehyde. Meanwhile, phenethyl alcohol was applied to test the reaction activity under the optimal catalytic conditions, it could be found that in case of non-activated phenethyl alcohol which had no activating substituents on phenyl ring, the reaction did not occur as obviously as expected. As long as there was an activated functional group on the benzene ring, the reaction could proceed well. For example, 2-(3-methoxyphenyl)ethan-1-ol reacted with benzaldehyde under optimal reaction conditions and 94% of the corresponding desired product 3q was obtained.
Table 1 [HEMTH]H2[PMo12O40]-catalyzed oxa-Pictet–Spengler reaction of arylethanols and aldehydes to access substituted isochromansa
Reaction conditions: 1 (0.6 mmol), 2 (0.66 mmol), [HEMTH]H2[PMo12O40] (4 mol%), DMC (3 mL), isolated yields. |
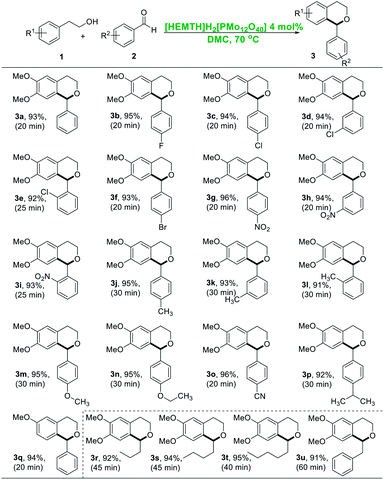 |
Additionally, it had been found that the catalytic system was easy to scale-up at gram-scale to synthesize the substituted isochromans via the direct oxa-Pictet–Spengler reaction of arylethanols and aldehydes, which is quite important in terms of sustainability and practical application. As can be seen from Scheme 2, the reaction of 1a (6 mmol, 1.09 g) and 2a (6.6 mmol, 0.70 g) generated the desired products 3a in 91% of isolated yield catalyzed by [HEMTH]H2[PMo12O40] (4 mol%) in DMC at 70 °C for 1 h (Scheme 2A). The gram-scale ring condensation reaction of 1a and 2g generated the product 3g in yield of 93% under identical conditions (Scheme 2B). Similarly, the reaction of 1b and 2a could produce the corresponding product 3q in 90% yield at the same reaction conditions as above (Scheme 2C).
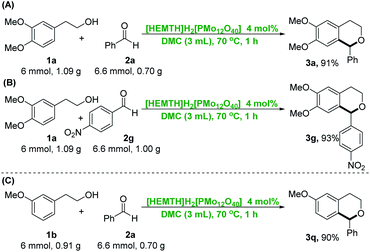 |
| Scheme 2 Gram-scale synthesis of substituted isochromans. | |
To test the reusability of the catalyst, the model reaction of 2-(3,4-dimethoxyphenyl)ethan-1-ol 1a and benzaldehyde 2a was carried out in the presence of 10 mol% of [HEMTH]H2[PMo12O40] under the optimal reaction conditions. As shown in Fig. 3A, the ionic liquid [HEMTH]H2[PMo12O40] could be recycled at least 8 times without significant loss of activity. And after 8 cycles of reactions, XRD comparisons of the catalyst [HEMTH]H2[PMo12O40] before and after the reaction were performed, and it was found that the catalyst did not change, which further confirmed that the catalyst still has catalytic activity (Fig. 3B).
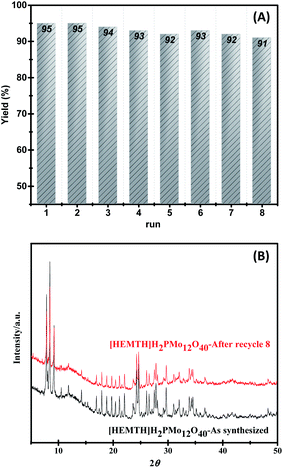 |
| Fig. 3 (A) Recyclability of [HEMTH]H2PMo12O40 in oxa-Pictet–Spengler reaction of 1a and 2a (yields were determined by GC using biphenyl as an internal standard); (B) XRD characterization before and after catalyst recycling. | |
According to literatures,35,37,38 a possible reaction mechanism was inferred as shown in Scheme 3. First, the aldehyde 2 was activated by ILs-2 to form A, and then the activated aldehyde A was attacked by two different positions of alcohol 1 to form B and C. Then B underwent an intramolecular Friedel–Crafts reaction and closed the ring to form the desired product 3, while C underwent an intramolecular dehydration to form the isochroman derivative.
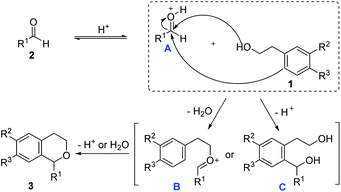 |
| Scheme 3 The proposed mechanism for the oxa-Pictet–Spengler. | |
Conclusions
In conclusion, we successfully developed the [HEMTH]H2PMo12O40/DMC green, recyclable and efficient heterogeneous catalytic system for the synthesis of isochromans from arylethanols and aldehydes via oxa-Pictet–Spengler cyclization in excellent yields. Furthermore, the catalytic system has the advantages of high yield, low catalyst loading, and short reaction time. It is worth noting that the reaction could be scaled up and the heterogeneous catalyst could be reused at least 8 times without significant loss of activity. Such findings may pave the way for the development of new green chemistry transformations.
Experimental
Typical procedure for direct oxa-Pictet–Spengler reaction of arylethanols with aldehydes
To a 4 mL reaction vial, 2-(3,4-dimethoxyphenyl)ethan-1-ol (0.6 mmol), benzaldehyde (0.66 mmol), [HEMTH]H2[PMo12O40] (4 mol%) and DMC (3 mL) were added. Then the reaction was carried out in screw cap vials with a Teflon seal at 70 °C for desired time. After cooling to room temperature, the mixture was further purified by column chromatography (petroleum ether/EtOAc) to afford the desired products (see the ESI† for details).
Disposal of catalyst in cyclic experiment
The reaction system was centrifuged, the supernatant was removed, the precipitation part (catalyst) was washed five times with ethyl acetate, dried under vacuum at 50 °C for 2 h, and then put into the next cycle again.
Conflicts of interest
There are no conflicts to declare.
Acknowledgements
Financial support from the Research Found of East China University of Technology (no. DHBK2019265, DHBK2019267, DHBK2019264), the Open Fund of the Jiangxi Province Key Laboratory of Synthetic Chemistry (JXSC202008), Natural Science Foundation of Jiangxi Province, China (20202BABL203004), National Natural Science Foundation of China (21804019, 22001034) are gratefully acknowledged.
Notes and references
- G. P. Yang, D. Dilixiati, T. Yang, D. Liu, B. Yu and C. W. Hu, Appl. Organomet. Chem., 2018, 32, e4450 CrossRef.
- G.-P. Yang, S.-X. Shang, B. Yu and C.-W. Hu, Inorg. Chem. Front., 2018, 5, 2472–2477 RSC.
- D. E. Katsoulis, Chem. Rev., 1998, 98, 359–388 CrossRef CAS.
- S. S. Wang and G. Y. Yang, Chem. Rev., 2015, 115, 4893–4962 CrossRef CAS PubMed.
- M.-Y. Wang, R. Ma and L.-N. He, Sci. China: Chem., 2016, 59, 507–516 CrossRef CAS.
- T. Uematsu, Y. Miyamoto, Y. Ogasawara, K. Suzuki, K. Yamaguchi and N. Mizuno, Catal. Sci. Technol., 2016, 6, 222–233 RSC.
- J. Zhang, Y. Huang, G. Li and Y. Wei, Coord. Chem. Rev., 2019, 378, 395–414 CrossRef CAS.
- G. P. Yang, X. He, B. Yu and C. W. Hu, Appl. Organomet. Chem., 2018, 32, e4532 CrossRef.
- X. Feng, T. Yang, X. He, B. Yu and C. W. Hu, Appl. Organomet. Chem., 2018, 32, e4314 CrossRef.
- G. P. Yang, N. Zhang, N. N. Ma, B. Yu and C. W. Hu, Adv. Synth. Catal., 2017, 359, 926–932 CrossRef CAS.
- G. Yang, Y. Liu, K. Li, W. Liu, B. Yu and C. Hu, Chin. Chem. Lett., 2020, 31, 3233–3236 CrossRef CAS.
- K. Suzuki, J. Jeong, K. Yamaguchi and N. Mizuno, New J. Chem., 2016, 40, 1014–1021 RSC.
- Q. Yue, Y. Lu, Z. Zhang, H. R. Tian, H. N Wang, X. H Li and S. X Liu, New J. Chem., 2020, 44, 16913–16920 RSC.
- Y. Leng, J. Wang, D. Zhu, M. Zhang, P. Zhao, Z. Long and J. Huang, Green Chem., 2011, 13, 1636–1639 RSC.
- L.-H. Lu, S.-J. Zhou, M. Sun, J.-L. Chen, W. Xia, X. Yu, X. Xu and W.-M. He, ACS Sustainable Chem. Eng., 2018, 7, 1574–1579 CrossRef.
- C. Wu, H.-J. Xiao, S.-W. Wang, M.-S. Tang, Z.-L. Tang, W. Xia, W.-F. Li, Z. Cao and W.-M. He, ACS Sustainable Chem. Eng., 2019, 7, 2169–2175 CrossRef CAS.
- G. Cui, Y. Li, J. Liu, H. Wang, Z. Li and J. Wang, ACS Sustainable Chem. Eng., 2018, 6, 15292–15300 CrossRef CAS.
- B. Yu, B. B. Cheng, W. Q. Liu, W. Li, S. S. Wang, J. Cao and C. W. Hu, Adv. Synth. Catal., 2016, 358, 90–97 CrossRef CAS.
- X. Huang, X. Zhang, D. Zhang, S. Yang, X. Feng, J. Li, Z. Lin, J. Cao, R. Pan and Y. Chi, Chem.–Eur. J., 2014, 20, 2557–2564 CrossRef CAS.
- N. Mizuno and M. Misono, Chem. Rev., 1998, 98, 199–218 CrossRef CAS PubMed.
- M. M. Heravi, M. Vazin Fard and Z. Faghihi, Green Chem. Lett. Rev., 2013, 6, 282–300 CrossRef CAS.
- G.-P. Yang, N. Jiang, X.-Q. Huang, B. Yu and C.-W. Hu, Mol. Catal., 2019, 468, 80–85 CrossRef CAS.
- M.-Y. Wang, Q.-W. Song, R. Ma, J.-N. Xie and L.-N. He, Green Chem., 2016, 18, 282–287 RSC.
- C. Wu, L.-H. Lu, A.-Z. Peng, G.-K. Jia, C. Peng, Z. Cao, Z. Tang, W.-M. He and X. Xu, Green Chem., 2018, 20, 3683–3688 RSC.
- L.-Y. Xie, S. Peng, L.-H. Lu, J. Hu, W.-H. Bao, F. Zeng, Z. Tang, X. Xu and W.-M. He, ACS Sustainable Chem. Eng., 2018, 6, 7989–7994 CrossRef CAS.
- A. S. Amarasekara, Chem. Rev., 2016, 116, 6133–6183 CrossRef CAS PubMed.
- X.-Y. Li, S.-S. Zheng, X.-F. Liu, Z.-W. Yang, T.-Y. Tan, A. Yu and L.-N. He, ACS Sustainable Chem. Eng., 2018, 6, 8130–8135 CrossRef CAS.
- G.-P. Yang, X. Wu, B. Yu and C.-W. Hu, ACS Sustainable Chem. Eng., 2019, 7, 3727–3732 CrossRef CAS.
- R. Bernini, F. Crisante, F. D'Acunzo, P. Gentili and E. Ussia, New J. Chem., 2016, 40, 3314–3322 RSC.
- D. W. Cameron, R. I. T. Cromartie, D. G. I. Kingston and A. R. Todd, J. Chem. Soc., 1964, 51 RSC.
- J. Peng, F. Lu and J. Ralph, Phytochemistry, 1999, 50, 659–666 CrossRef CAS.
- E. L. Larghi and T. S. Kaufman, Eur. J. Org. Chem., 2011, 2011, 5195–5231 CrossRef CAS.
- A. Yokoyama, T. Ohwada and K. Shudo, J. Org. Chem., 1999, 64, 611–617 CrossRef CAS.
- A. Saeed, Chin. Chem. Lett., 2010, 21, 261–264 CrossRef CAS.
- A. Saito, M. Takayama, A. Yamazaki, J. Numaguchi and Y. Hanzawa, Tetrahedron, 2007, 63, 4039–4047 CrossRef CAS.
- J. Zhou, C. Wang, D. Xue, W. Tang, J. Xiao and C. Li, Tetrahedron, 2018, 74, 7040–7046 CrossRef CAS.
- A. Hegedüs and Z. Hell, Org. Biomol. Chem., 2006, 4, 1220–1222 RSC.
- M. Guiso, C. Marra and C. Cavarischia, Tetrahedron Lett., 2001, 42, 6531–6534 CrossRef CAS.
- D. A. Bianchi, N. E. Blanco, N. Carrillo and T. S. Kaufman, J. Agric. Food Chem., 2004, 52, 1923–1927 CrossRef CAS PubMed.
- C. Lherbet, D. Soupaya, C. Baudoin-Dehoux, C. André, C. Blonski and P. Hoffmann, Tetrahedron Lett., 2008, 49, 5449–5451 CrossRef CAS.
- C. N. Eid, J. Shim, J. Bikker and M. Lin, J. Org. Chem., 2009, 74, 423–426 CrossRef CAS PubMed.
- E. L. Larghi and T. S. Kaufman, Synthesis, 2006, 2006, 187–220 Search PubMed.
- J. J. Maresh, S. O. Crowe, A. A. Ralko, M. D. Aparece, C. M. Murphy, M. Krzeszowiec and M. W. Mullowney, Tetrahedron Lett., 2014, 55, 5047–5051 CrossRef CAS.
- B. Bouguerne, C. Lherbet and M. Baltas, Lett. Org. Chem., 2010, 7, 420–423 CrossRef CAS.
- M. Guiso, A. Bianco, C. Marra and C. Cavarischia, Eur. J. Org. Chem., 2003, 3407–3411 CrossRef CAS.
Footnote |
† Electronic supplementary information (ESI) available. See DOI: 10.1039/d1ra01004b |
|
This journal is © The Royal Society of Chemistry 2021 |