DOI:
10.1039/D1RA00900A
(Paper)
RSC Adv., 2021,
11, 14148-14153
Structure transition of a C60 monolayer on the Bi(111) surface
Received
3rd February 2021
, Accepted 31st March 2021
First published on 15th April 2021
Introduction
C60 molecule, as a prototypical fullerene molecule, has attracted widespread attention due to its potential in endohedral fullerenes,1 photovoltaic devices,2 peapod nanotubes,3 and single-molecule transistors.4 A C60 monolayer grown on solid surfaces is critical for understanding and controlling the interfacial properties of fullerene-derived electronic and photovoltaic devices.5,6 STM studies demonstrated that the C60 monolayer on the solid surface exhibit a variety of lattice orientations such as the “in phase” (2√3 × 2√3) R30°,7–13, (7 × 7) R0°13 and (√589 × √589) R14.5°.13–15 The individual molecules of fullerene and fulleride within a single domain display different orientations. In the complex orientational ordering (7 × 7) R0° structure, a 7-molecule C60 cluster consists of a central molecule sitting atop of a gold atom and six tilted surrounding molecules.10 In the unit cell of the (√589 × √589) R14.5° structure, 49 C60 molecules adopt 11 different orientations.14 In the (2√3 × 2√3) R30° structure, all C60 molecules are in the same orientation.12,16 The complex chiral motifs have been observed.17 In CsnC60 fulleride films, orientational ordering appears.18 Moreover, “bright” and “dim” molecules have been widely found in the C60 monolayer.9–17 However, the “dim” molecules in superstructures reported so far arrange irregularly.
The structure of C60 monolayers grown on the solid surface is not only related to C60 molecules themselves but also the substrate. In the past reports, there have been a large number of investigation on the C60 monolayer structures grown on numerous metals or semiconducting substrates, such as Ag,7–9,19,20 Au,10–16,21,22 Cu,23–25 graphene,26,27 Si,28,29 Ge,30 C60,29 or NaCl.31 However, few reports address the superstructure of C60 molecules adsorbed on semi-metal substrates. It is found that thin films of organic molecules grown on a semi-metallic Bi(111) surface shows a lot of interesting phenomena, such as the ordered crystalline layer with the standing-up orientation of pentacene molecules,32 the chiral self-assembly of rubrene molecules,33 structural transitions in different monolayers of cobalt phthalocyanine films,34 and the Moire' pattern in C60 thin films.35
In this study, we use Bi(111) as the substrate and studied the structure transition of the C60 monolayer. C60 molecules were deposited at 100 K form local-order structures. When the deposition temperature increased to room temperature, the local-order structures turn into a long-range ordered (√93 × √93) R20° superstructure. After annealing at 400 K, the ordered superstructure transforms into the (11 × 11) R0° superstructure. These superstructures are different from the structures of the C60 monolayer reported so far. Furthermore, the individual C60 molecules in the local-order structure, (√93 × √93) R20° and (11 × 11) R0° superstructure, show the 6
:
6 C–C bond and 5
:
6 C–C bond facing-up, mixed orientations, and hexagon facing-up, respectively. The 6
:
6 (5
:
6) C–C bond indicates the common side of two adjacent hexagons (pentagon and hexagon) in C60 molecules.
Experimental
The experiments were conducted in an ultra-high vacuum low-temperature scanning tunneling microscope produced by Unisoku. The base pressure was kept at ∼1.2 × 10−10 Torr. An Si(111) substrate was continuously degassed at ∼870 K for 8 h with subsequent flashing to 1400 K for several seconds. The Bi(111) film was prepared by depositing 20 monolayers of bismuth atoms on a Si(111)-7 × 7 surface at room temperature with subsequent annealing at 400 K.36 C60 molecules were deposited onto the Bi(111) surface by heating the tantalum cell to 700 K. The growth rate of C60 molecules was about 0.4 monolayers per minute. All STM images were acquired with a tungsten tip in constant-current mode at liquid nitrogen temperature (78 K).
Results and discussion
First, a small number of C60 molecules were deposited onto the Bi(111) surface when the substrate was maintained at 100 K. Fig. 1(a) shows the atomic-resolution image of the hexagonal lattices of the Bi(111) thin film. The lattice constants of the Bi(111) surface are measured to be a1 = a2 = 0.45 ± 0.02 nm, very close to the bulk value (a = 0.454 nm) in Bi crystals.36 Fig. 1(b) shows the isolated C60 molecules on the Bi(111) surface presenting round protrusions. When reducing the bias voltage, the round protrusions are separated into two asymmetrical [Fig. 1(c)] or symmetrical [Fig. 1(d)] lobes, corresponding to the two different adsorption configurations, 5
:
6 C–C bond facing-up and 6
:
6 C–C bond facing-up, similar to C60 molecules on Au(111).12 This indicates that there are two stable adsorption orientations of isolated C60 molecules on the Bi(111) substrate, 6
:
6 C–C bond, and 5
:
6 C–C bond facing-up.
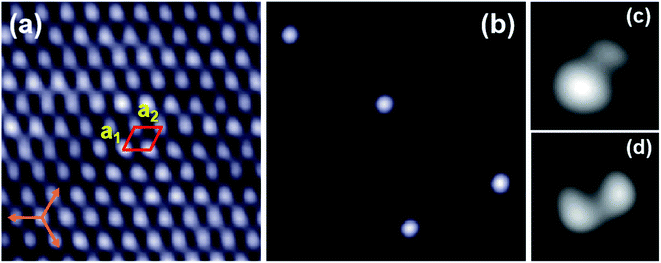 |
| Fig. 1 The initial stage of C60 molecules adsorbed on the Bi(111) surface. (a) Hexagonal lattices of the Bi(111) surface, 5 nm × 5 nm, −0.1 V. The unit cell is marked with a red box and the orange arrows indicate the directions of the Si(111) substrate. (b) Isolated C60 molecules adsorbed on Bi(111), 20 nm × 20 nm, 2.2 V. (c) STM image of an isolated C60 molecule with two asymmetrical lobes corresponding to the 5 : 6 C–C bond facing up, 1.3 nm × 1.3 nm, 400 mV. (d) STM image of an isolated C60 molecule with two symmetrical lobes corresponding to the 6 : 6 C–C bond facing up, 1.3 nm × 1.3 nm, 200 mV. | |
When the coverage increases, C60 molecules form the close-packed hexagonal structure, as shown in Fig. 2. We noticed that all the C60 molecules present a uniform height, except a few dim molecules (marked by green dotted circles). The brightness contrast in images stems from the different adsorption sites of C60 molecules. It is well known that metal surfaces do not behave as rigid templates for the chemisorption of C60 molecules, but may reconstruct substantially to accommodate the molecules.37 We speculate that Dim C60 molecules are located at the vacancies of the Bi(111) substrate, originating from the reconstruction of the Bi(111) surface, similar to C60 molecules on Au(111)16 and Cu(111).38
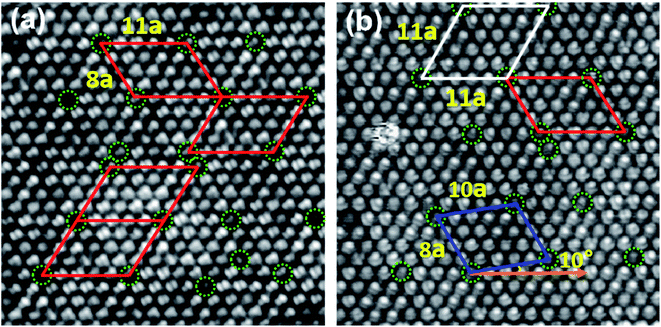 |
| Fig. 2 Local-order structure in the monolayer C60 grown at a low temperature (∼100 K). (a) Four unit cells of the (11 × 8) R0° superstructure appeared in the C60 monolayer, 20 nm × 20 nm, −1.2 V. The dim C60 molecules, located at the hollow position of Bi(111), are marked by the green dotted circles. (b) The mixture of three types of superstructures, 20 nm × 20 nm, −0.9 V. The red, white, and blue unit cells correspond to the superstructure (11 × 8) R0°, (11 × 11) R0°, and (10 × 8) R10°. | |
According to the arrangement of bright and dim molecules, we can see some local-order structures, though there is a lack of long-range ordering. In Fig. 2(a), there is an (11 × 8) R0° local-order structure (marked by red parallelogram). The lattice directions of (11 × 8) R0° are along with the directions of Bi(111), and the measured lattice constants are 5.00 ± 0.02 nm and 3.64 ± 0.02 nm, corresponding to 11 and 8 times of the lattice constant of the Bi(111) surface. The lattice directions of Bi(111) were obtained on the surface, which was not covered with C60 molecules. In another domain, shown in Fig. 2(b), the local-order structure is mixed with three types of structures, namely (11 × 8) R0° (red quadrilateral), (11 × 11) R0° (white quadrilateral), and (10 × 8) R10° (blue quadrilateral). In particular, we noticed that C60 molecules exhibit almost the same orientation in a single domain, and most of the individual C60 molecules in the local-order structure adopt two favorite orientations (6
:
6 C–C bond and 5
:
6 C–C bond facing up) as the isolated molecules on Bi(111). For example, most of the molecules shown in Fig. 2(a) present two symmetrical lobes, corresponding to C60 molecules with a 6
:
6 C–C bond facing up. However, in Fig. 2(b), the molecules present two asymmetric lobes, corresponding to the 5
:
6 C–C bond facing up. We suggest that the formation of a local-order structure is due to the low-temperature growth. Because of the low kinetic energy of C60 molecules at 100 K, molecular mobility is not high enough to form a long-range ordered superstructure. The C60 molecules adsorbed on Bi(111) adopt their preferred orientations (6
:
6 C–C bond and 5
:
6 C–C bond facing up), similar to the isolated molecules adsorbed on the substrate. This proves the strong molecule–substrate interaction in the local-order structure.
To investigate the influence of temperature on the structure, we deposited C60 molecules on Bi(111) at room temperature. It is found that C60 molecules aggregate into a hexagonal structure, the same as C60 molecules in the local-order structure. However, the local-order structures, originating from the dim and bright molecules, turn into a long-range ordered (√93 × √93) R20° superstructure [Fig. 3(a)]. This superstructure is different from the structures of the C60 monolayer reported so far. There is a misorientation angle of 20° between the lattice directions of the C60 monolayer and the Bi(111) surface. The measured lattice constants of (√93 × √93) R20° are b1 = b2 = 4.38 ± 0.02 nm, agreeing well with √93 times the lattice constant of Bi(111) (0.45 nm). Fig. 3(b) shows the schematic of the (√93 × √93) R20° superstructure. Based on the lattice constant of the Bi(111) substrate, the lattice vectors of the (√93 × √93) R20° superstructure can be expressed as following matrixes:
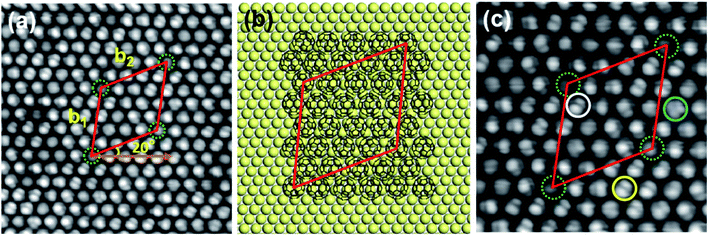 |
| Fig. 3 (a) The STM image of the (√93 × √93) R20° superstructure, 15 nm × 15 nm, −1.2 V. (b) Schematic model of the (√93 × √93) R20° superstructure. The yellow balls and black hollow balls represent Bi atoms and C60 molecules. (c) High-resolution STM image of the (√93 × √93) R20° superstructure, 10 nm × 10 nm, −1.0 V. The individual molecules exhibit different orientations, such as 5 : 6 C–C bond, 6 : 6 C–C bond, and hexagon facing up, marked by white, yellow, and blue solid circles, respectively. | |
This ordered superstructure implies two things: first, the intermolecular interaction is getting stronger than that in the local-order structure prepared at low temperature (100 K). Second, the molecule–substrate interaction is also strong since the orientations of the C60 superstructure are commensurate with those of the substrate. Furthermore, we can clearly see that individual C60 molecules adopt various orientations, rather than the favorite orientations as C60 molecules in the local-order structure. As shown in the high-resolution STM image [Fig. 3(c)], C60 molecules in (√93 × √93) R20° present various shapes, such as two asymmetric lobes (white circle), two symmetrical lobes (yellow circle), and three lobes (blue circle), corresponding to the 5
:
6 C–C bond, 6
:
6 C–C bond, and hexagon facing up. The diversity of C60 molecular orientations is due to the enhancement of intermolecular interaction in the (√93 × √93) R20° superstructure. The intermolecular interaction enables C60 molecules to overcome the molecule–substrate interaction and adopt other orientations, and then make the (√93 × √93) R20° superstructure stable.
When annealed at 400 K for about 20 min, C60 molecules still revealed a hexagonal lattice, while the superstructure transformed from (√93 × √93) R20° into (11 × 11) R0° superstructure [Fig. 4(a)], indicating that (11 × 11) R0° is more stable than (√93 × √93) R20°. The lattice directions of (11 × 11) R0° are along the directions of the Bi(111) substrate, and the lattice constants are c1 = c2 = 5.00 ± 0.02 nm [Fig. 4(b)], corresponding to 11 times of the lattice constant of Bi(111). Fig. 4(d) is the fast Fourier transform (FFT) image of the (11 × 11) R0° superstructure, where the spots marked by red and green circles correspond to C60 hexagonal lattices and the (11 × 11) R0° superstructure. In the FFT image, the spots of the superstructure are clearly visible, though they are dimmer than the spots of C60 hexagonal lattices, implying that the (11 × 11) R0° superstructure has long-range order. The schematic model of (11 × 11) R0° is shown in Fig. 4(c). From STM images, the (11 × 11) R0° superstructure seems to have the same structure as the reported structure attributed to a Moire' pattern in ref. 36. However, in our experiment, the (11 × 11) R0° superstructure is transformed from the (√93 × √93) R20° superstructure and have no relationship with the Moire' pattern. From the close-up view of the (11 × 11) R0° superstructure in Fig. 4(e), it is found that all the C60 molecules reveal a unified three-lobe structure, corresponding to the hexagon facing up, different from favorite orientations in the local-order structure and mixed orientations in (√93 × √93) R20°. With an increase in temperature, the superstructure of the C60 monolayer changes from local order to long-range order and C60 molecules are re-orientated. This is because the thermal diffusivities of C60 molecules and Bi atoms increase with the increase in temperature, which is conducive to the formation of a more orderly and stable superstructure.
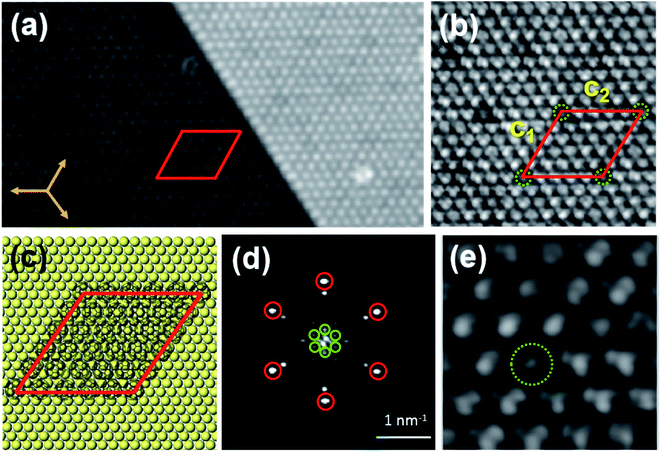 |
| Fig. 4 (a) The STM image of the (11 × 11) R0° superstructure corresponding to the Bi(111), 39 nm × 21 nm, −1.5 V. (b) Close-up view of the (11 × 11) R0° superstructure, 14 nm × 14 nm, −0.7 V. (c) Schematic model of the (11 × 11) R0° superstructure with respect to the Bi(111) lattices. (d) FFT of the image (a). The spots marked by red circles correspond to the C60 hexagonal lattices, while the spots marked by the green circles represent the (11 × 11) R0° superstructure. (e) STM image with a sub-molecular resolution of the superstructure, 5 nm × 5 nm, −0.7 V. | |
Conclusions
In summary, the structure of C60 molecules on Bi(111) changes with temperature variation. When deposited on the Bi(111) surface at 100 K, C60 molecules form local-order structures, and the molecules in local-order structures adopt their favorite orientations. As the deposition temperature increases to room temperature, the local-order structures turn into a long-range ordered (√93 × √93) R20° superstructure. The orientations of C60 molecules in (√93 × √93) R20° superstructures are diverse. After annealing at 400 K for about 20 min, the C60 film exhibits a (11 × 11) R0° superstructure, and all C60 molecules in this superstructure take the unified orientation, hexagon facing-up. The appearance of numerous superstructures and the molecular orientations in superstructures is due to the change in the thermal diffusivity of C60 molecules and Bi atoms at different temperatures.
Conflicts of interest
There are no conflicts to declare.
Acknowledgements
This work was supported by the National Natural Science Foundation of China (Grant Nos. 11804282, 11874304, 11574253).
References
- T. Ohtsuki, K. Masumoto, K. Ohno, Y. Maruyma, Y. Kawazoe, K. Sueki and K. Kikuchi, Insertion of Be Atoms in C60 Fullerene Cages: Be@C60, Phys. Rev. Lett., 1996, 77, 3522 CrossRef CAS PubMed.
- C. Deibel and V. Dyakonov, Polymer–fullerene bulk heterojunction solar cells, Rep. Prog. Phys., 2010, 73, 096401 CrossRef.
- D. J. Hornbaker, S.-J. Kahng, S. Misra, B. W. Smith, A. T. Johnson, E. J. Mele, D. E. Luzzi and A. Yazdani, Mapping the One-Dimensional Electronic States of Nanotube Peapod Structures, Science, 2002, 295, 828–831 CrossRef CAS PubMed.
- H. Park, J. Park, A. K. L. Lim, E. H. Anderson, A. P. Alivisatos and P. L. McEuen, Nanomechanical oscillations in a single-C60 transistor, Nature, 2000, 407, 57–60 CrossRef PubMed.
- P. W. Stephens, G. Bortel, G. Faigel, M. Tegze, A. Jánossy, S. Pekker, G. Oszlanyi and L. Forró, Polymeric fullerene chains in RbC60 and KC60, Nature, 1994, 370, 636–639 CrossRef CAS.
- R. Yamachika, M. Grobis, A. Wachowiak and M. F. Crommie, Controlled atomic doping of a single molecule, Science, 2004, 304, 281–284 CrossRef CAS PubMed.
- H. I. Li, K. Pussi, K. J. Hanna, L. L. Wang, D. D. Johnson, H. P. Cheng, H. Shin, S. Curtarolo, W. Moritz and J. A. Smerdon, Surface Geometry of C60 on Ag(111), Phys. Rev. Lett., 2009, 103, 056101 CrossRef CAS PubMed.
- K. Pussi, H. I. Li, H. Shin, L. N. S. Loli, A. K. Shukla, J. Ledieu, V. Fournee, L. L. Wang, S. Y. Su and K. E. Marino, Elucidating the dynamical equilibrium of C60 molecules on Ag(111), Phys. Rev. B: Condens. Matter Mater. Phys., 2012, 86, 205426 CrossRef.
- H. I. Li, G. J. P. Abreu, A. K. Shukla, V. Fournée, J. Ledieu, L. N. Serkovic Loli, S. E. Rauterkus, M. V. Snyder, S. Y. Su and K. E. Marino, Ordering and dynamical properties of superbright C60 molecules on Ag(111), Phys. Rev. B: Condens. Matter Mater. Phys., 2014, 89, 085428 CrossRef.
- L. Tang, Y. Xie and Q. Guo, Complex orientational ordering of C60 molecules on Au(111), J. Chem. Phys., 2011, 135, 11470211 Search PubMed.
- X. Torrelles, M. Pedio, C. Cepek and R. Felici, (2√3 × 2√3)R30 degrees induced self-assembly ordering by C60 on a Au(111) surface: X-ray diffraction structure analysis, Phys. Rev. B: Condens. Matter Mater. Phys., 2012, 86, 075461 CrossRef.
- M. Passens, R. Waser and S. Karthaeuser, Enhanced fullerene-Au(111) coupling in (2√3 × 2√3)R30 degrees superstructures with intermolecular interactions, Beilstein J. Nanotechnol., 2015, 6, 1421–1431 CrossRef CAS PubMed.
- H. Shin, A. Schwarze, R. D. Diehl, K. Pussi, A. Colombier, E. Gaudry, J. Ledieu, G. M. McGuirk, L. N. S. Loli and V. Fournee, Structure and dynamics of C60 molecules on Au(111), Phys. Rev. B: Condens. Matter Mater. Phys., 2014, 89, 245428 CrossRef.
- G. Schull and R. Berndt, Orientationally ordered (7 × 7) superstructure of C60 on Au(111), Phys. Rev. Lett., 2007, 99, 226105 CrossRef CAS PubMed.
- X. Zhang, F. Yin, R. E. Palmer and Q. Guo, The C60/Au(111) interface at room temperature: A scanning tunnelling microscopy study, Surf. Sci., 2008, 602, 885–892 CrossRef CAS.
- J. A. Gardener, G. A. D. Briggs and M. R. Castell, Scanning tunneling microscopy studies of C60 monolayers on Au(111), Phys. Rev. B: Condens. Matter Mater. Phys., 2009, 80, 235434 CrossRef.
- Y. Z. Shang, Z. L. Wang, D. X. Yang, Y. Y. Wang, C. K. Ma, M. L. Tao, K. Sun, J. Y. Yang and J. Z. Wang, Orientation ordering and chiral superstructures in fullerene monolayer on Cd(0001), Nanomaterials, 2020, 10, 1305 CrossRef CAS PubMed.
- S. Han, M. X. Guan, C. L. Song, Y. L. Wang, M. Q. Ren, S. Meng, X. C. Ma and Q. K. Xue, Visualizing molecular orientational ordering and electronic structure in CsnC60 fulleride films, Phys. Rev. B, 2020, 101, 085413 CrossRef CAS.
- W. W. Pai and C. L. Hsu, Ordering of an incommensurate molecular layer with adsorbate-induced reconstruction:C60/Ag(100), Phys. Rev. B: Condens. Matter Mater. Phys., 2003, 68, 121403 CrossRef.
- C. Grosse, O. Gunnarsson, P. Merino, K. Kuhnke and K. Kern, Nanoscale Imaging of Charge Carrier and Exciton Trapping at Structural Defects in Organic Semiconductors, Nano Lett., 2016, 16, 2084–2089 CrossRef CAS PubMed.
- L. Tang, X. Zhang, Q. Guo, Y. Wu, L. Wang and H. Cheng, Two bonding configurations for individually adsorbed C60 molecules on Au(111), Phys. Rev. B: Condens. Matter Mater. Phys., 2010, 82, 125414 CrossRef.
- L. Tang, X. Zhang and Q. Guo, Organizing C60 molecules on a nanostructured Au(111) surface, Surf. Sci., 2010, 604, 1310–1314 CrossRef CAS.
- T. Hashizume, K. Motai, X. D. Wang, H. Shinohara, Y. Saito, Y. Maruyama, K. Ohno, Y. Kawazoe, Y. Nishina and H. W. Pickering, Intramolecular structures of C60 molecules adsorbed on the Cu(111)-(1 × 1) surface, Phys. Rev. Lett., 1993, 71, 2959–2962 CrossRef CAS PubMed.
- S. Wong, W. W. Pai, C. Chen and M. Lin, Coverage-dependent adsorption superstructure transition of C60/Cu(001), Phys. Rev. B: Condens. Matter Mater. Phys., 2010, 82, 125442 CrossRef.
- G. Xu, X. Shi, R. Q. Zhang, W. W. Pai, H. T. Jeng and M. A. Van Hove, Detailed low-energy electron diffraction analysis of the (4 × 4) surface structure of C60 on Cu(111): Seven-atom-vacancy reconstruction, Phys. Rev. B: Condens. Matter Mater. Phys., 2012, 86, 075419 CrossRef.
- G. Li, H. T. Zhou, L. D. Pan, Y. Zhang, J. H. Mao, Q. Zou, H. M. Guo, Y. L. Wang, S. X. Du and H. J. Gao, Self-assembly of C60 monolayer on epitaxially grown, nanostructured graphene on Ru(0001) surface, Appl. Phys. Lett., 2012, 100, 0133041 Search PubMed.
- M. Jung, D. Shin, S. Sohn, S. Kwon, N. Park and H. Shin, Atomically resolved orientational ordering of C60 molecules on epitaxial graphene on Cu(111), Nanoscale, 2014, 6, 11835–11840 RSC.
- J. G. Hou, J. L. Yang, H. Q. Wang, Q. X. Li, C. G. Zeng, H. Lin, W. Bing, D. M. Chen and Q. S. Zhu, Identifying molecular orientation of individual C60 on a Si(111)-(7 × 7) surface, Phys. Rev. Lett., 1999, 83, 3001–3004 CrossRef CAS.
- H. Q. Wang, C. G. Zeng, B. Wang, J. G. Hou, Q. X. Li and J. L. Yang, Orientational configurations of the C60 molecules in the (2 × 2) superlattice on a solid C60 (111) surface at low temperature, Phys. Rev. B: Condens. Matter Mater. Phys., 2001, 63, 085417 CrossRef.
- A. Goldoni, C. Cepek, M. De Seta, J. Avila, M. C. Asensio and M. Sancrotti, Interaction of C60 with Ge(111) in the 3√3 × 3√3 R30° phase: A (2 × 2) model, Phys. Rev. B: Condens. Matter Mater. Phys., 2000, 61, 10411–10416 CrossRef CAS.
- J. Leaf, A. Stannard, S. P. Jarvis, P. Moriarty and J. L. Dunn, A combined monte carlo and huckel theory simulation of orientational ordering in C60 assemblies, J. Phys. Chem. C, 2016, 120, 8139–8147 CrossRef CAS.
- G. E. Thayer, J. T. Sadowski, F. M. z. Heringdorf, T. Sakurai and R. M. Tromp, Role of surface electronic structure in thin film molecular ordering, Phys. Rev. Lett., 2005, 95, 25610 CrossRef PubMed.
- K. Sun, M. Lan and J. Z. Wang, Absolute configuration and chiral self-assembly of rubrene on Bi(111), Phys. Chem. Chem. Phys., 2015, 17, 26220 RSC.
- M. L. Tao, Y. B. Tu, K. Sun, Y. Zhang, X. Zhang, Z. B. Li, S. J. Hao, H. F. Xiao, J. Ye and J. Z. Wang, Structural transitions in different monolayers of cobalt phthalocyanine film grown on Bi(111), J. Phys. D: Appl. Phys., 2016, 49, 015307 CrossRef.
- J. T. Sadowski, R. Z. Bakhtizin, A. I. Oreshkin, T. Nishihara, A. Al-Mahboob, Y. Fujikawa, K. Nakajima and T. Sakurai, Epitaxial C60 thin films on Bi(0001), Surf. Sci., 2007, 601, 136–139 CrossRef.
- T. Nagao, J. T. Sadowski, M. Saito, S. Yaginuma, Y. Fujikawa, T. Kogure, T. Ohno, Y. Hasegawa, S. Hasegawa and T. Sakurai, Nanofilm allotrope and phase transformation of ultrathin Bi film on Si(111)−7 × 7, Phys. Rev. Lett., 2004, 93, 105501 CrossRef CAS PubMed.
- X. Q. Shi, A. M. Van Hove and R. Q. Zhang, Survey of structural and electronic properties of C60 on close-packed metal surfaces, J. Mater. Sci., 2012, 47, 7341–7355 CrossRef CAS.
- W. W. Pai, H. T. Jeng, C. M. Cheng, C. H. Lin, X. Xiao, A. Zhao, X. Zhang, G. Xu, X. Q. Shi, M. A. Van Hove, C. S. Hsue and K. D. Tsuei, Optimal electron doping of a C60 monolayer on Cu(111) via interface reconstruction, Phys. Rev. Lett., 2010, 104, 036103 CrossRef PubMed.
|
This journal is © The Royal Society of Chemistry 2021 |
Click here to see how this site uses Cookies. View our privacy policy here.