DOI:
10.1039/D1RA00686J
(Paper)
RSC Adv., 2021,
11, 12136-12140
Ligand-free copper-catalyzed C(sp3)–H imidation of aromatic and aliphatic methyl sulfides with N-fluorobenzenesulfonimide†
Received
26th January 2021
, Accepted 20th March 2021
First published on 25th March 2021
Abstract
A novel and efficient process has been developed for copper-catalyzed C(sp3)–H direct imidation of methyl sulfides with N-fluorobenzenesulfonimide(NFSI). Without using any ligands, various methyl sulfides including aromatic and aliphatic methyl sulfides, can be transformed to the corresponding N-((phenylthio)methyl)-benzenesulfonamide derivatives in good to excellent yields.
Nitrogen-containing molecules are widely present in natural products, drug molecules, agrochemicals, and other chemical materials.1 Among the large number of approaches developed for their synthesis,2 the pathway through C–N bond formation has been of great interest to synthetic chemists. This is mainly due to the fact that the introduction of amino groups can facilitate the tuning of physicochemical properties of the molecules leading to biologically significant structures(Fig. 1).3 Typical methods for C–N bond formation include Buchwald–Hartwig, Ullmann–Goldberg and Chan–Evans–Lam imidations, which are mostly transition metal catalyzed, and require prefunctionalization of starting materials.4 Recently, a more concise and atom-economic alternative to such imidations has been investigated by direct C–H imidation of oxidized nitrogen reagents and carbon nucleophiles. This process is also transition metal-catalyzed, however avoids prefunctionalization of coupling partners, thus providing a more efficient and environmentally friendly approach for C–N bond formation.5,6 However, the requirements of directing groups and a stoichiometric amount of oxidants have limited the wide application of these C–H imidation processes.
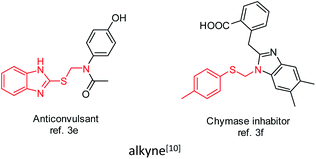 |
| Fig. 1 Representative examples of bioactive amides. | |
N-Fluorobenzenesulfonimide (NFSI), a commonly used fluorination reagent and nitrogen source,7–16 has been recently employed in a variety of aminative functionalization reactions, such as imidations of aromatic C–H bond,8 alkenes or unsaturated ketones,9 alkyne10 and benzylic or allylic C–H bond.11–16 Various transition metals have been explored to catalyze direct imidation of C(sp3)–H bonds with NFSI (Scheme 1). In 2010, a pioneering work on remote amide-directed palladium-catalyzed intermolecular highly selective benzylic C–H imidation with N-fluorobenzenesulfonimide was disclosed by Zhang's group.11 Liu's12 group proposed a copper-catalyzed C(sp3)–H imidation strategy for synthesizing various benzylic amines from benzylic hydrocarbons. The first biocompatible iron-catalyzed benzylic C(sp3)–H imidation of methylarenes with NFSI have been achieved by Bao et al. (Scheme 1a).13 Using palladium as catalyst has also been reported by Muñiz et al.14 to catalyze intermolecular sequence consisting of the C–H activation, where amidation of methyl groups relied on NFSI as both oxidant and nitrogen sources. In addition, copper-catalyzed amidation of 8-methylquinolines with N-fluoroarylsulfonimides via C(sp3)–H activation has been examined by Zheng et al. (Scheme 1b).15 Furthermore, copper-catalyzed sequential C(sp2)/C(sp3)–H imidation of 2-vinylanilines has been performed with NFSI for the efficient synthesis of indole frameworks (Scheme 1c).16
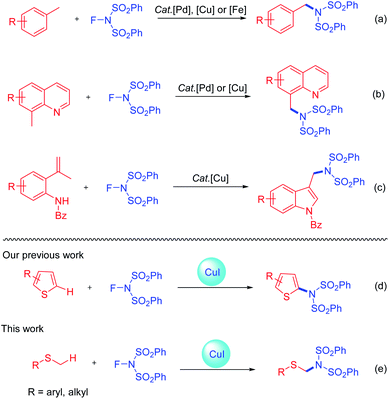 |
| Scheme 1 Methodologies for C(sp3)–H imidation using NFSI. | |
Despite considerable progress in studying transition metal catalyzed C(sp3)–H imidation reactions, limited effort has been made in the methyl C(sp3)–H imidation of thioanisoles with NFSI. Xu and co-workers17 reported the direct imidation of the methyl C(sp3)–H bond of thioanisoles. However, the scope of substrates was limited to thioaromatics with low product yields. Recently, our group has developed a novel method for copper-catalyzed direct imidation of thiophene with NFSI, demonstrating a convenient and complementary approach to synthesizing the gem-thiazamethylene structural motifs exist in biologically active structures (Scheme 1d).8h Built on this development, the present study was extended to the direct C(sp3)–H imidation of methyl sulfides with NFSI catalyzed with ligand-free copper catalysts (Scheme 1e), where both aromatic and aliphatic methyl sulfides were investigated. This strategy provides a facile and efficient process for transition metal-catalyzed C(sp3)–H direct imidation of methyl sulfides.
Initially, the reaction of methyl(phenyl)sulfane 1a with NFSI was carried out successfully by using CuI as a catalyst and NaHCO3 as a base. In the presence of 10 mol% CuI and 2 equiv. of NaHCO3, 1a and with NFSI were under stirring in a reaction medium of 1,2-dichloroethane (DCE) at room temperature for 8 h, and the desired product 3a was obtained in 55% yield (Table 1, entry 1). A brief eximidation of the temperature effect revealed that a temperature of 60 °C gave the highest yield of 3a, i.e. 85% (Table 1, entries 2–4). A higher temperatures of 100 °C showed unfavorable effect on the product yield (Table 1, entry 5). By further examining more copper catalysts including CuCl, CuBr and CuOAc, no higher yields were observed (Table 1, entries 6–8). In the absence of copper catalyst, a 49% product yield was obtained (Table 1, entry 9). Different bases were also employed where NaHCO3 was found to be the most suitable under identical conditions in the reaction process (Table 1, entries 3 and 10–13). In the absence of a base, no detectable product 3a was obtained which indicated a crucial role that the base played in the reaction (Table 1, entry 14). The effect of solvent was then investigated with a range of solvents for this reaction including acetonitrile (CH3CN), dichloromethane (DCM), dimethyl sulfoxide (DMSO) and N,N-dimethylformamide (DMF). It was found that, interestingly, the reaction proceeded in both CH3CN and DCM whereas the use of DMSO and DMF failed to give the desired product (Table 1, entries 15–18). In summary, the optimal reaction conditions were as follows: methyl(phenyl)sulfane 1a (0.5 mmol), NFSI (1.2 equiv.), CuCl (0.1 equiv.) and NaHCO3 (2.0 equiv.) at 60 °C in DCE (3 mL) for 8 h.
Table 1 Optimization of the reaction conditionsa,b

|
Entry |
Catalyst |
Base (2 equiv.) |
Solvent |
Temperature (°C) |
Yield b(%) |
Reactions were carried out on a 0.5 mmol scale in 3.0 mL of solvent with 1 equiv. of 1a, 1.2 equiv. of 2, 2 equiv. of base and 10 mol% of catalyst at 60 °C for 8 h. Isolated yield after column chromatography. n. r. = No reaction. |
1 |
CuI |
NaHCO3 |
DCE |
25 |
55 |
2 |
CuI |
NaHCO3 |
DCE |
40 |
76 |
3 |
CuI |
NaHCO3 |
DCE |
60 |
85 |
4 |
CuI |
NaHCO3 |
DCE |
80 |
81 |
5 |
CuI |
NaHCO3 |
DCE |
100 |
73 |
6 |
CuCl |
NaHCO3 |
DCE |
60 |
76 |
7 |
CuBr |
NaHCO3 |
DCE |
60 |
80 |
8 |
CuOAc |
NaHCO3 |
DCE |
60 |
56 |
9 |
— |
NaHCO3 |
DCE |
60 |
49 |
10 |
CuI |
Na2CO3 |
DCE |
60 |
10 |
11 |
CuI |
NaOtBu |
DCE |
60 |
15 |
12 |
CuI |
K2CO3 |
DCE |
60 |
18 |
13 |
CuI |
Cs2CO3 |
DCE |
60 |
20 |
14 |
CuI |
— |
DCE |
60 |
n. r.c |
15 |
CuI |
NaHCO3 |
CH3CN |
60 |
45 |
16 |
CuI |
NaHCO3 |
DCM |
60 |
55 |
17 |
CuI |
NaHCO3 |
DMSO |
60 |
n. r.c |
18 |
CuI |
NaHCO3 |
DMF |
60 |
n. r.c |
By applying the optimized reaction conditions, the generality and limitations of this ligand-free C–N bond formation were further investigated. The results are presented in Scheme 2. The reaction of NFSI and a variety of methyl(phenyl)sulfane derivatives 1 afforded the desired imidation products 3 in a range of 72–93% either electron electron-donating (1b, 1c) or electron-withdrawing groups (1d–1g) at the para-position of the benzene ring, such as methy, OMe, halogens, or CN, reacted with NFSI effectively to give the desired benzenesulfonamides 3b–3g in moderate to good yields (72–90%). Thioanisole substrates bearing methoxyl, bromo and chloro substituents at meta-position of the benzene ring were found to be good candidates for the imidation, where the corresponding products (3h–3j) were obtained in satisfactory yields (79–93%). Interestingly, (3,5-dichlorophenyl)(methyl)sulfane also afforded target product 3k in a good yield (76%). Substrates bearing methoxyl (1l) or halogen (1m–1p) groups at ortho-position of the benzene ring were also successfully imidated with high yields (3l–3p). Both heterocyclic substrates 2-(methylthio)pyridine (1q) and 2-(methylthio)thiophene (1r) also worked nicely to deliver the imidation products with good to excellent yields (3q–3r, 83–84%). Likely due to the steric effect, ethyl(phenyl)sulfane 1s did not provide imidated product under the optimized reaction conditions. Unfortunately, largely associated with the electronic effect, anisole 1t also failed to afford the desired product.
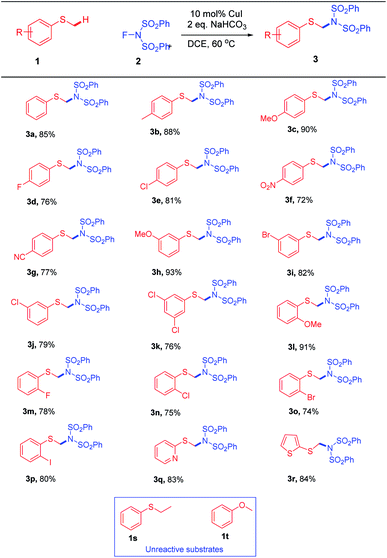 |
| Scheme 2 Substrate Scope of Thioanisoles.a,b aReactions were carried out on a 0.5 mmol scale in 3.0 mL of solvent with 1.0 equiv. of 1, 1.2 equiv. of 2, 2 equiv. of base and 10 mol% of catalyst at 60 °C for 8h. b Isolated yield after column chromatography. | |
To further extend the scope of the imidation reaction, various alky methyl sulfides were also explored as the reactants (Scheme 3). It was demonstrated that both short chain methyl(propyl)sulfane (4a) and long chain dodecyl(methyl)sulfane (4b) reacted well to afford the desired products in satisfactory yields (5a and 5b). Moreover, cyclohexyl(methyl)sulfane (4c) smoothly participated in this reaction to give the desired imidated product in good yields (5c).
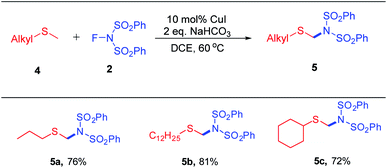 |
| Scheme 3 Substrate scope of alkyl methyl sulfides.a,b aReactions were carried out on a 0.5 mmol scale in 3.0 mL of solvent with 1.0 equiv. of 1, 1.2 equiv. of 2, 2 equiv. of base and 10 mol% of catalyst at 60 °C for 8h. b Isolated yield after column chromatography. | |
In order to demonstrate the synthetic utility of the reaction we developed, this imidation reaction was performed on a 7.0 mmol scale in the presence of 10 mol% of CuI catalyst, giving 2.9 g of the desired product 3i in 83% yield under standard conditions (Scheme 4). Notably, 3i could undergo an oxidation process in the presence of 3.0 equivalents of m-chloroperoxybenzoic acid (m-CPBA) to provide the sulfone 6 in 95% yield.
 |
| Scheme 4 Scaled-up study and application of 3i. | |
Based on the results and discussion above, a possible reaction pathway has been proposed as illustrated in Scheme 5, involving the formation of Cu(I), Cu(II), and Cu(III) complexes,2d,17 although the exact reaction mechanism still remains to be elucidated. Initially, NFSI 2 oxidizes CuI to provide a Cu(III) species A. Next, the substrate 1 can attack A forming the key methylenethionium ions C and the Cu(II) species B. Finally, the methylenethionium ions C is oxidized by the Cu(II) species B to form the imidation product 3 and regenerate CuI for the next catalytic cycle. In this circle system, the addition of chemical equivalent base can clearly enhance increasing the product yield, where the neutralization of HF by NaHCO3 is likely to accelerate the transformation from substrate 1 to intermediate C.
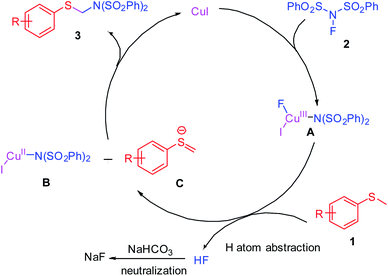 |
| Scheme 5 Plausible reaction pathway. | |
Conclusions
In conclusion, a novel and efficient process has been developed for copper-catalyzed C(sp3)–H direct imidation of methyl sulfides with NFSI. Various methyl sulfides, including aromatic and aliphatic methyl sulfides, can be transformed to the corresponding N-((phenylthio)methyl)-benzenesulfonamide derivatives in good to excellent yields. Currently, the reaction mechanism and further applications of this imidation method are under active investigation in our laboratories.
Conflicts of interest
The authors declare that they have no known competing financial interests or personal relationships that could have appeared to influence the work reported in this paper.
Acknowledgements
This work was supported by Natural Science Foundation of China (No. 2180011182), the Key Research and Development Program of Shaanxi Province (2020ZDLSF03-07), Scientific Research Program Funded by Shaanxi Provincial Education Department (Program No. 20JK0830), Collaborative Innovation Center for Unconventional Oil and Gas Exploration and Development (17JF033).
Notes and references
-
(a) R. Hili and A. K. Yudin, Nat. Chem. Biol., 2006, 2, 284 CrossRef CAS PubMed;
(b) A. L. Harvey, Drug Discovery Today, 2008, 13, 894 CrossRef CAS PubMed;
(c) D. J. Newman and G. M. Cragg, J. Nat. Prod., 2012, 75, 311 CrossRef CAS;
(d) Z.-L. Li and C. Cai, ChemistrySelect, 2017, 2, 8076 CrossRef CAS;
(e) J. P. Clark, K. Feng, A. Sookezain and M. C. White, Nat. Chem., 2018, 10, 583 CrossRef CAS.
-
(a) T. Xiong and Q. Zhang, Chem. Soc. Rev., 2016, 45, 3069 RSC;
(b) C. E. Hendrick and Q. Wang, J. Org. Chem., 2017, 82, 839 CrossRef CAS PubMed;
(c) S. Bhunia, G. G. Pawar, S. V. Kumar, Y. Jiang and D. Ma, Angew. Chem., Int. Ed., 2017, 56, 16136 CrossRef CAS PubMed;
(d) S. Lu, L.-L. Tian, T.-W. Cui, X.-Q. Hao and M.-P. Song, J. Org. Chem., 2018, 83, 13991 CrossRef CAS PubMed;
(e) W. S. Ham, J. Hillenbrand, C. Genicot and T. Ritter, Angew. Chem., Int. Ed., 2019, 58, 532 CrossRef CAS PubMed.
-
(a) J. E. Ney and J. P. Wolfe, J. Am. Chem. Soc., 2006, 128, 15415 CrossRef CAS PubMed;
(b) J. Bariwal and E. V. Eycken, Chem. Soc. Rev., 2013, 42, 9283 RSC;
(c) A. K. Mailyan, J. A. Eickhoff, A. S. Minakova, P. Lu and A. Zakarian, Chem. Rev., 2016, 116, 4441 CrossRef CAS PubMed;
(d) Sushmita, T. Aggarwal, N. Shibata and A. K. Verma, Chem. - Eur. J., 2019, 25, 16063 CrossRef CAS;
(e) R. N. Tiwari, K. G. Bothara, G. S. Chhabra and S. Kulkarni, Res. J. Pharm. Technol., 2010, 2, 466 Search PubMed;
(f) Y. Matsumoto, S. Takeuchi and N. Hase, PCT Int. Appl., 2000, WO2000003997 A1 20000127 Search PubMed.
-
(a) J. F. Hartwig, Acc. Chem. Res., 2008, 41, 1534 CrossRef CAS PubMed;
(b) F. Monnier and M. Taillefer, Angew. Chem., Int. Ed., 2009, 48, 6954 CrossRef CAS;
(c) D. S. Surry and S. L. Buchwald, Chem. Sci., 2011, 2, 27 RSC;
(d) P. Ruiz-Castillo and S. L. Buchwald, Chem. Rev., 2016, 116, 12564 CrossRef CAS PubMed;
(e) S. M. Kelly, C. Han, L. Tung and F. Gosselin, Org. Lett., 2017, 19, 3021 CrossRef CAS PubMed;
(f) W. Xu, Y. Jin, H. Liu, Y. Jiang and H. Fu, Org. Lett., 2011, 13, 1274 CrossRef CAS PubMed;
(g) Z.-Y. Ge, X.-D. Fei, T. Tang, Y.-M. Zhu and J.-K. Shen, J. Org. Chem., 2012, 77, 5736 CrossRef CAS PubMed;
(h) C. Sambiagio, S. Marsden, P. A. J. Blacker and P. C. McGowan, Chem. Soc. Rev., 2014, 43, 3525 RSC;
(i) J. C. Vantourout, H. N. Miras, A. Isidro-Llobet, S. Sproules and A. J. B. Watson, J. Am. Chem. Soc., 2017, 139, 4769 CrossRef CAS PubMed.
-
(a) X. Yan, X. Yang and C. Xi, Catal. Sci. Technol., 2014, 4, 4169 RSC;
(b) C. E. Hendrick, K. J. Bitting, S. Cho and Q. Wang, J. Am. Chem. Soc., 2017, 139, 11622 CrossRef CAS PubMed;
(c) Y.-H. Chen, S. Graβl and P. Knochel, Angew. Chem., Int. Ed., 2018, 57, 1108 CrossRef CAS PubMed;
(d) Y. Segawa, T. Maekawa and K. Itami, Angew. Chem., Int. Ed., 2015, 54, 66 CrossRef CAS PubMed;
(e) K. Murakami, S. Yamada, T. Kaneda and K. Itami, Chem. Rev., 2017, 117, 9302 CrossRef CAS PubMed;
(f) P. Becker, T. Duhamel, C. J. Stein, M. Reiher and K. Muniz, Angew. Chem., Int. Ed., 2017, 56, 8004 CrossRef CAS PubMed;
(g) A. E. Bosnidou and K. Muniz, Angew. Chem., Int. Ed., 2019, 58, 7485 CrossRef CAS PubMed.
-
(a) M.-L. Louillat and F. W. Patureau, Chem. Soc. Rev., 2014, 43, 901 RSC;
(b) K. Shin, H. Kim and S. Chang, Acc. Chem. Res., 2015, 48, 1040 CrossRef CAS PubMed;
(c) J. Jiao, K. Murakami and K. Itami, ACS Catal., 2016, 6, 610 CrossRef CAS;
(d) H. Kim and S. Chang, ACS Catal., 2016, 6, 2341 CrossRef CAS;
(e) Y. Park, Y. Kim and S. Chang, Chem. Rev., 2017, 117, 9247 CrossRef CAS.
- For selected examples, see:
(a) X.-Y. Yang, T. Wu, R. J. Phipps and F. D. Toste, Chem. Rev., 2015, 115, 826 CrossRef CAS PubMed;
(b) T. Liang, C. N. Neumman and T. Ritter, Angew. Chem., Int. Ed., 2013, 52, 8214 CrossRef CAS PubMed;
(c) X. Wang, Z.-J. Wu and J. Wang, Org. Lett., 2016, 18, 576 CrossRef CAS PubMed;
(d) H.-H. Peng and G.-S. Liu, Org. Lett., 2011, 13, 772 CrossRef CAS PubMed;
(e) L. Yang, Y.-H. Ma, F.-J. Song and J.-S. You, Chem. Commun., 2014, 50, 3024 RSC;
(f) F.-H. Li, Z.-J. Cai, L. Yin, S.-Y. Wang and S.-J. Ji, Org. Lett., 2017, 19, 1662 CrossRef CAS PubMed;
(g) Y.-L. Zhu, A.-F. Wang, J.-Y. Du, B.-R. Leng, S.-J. Tu, W.-J. Hao and B. Jiang, Chem. Commun., 2017, 53, 6397 RSC;
(h) D. S. Timofeeva, A. R. Ofial and H. Mayr, J. Am. Chem. Soc., 2018, 140, 11474 CrossRef CAS PubMed;
(i) Y. Li and Q. Zhang, Synthesis, 2015, 47, 159 CAS.
- For selected examples, see:
(a) K. Sun, Y. Li, T. Xiong, J.-P. Zhang and Q. Zhang, J. Am. Chem. Soc., 2011, 133, 1694 CrossRef CAS;
(b) G. B. Boursalian, M.-Y. Ngai, K. N. Hojczyk and T. Ritter, J. Am. Chem. Soc., 2013, 135, 13278 CrossRef CAS;
(c) R.-J. Tang, C.-P. Luo, L. Yang and C.-J. Li, Adv. Synth. Catal., 2013, 355, 869 CrossRef CAS;
(d) Y. Wang, Z.-Y. Guo, Q. Zhang and D. Li, Asian J. Org. Chem., 2016, 5, 1438 CrossRef CAS;
(e) T. Kawakami, K. Murakami and K. Itami, J. Am. Chem. Soc., 2015, 137, 2460 CrossRef CAS PubMed;
(f) E. Ito, T. Fukushima, T. Kawakami, K. Murakami and K. Itami, Chem, 2017, 2, 383 CrossRef CAS;
(g) H.-H. Liu, Y. Wang, G.-J. Deng and L. Yang, Adv. Synth. Catal., 2013, 355, 3396 CrossRef;
(h) S. Wang, Z. Ni, J. Wang and Y. Pan, Org. Lett., 2014, 16, 5648 CrossRef CAS PubMed;
(i) M. Singsardar, S. Mondal, R. Sarkar and A. Hajra, ACS Omega, 2018, 3, 12505 CrossRef CAS PubMed.
- For selected examples, see:
(a) P. A. Sibbald, C. F. Rosewall and F. E. Michael, J. Am. Chem. Soc., 2009, 131, 15945 CrossRef CAS PubMed;
(b) P. A. Sibbald and F. E. Michael, Org. Lett., 2009, 11, 1147 CrossRef CAS PubMed;
(c) T. Xiong, Y. Li, L.-J. Mao and Q. Zhang, Chem. Commun., 2012, 48, 2246 RSC;
(d) H.-W. Zhang, W.-Y. Pu, T. Xiong, K. Sun, Q. Liu and Q. Zhang, Angew. Chem., Int. Ed., 2013, 52, 2529 CrossRef CAS PubMed;
(e) H.-W. Zhang, Y.-C. Song, J.-B. Zhao, J.-P. Zhang and Q. Zhang, Angew. Chem., Int. Ed., 2014, 53, 11079 CrossRef CAS PubMed;
(f) G. Zhang, T. Xiong, Z.-N. Wang, G.-X. Xu and Q. Zhang, Angew. Chem., Int. Ed., 2015, 54, 12649 CrossRef CAS PubMed;
(g) D.-H. Wang, L.-Q. Wu, Z.-Y. Lin and G.-S. Liu, J. Am. Chem. Soc., 2017, 139, 6811 CrossRef CAS PubMed;
(h) T. W. Pouambeka, G. Zhang, T. Xiong and Q. Zhang, Org. Chem. Front., 2017, 4, 1420 RSC;
(i) D.-K. Li, T.-T. Mao, J.-B. Huang and Q. Zhu, Chem. Commun., 2017, 53, 3450 RSC;
(j) J. Xie, Y.-W. Wang, L.-W. Qi and B. Zhang, Org. Lett., 2017, 19, 1148 CrossRef CAS PubMed;
(k) H.-W. Xiao, H.-G. Shen, L. Zhu and C.-Z. Li, J. Am. Chem. Soc., 2019, 141, 11440 CrossRef CAS PubMed;
(l) M. Iwasaki, K. Nonaka, S. Zou, K. Nakajima and Y. Nishihara, J. Org. Chem., 2019, 84, 15373 CrossRef CAS PubMed.
-
(a) G.-F. Zheng, Y. Li, J.-J. Han, T. Xiong and Q. Zhang, Nat. Commun., 2015, 6, 7011 CrossRef CAS PubMed;
(b) G.-F. Zheng, J.-B. Zhao, Z.-Y. Li, H.-Z. Sun and Q. Zhang, Chem. - Eur. J., 2016, 22, 3513 CrossRef CAS PubMed;
(c) J.-Q. Sun, G.-F. Zheng, T. Xiong and Q. Zhang, ACS Catal., 2016, 6, 3674 CrossRef CAS;
(d) J.-Q. Sun, G.-F. Zheng, Y. Li and Q. Zhang, Org. Lett., 2017, 19, 3767 CrossRef CAS PubMed;
(e) C. R. Reddy, S. K. Prajapti and R. Ranjan, Org. Lett., 2018, 20, 3128 CrossRef CAS PubMed;
(f) S. Samanta and A. Hajra, J. Org. Chem., 2018, 83, 13157 CrossRef CAS PubMed;
(g) X.-T. Zhu, W.-L. Deng, L. Ge, X.-H. Zhang and H.-L. Bao, J. Am. Chem. Soc., 2019, 141, 548 CrossRef CAS PubMed.
- T. Xiong, Y. Li, Y. Lv and Q. Zhang, Chem. Commun., 2010, 46, 6831 RSC.
- Z.-K. Ni, Q. Zhang, T. Xiong, J.-P. Zhang and Q. Liu, Angew. Chem., Int. Ed., 2012, 51, 1244 CrossRef CAS PubMed.
- F.-Y. Bao, Y.-H. Cao, W.-B. Liu and J.-H. Zhu, RSC Adv., 2019, 9, 27892 RSC.
- Á. Iglesias, R. Álvarez, Á. R. de Lera and K. Muñiz, Angew. Chem., Int. Ed., 2012, 51, 2225 CrossRef PubMed.
- X.-L. Zhang, R. Wu, P.-J. Jiang and Q.-Z. Zheng, Org. Biomol. Chem., 2016, 14, 4789 RSC.
- W.-B. Cao, X.-P. Xu and S.-J. Ji, Adv. Synth. Catal., 2019, 361, 1771 CrossRef CAS.
- Z.-H. Yang, S.-Y. Yang and J.-X. Xu, Tetrahedron, 2017, 73, 3240 CrossRef CAS.
Footnote |
† Electronic supplementary information (ESI) available. See DOI: 10.1039/d1ra00686j |
|
This journal is © The Royal Society of Chemistry 2021 |