DOI:
10.1039/D1RA00610J
(Paper)
RSC Adv., 2021,
11, 9856-9864
The effect of different thawing methods on the health-promoting compounds and antioxidant capacity in frozen baby mustard†
Received
23rd January 2021
, Accepted 26th February 2021
First published on 9th March 2021
Abstract
The effects of five domestic thawing methods, including air thawing (AIR), water thawing with bags (W + B), water thawing without bags (W − B), refrigerator thawing (REF), and microwave thawing (MIC), on the main health-promoting compounds and antioxidant capacity in both unblanched and blanched baby mustard were investigated in this study. The results showed that different thawing methods markedly affected the health-promoting compounds and antioxidant capacity of baby mustard. MIC better retained the overall nutritional quality of frozen baby mustard compared with the four other treatments. AIR led to significant decreases in the glucosinolate contents in unblanched and blanched baby mustard. W + B led to significant decreases in the total phenols contents and antioxidant capacity levels in unblanched and blanched baby mustard, as well as the ascorbic acid content in unblanched baby mustard. W + B led to a significant decrease in the FRAP level in unblanched baby mustard, as well as the glucosinolate and ascorbic acid contents and ABTS level in blanched baby mustard. REF led to significant decreases in glucosinolates and proanthocyanidins contents in unblanched baby mustard, as well as the ascorbic acid content in blanched baby mustard. Furthermore, the thawing time was greatly shortened by MIC (only approximately 1 min). Thus, MIC was the optimal thawing method for frozen baby mustard regardless of whether it was blanched, as MIC best preserved nutritional quality and reduced the thawing time.
Introduction
Baby mustard (Brassica juncea var. gemmifera) is a variant of stem mustard. The heads of baby mustard are commonly consumed, and the lateral buds of the head are tender with a sweet and fragrant flavor and can be eaten cooked or pickled.1,2 Baby mustard contains numerous health-promoting compounds, including glucosinolates, carotenoids, ascorbic acid, and polyphenols.1–3 Epidemiological studies have shown that glucosinolates are associated with a reduced risk of various cancers (bladder, colon and lung cancers, etc.) and cardiovascular diseases.1,4 Additionally, glucosinolates also affect flavor (such as pungent and hot flavors) of cruciferous plants.1,5 Carotenoids, ascorbic acid, and polyphenols are also considered important antioxidants because of their activities against free radicals, carcinogenesis, and cardiovascular diseases, as well as their ability to stimulate the immune system.6,7 In addition, baby mustard is easy to survive and be managed, and does not require high labor. In Southwest China, the cultivated area of baby mustard is more than 60
000 ha every year. The yield of baby mustard is also as large as 35–60 tons per ha under normal years, which is higher than that of many local traditional vegetables. Thus, the economic benefits of baby mustard are relatively good for growers and sellers.
However, baby mustard is a seasonal and highly perishable vegetable with a short shelf life.2,3 After it is harvested, baby mustard begins to undergo higher rates of respiration, resulting in moisture loss, browning of the epidermis, and quality deterioration.3 These characteristics of baby mustard make its distribution and marketing particularly challenging. Thus, the application of effective and proper post-harvest techniques is critically important for promoting the development of the baby mustard industry. Frozen storage is one of the most popular and effective processing approaches for maintaining the post-harvest quality and nutritional properties of various vegetables.8–10 In Southwestern China, baby mustard is often used as a frozen vegetable, and blanching is an optional treatment before freezing. However, frozen vegetables have to be thawed before consumption. Thawing is the process of changing a product from a frozen to unfrozen state. The point at which ice crystals are converted back to water occurs completely when the temperature throughout the product reaches 0 °C and no free ice is present.11 Frozen vegetables are vulnerable to damage induced by chemical changes during the thawing process.12–15 For example, thawing affected the stability of bioactive compounds, including glucosinolates, total phenolics, and carotenoids, of frozen broccoli,13 also resulted in loss of vitamin C in frozen red radishes.16 The goal of thawing, one of the most important stages for determining the final quality of frozen vegetables, is to obtain high-quality thawed vegetables with the shortest possible thawing time.16 Few studies have examined the effect of thawing methods on the main health-promoting compounds and antioxidant capacity in both blanched and unblanched baby mustard.
Here, we assessed the effect of five domestic thawing methods on various quality characteristics, such as glucosinolates, carotenoids, ascorbic acid, proanthocyanidins, total phenolics, and antioxidant capacity, in both unblanched and blanched baby mustard. The overall goal was to assess the efficacy and efficiency of the different thawing methods for maintaining the quality of both blanched and unblanched baby mustard.
Experimental
Plant materials
Baby mustard (Brassica juncea var. gemmifera cv. Linjiang-Ercai) was obtained from a local farm in Chengdu City, China. Heads with uniform size and absence of external damage were selected and harvested in the morning, and then transported to the laboratory immediately. Healthy lateral buds, the main edible parts of baby mustard, were cut off, washed in tap water, dried on blotting paper, and then cut into thin slices of approximately 3 mm. The lateral buds were assigned randomly to “unblanched” and “blanched” treatment groups. The blanched treatment group of bud slices was randomly divided into four samples that were separately blanched at 96 °C for 30 s and then immediately cooled in cold water (3 °C for 90 s), drained, and dry using paper towels. Similarly, the unblanched treatment group of bud slices were also randomly divided into four samples. Each sample of the unblanched and blanched treatment groups was divided into 6 portions (100 ± 5 g each) that were packed in transparent polypropylene bags, and then stored at −20 °C in a domestic deep chest freezer until thawing process.
Thawing of frozen baby mustard
The frozen baby mustard samples were thawed by five methods: (i) thawing with bags at room temperature (20 ± 2 °C) in air (AIR); (ii) thawing with bags in 10 volumes of 18 °C water (W + B); (iii) thawing without bags in 10 volumes of 18 °C water (W − B); (iv) thawing with bags in a 5 °C refrigerator (REF); and (v) thawing without bags in a microwave oven at 700 W (MIC). The thawing time reached the endpoint when the temperature in the center of the bud slices of baby mustard reached 4 °C (ESI Fig. 1†). The control samples consisted of frozen (not thawed) samples. The thawed or frozen samples were then lyophilized in a freeze-dryer and stored at −20 °C for further analysis.
Thawing time monitoring
Thawing time by different methods was recorded from the beginning of thawing to the temperature in the center of the bud slices of baby mustard reached 4 °C. The temperature was monitored by a probe type food thermometer (Chigo Technology Co., Ltd., Foshan City, China).
Quality assessment
Glucosinolate composition and contents. Glucosinolates were extracted and analyzed as previously described.1 Freeze-dried samples (100 mg) were boiled in 5 mL water for 10 min. The supernatant was collected after centrifugation, and the residues were washed once with water, centrifuged and then combined with the previous extract. The aqueous extract was applied to a DEAE-Sephadex A-25 column. The glucosinolates were converted into their desulpho analogues by overnight treatment with 100 μL of 0.1% aryl sulphatase, and the desulphoglucosinolates were eluted with 1 mL water. High performance liquid chromatography (HPLC) analysis of desulphoglucosinolates was carried out using an Agilent 1260 HPLC instrument equipped with a variable wavelength detector (VWD). Samples were separated at 30 °C on a Waters Spherisorb C18 column (250 × 4.6 mm) using acetonitrile and water at a flow rate of 1.0 mL min−1. Absorbance was detected at 226 nm.
Chlorophyll and carotenoid contents. The contents of chlorophyll and carotenoid were determined using the method of Sun et al.3 Two hundred mg powder of lateral bud were ground and extracted with 25 mL acetone. The samples were sonicated for 20 min, and centrifuged at 4000g at room temperature (20 ± 2 °C) for 5 min. The supernatant was filtered through 0.22 μm nylon syringe filters and analyzed by HPLC. HPLC analysis of chlorophylls and carotenoids were carried out using an Agilent 1260 instrument with a VWD detector. Samples (10 μL) were separated at 30 °C on a Waters C18 column (150 × 3.9 mm) using isopropanol and 80% acetonitrile–water at a flow rate of 0.5 mL min−1. Absorbances were detected at 448 and 428 nm.
Ascorbic acid content. Ascorbic acid content was determined according to the previous report.1 Fifty mg of sample powder was extracted with 5 mL 1.0% oxalic acid, subsequently centrifuged 5 min at 4000g. Each sample was filtered through a 0.45 μm cellulose acetate filter. HPLC analysis of ascorbic acid was carried out using an Agilent 1260 instrument with a VWD detector. Samples were separated on a Waters Spherisorb C18 column (150 × 4.6 mm), using a solvent of 0.1% oxalic acid at a flow rate of 1.0 mL min−1. The amount of ascorbic acid was calculated from absorbance values at 243 nm, using authentic ascorbic acid (Solarbio Science & Technology Co., Ltd., Beijing, China) as a standard.
Proanthocyanidins content. Proanthocyanidins content was determined according to the method described by Prior et al.17 Forty milligrams of sample powder was transferred to 4 mL of the extracting reagent (acetone
:
distilled water
:
acetic acid = 150
:
49
:
1, v/v). The solution was vigorously vortexed for 5 min, shaken for 1 h, and then centrifuged at 4000g for 5 min. Subsequently, 2.1 mL p-dimethylaminocinnamaldehyde reagent was added to 700 μL of supernatant. The absorbance of the mixture was spectrophotometrically detected at 640 nm after 20 min, and the proanthocyanidins content was determined using a standard curve of procyanidin B2 (Sigma Chemical Co., Saint Louis, USA).
Total phenolics content. Total phenolics were extracted with 10 mL of 50% ethanol (anhydrous) and incubated at room temperature (20 ± 2 °C) for 24 h in the dark. The suspension was centrifuged at 4000g for 5 min at room temperature. The supernatant was used for the measurements of total phenolics content and antioxidant activity. The supernatant was mixed with Folin–Ciocalteu reagent, after 3 min, saturated sodium carbonate was added. The absorbance was measured at 760 nm with the UV-1800 spectrophotometer (Mapada Instruments Co., Ltd., Shanghai, China) as previously described.1 Gallic acid was used as a standard and the results were expressed as mg gallic acid equivalent per g dry weight.
Ferric reducing antioxidant power (FRAP). FRAP assay was performed according to the previous report.18 The extracted samples were added to the FRAP working solution incubated at 37 °C and vortexed. The absorbance was then recorded at 593 nm using a UV-1800 spectrophotometer (Mapada Instruments Co., Ltd., Shanghai, China) after the mixture had been incubated in at 37 °C for 10 min. FRAP values were calculated based on FeSO4·7H2O standard curves and expressed as μmol g−1 dry weight.
2,2-Azinobis(3-ethyl-benzothiazoline-6-sulfonic acid) (ABTS) assay. ABTS antioxidant activity was performed according to the previous report.1 An aliquot of 300 μL of each extracted sample was added to 3 mL of ABTS+ solution. The absorbance was measured spectrophotometrically at 734 nm after accurately 2 h. The percentage inhibition was calculated according to the formula: % inhibition = [(Acontrol − Asample)/Acontrol] × 100%.
Statistical analysis
All assays were performed in quadruplicate. The design was completely random. Statistical analysis was performed using the SPSS package program version 18 (SPSS Inc., Chicago, IL, USA). Data were analyzed using a one-way analysis of variance model. The means were compared through the least significant difference (LSD) test at a significance level of 0.05. Principal component analysis (PCA) was performed in SIMCA-P 11.5 software (Umetrics, Sweden) with unit variance (UV)-scaling to decipher the relationships among samples.1
Results and discussion
Thawing time
The thawing time of frozen baby mustard varied greatly among the various thawing methods (Table 1). But, remained equal for bleached and unbleached samples. The thawing time mainly depends on the temperature difference between the thawing medium and frozen samples, and the heat transfer of thawing methods is from the surface to the inside.14 In addition, the thermal conductivity of water thawing is greater than that of air thawing.19 As expected, REF had the longest thawing time, followed by AIR, W + B, and W − B. MIC was the fastest thawing method, and unblanched and blanched frozen baby mustard both reached 4 °C in approximately 1 min. The thawing time of MIC decreased approximately 360 and 60 times compared with REF and AIR, respectively, which might be attributed to the fact that microwave energy can penetrate food and produce heat internally.14,20
Table 1 Thawing time of baby mustard with different thawing methods
Thawing methods |
Thawing time/min |
Unblanched |
Blanched |
AIR |
60 ± 2b |
60 ± 3b |
WB |
5.5 ± 0.4c |
5.5 ± 0.2c |
WNB |
4.0 ± 0.4c |
3.5 ± 0.1c |
REF |
361 ± 1a |
362 ± 6a |
MIC |
1.0 ± 0.1c |
1.0 ± 0.1c |
Glucosinolates
Total and individual glucosinolates were determined in frozen and defrosted baby mustard (Fig. 1). Seven glucosinolates were detected in the lateral buds of baby mustard, including three aliphatic glucosinolates (sinigrin, gluconapin, and progoitrin) and four indole glucosinolates (4-methoxyglucobrassicin, glucobrassicin, neoglucobrassicin, and 4-hydroxy glucobrassicin). The most abundant glucosinolate in the lateral buds of baby mustard was sinigrin, which accounted for more than 94% and 90% of total aliphatic and total glucosinolate contents, respectively, followed by gluconapin. The predominant indole glucosinolates were 4-methoxyglucobrassicin and glucobrassicin. The glucosinolate profile observed was similar to that obtained in our previous studies.2,3 Nevertheless, glucoalyssin and glucoiberin, the contents of which were found to be low in previous studies,2,3 were not detected in frozen and defrosted baby mustard. The various glucosinolate profiles may stem from the different post-harvest treatments applied in each study. Baby mustard used in this study was previously subjected to the cutting and freezing process, which may degrade glucosinolates of baby mustard in a certain extent.13
 |
| Fig. 1 Effect of different thawing methods on the contents of individual and total glucosinolates in frozen baby mustard. Each value is the mean ± standard error of four replicates (n = 4). The values were compared through the least significant difference (LSD) test within the unblanched and blanched groups, respectively. Values in each group not sharing a common letter are significantly different at p < 0.05. DW: dry weight. | |
The aliphatic and total glucosinolates in unblanched baby mustard were significantly decreased to varying degrees after thawing by different methods (with the exception of the gluconapin content under W − B), and the declines were the greatest under REF. The contents of indole glucosinolates under MIC, W − B, and W + B were relatively high, whereas those under REF and AIR were relatively low. Therefore, REF was unfavorable for the maintenance of glucosinolate contents for unblanched baby mustard. This may stem from the long thawing time of REF and the resurrection of part of the myrosinase in the cells of unblanched baby mustard,21 leading to the degradation of glucosinolates.
The aliphatic and total glucosinolate contents in blanched baby mustard under W + B, MIC, REF, and AIR were not significantly different from those in frozen baby mustard (with the exception of the progoitrin content of baby mustard under AIR), whereas those under W − B were significantly reduced. The individual and total indole glucosinolates (with the exception of the 4-hydroxy-glucobrassicin content under MIC) were high under REF, MIC, and W + B, and those under AIR and W − B were relatively low. Therefore, W − B is not conducive to the maintenance of glucosinolate contents for blanched baby mustard. Glucosinolates are water-soluble compounds.22 Baby mustard was in direct contact with water during the thawing process only under W − B, which caused more glucosinolates to be immersed in the water, resulting in greater losses of glucosinolates compared with the other thawing methods. Additionally, blanched treatment resulted in lower losses of glucosinolate contents compared with the unblanched treatment during thawing process, except for WNB thawing.
Chlorophylls and carotenoids
Vegetable color is an important factor used by consumers for judging quality.23 The color of the lateral buds of baby mustard is mainly determined by chlorophylls and carotenoids.1,3 In this study, there were two chlorophylls (chlorophyll a and chlorophyll b) and four carotenoids (neoxanthin, violaxanthin, lutein, and β-carotene) detected in the baby mustard buds (Fig. 2 and ESI Fig. 2†), which was also consistent with previous studies.1,3
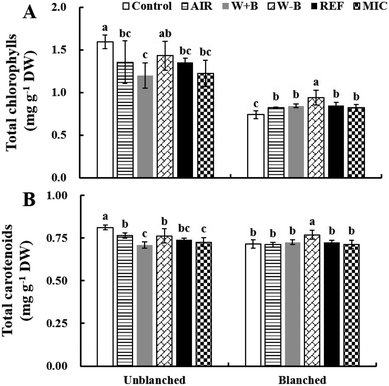 |
| Fig. 2 Effect of different thawing methods on the contents of total chlorophylls (A) and total carotenoids (B) in frozen baby mustard. Each value is the mean ± standard error of four replicates (n = 4). The values were compared through the least significant difference (LSD) test within the unblanched and blanched groups, respectively. Values in each group not sharing a common letter are significantly different at p < 0.05. DW: dry weight. | |
For unblanched baby mustard, the total chlorophyll contents were 10.2%, 14.9%, 15.0%, 23.2%, and 24.7% lower in W − B, REF, AIR, MIC, and W + B samples, respectively, compared with the control; the total carotenoid contents were 5.8%, 6.1%, 8.8%, 10.7%, and 12.5% lower in AIR, W − B, REF, MIC, and W + B samples, respectively, compared with the control. These changes might occur because of the degradation associated with pigment-degrading enzymes. Enzyme activity is slowed at temperatures below −18 °C, and enzymes become activated again during the thawing process.21 This indicates that W + B was not conducive to the maintenance of chlorophyll and carotenoid contents in unblanched baby mustard.
The chlorophyll content of blanched baby mustard increased significantly after various thawing treatments, and the content increased the most after W − B. By contrast, the carotenoid content remained unchanged after various thawing treatments; the exception was W − B, in which the carotenoid contents increased significantly after thawing. These results were different from those of unblanched baby mustard: the chlorophyll and carotenoid contents of blanched baby mustard after thawing did not significantly differ even increase compared with the control. There are two possible reasons for this result. First, blanching before freezing might inactivate the pigment-related enzymes, thus preventing the degradation of chlorophylls and carotenoids during thawing.24,25 Second, the extractability of chlorophyll and carotenoids was likely improved by blanching treatment because of the disruption of the pigment–protein complexes and/or the loss of soluble solids.13,26 A previous study also reported that defrosting broccoli in boiling water increased the carotenoid content.13 In addition, most of chlorophyll and carotenoid contents without blanching treatment were significantly reduced after thawing, whereas the samples with blanching did not differ significantly from the control after thawing or increased significantly, this behaviour could be related to the balance between increase in thermal-induced extractability and degradation of the compounds.13
Ascorbic acid
Fruits and vegetables are key sources of dietary vitamin C and provide more than 90% of the vitamin C required for humans; the main biologically active form of vitamin C is L-ascorbic acid.27 The ascorbic acid content of frozen, unblanched baby mustard decreased significantly after various thawing treatments (Fig. 3A). A similar decrease has also been observed for Hami melon14 and strawberries.28,29 This probably stems from the enzymatic and non-enzymatic oxidation of ascorbic acid in the presence of oxygen.29 The highest retention of ascorbic acid was detected in baby mustard under AIR (87.0%), followed by MIC, W − B, and REF (86.1%, 80.3%, and 80.1%, respectively), and the lowest was observed for W + B (69.6%). However, no substantial difference was observed in the retention of ascorbic acid among various thawing methods (except for W + B). Therefore, W + B was not conducive to the maintenance of ascorbic acid in unblanched baby mustard.
 |
| Fig. 3 Effect of different thawing methods on the contents of ascorbic acid (A), proanthocyanidins (B), and total phenolics (C) in frozen baby mustard. Each value is the mean ± standard error of four replicates (n = 4). The values were compared through the least significant difference (LSD) test within the unblanched and blanched groups, respectively. Values in each group not sharing a common letter are significantly different at p < 0.05. DW: dry weight. | |
The ascorbic acid contents of W + B, MIC, and AIR for blanched baby mustard did not significantly differ from the ascorbic acid content of the control. By contrast, the ascorbic acid contents of W − B and REF were significantly reduced by 15.0% and 13.2%, respectively, compared with the control (Fig. 3A). The proportion of ascorbic acid lost under W − B was the highest among the various thawing methods, which presumably stems from the fact that the ascorbic acid of baby mustard under W − B was immersed in water.10 The loss of ascorbic acid under REF was also relatively large, which might be attributed to the prolonged thawing time. This is consistent with a previous study on strawberries showing that thawing at 4 °C caused the most pronounced losses (34.8%), indicating that thawing time is a key factor for improving ascorbic acid retention.29 Therefore, W − B and REF were not conducive to the maintenance of ascorbic acid content in blanched baby mustard. Like glucosinolates and pigments, blanched treatment resulted in a lower loss of ascorbic acid content compared with the unblanched treatment during thawing process.
Proanthocyanidins
Proanthocyanidins are one of the most important antioxidant substances.30 The proanthocyanidins contents of unblanched baby mustard decreased significantly after various thawing treatments, and the proanthocyanidins content decreased the most after REF. The proanthocyanidins content of blanched baby mustard after various thawing treatments did not significantly differ from that of the control; the exception was for MIC, wherein the proanthocyanidins content significantly decreased (Fig. 3B). Therefore, REF and MIC were not conducive to the maintenance of proanthocyanidins content in unblanched and blanched baby mustard, respectively.
Total phenolics
The content of polyphenols in vegetables can be influenced by various factors, such as species, varieties, climatic conditions, cultural practices, and maturity at harvest, storage, and processing conditions.31 Some researchers have reported an increase in the phenolic content with processing, whereas others have observed a decrease.10,13,29 In this study, the total phenolics contents of baby mustard thawed using various methods did not significantly differ from the control with the exception of W + B (Fig. 3C). Compared with the control, the total phenolics contents of unblanched and blanched baby mustard were 20.8% and 26.8% lower under W + B, respectively, suggesting that W + B was not conducive to the maintenance of total phenolics content in baby mustard regardless of whether it was blanched.
Antioxidant capacity
Vegetables belonging to the Brassica family have a rich antioxidant composition (ascorbic acid, carotenoids, and phenolics) and a high potential to protect against oxidative stress.6,27 In this study, the antioxidant capacities of baby mustard were investigated using both FRAP and ABTS assays (Fig. 4). FRAP is evaluated from a redox reaction occurring between the substrate (electron donor) and Fe3+ ions (electron acceptor), which leads to the production of Fe2+ ions.32 All thawing treatments led to a significant loss in FRAP antioxidant capacity in unblanched baby mustard; the largest decrease in the FRAP level was observed under W + B (37.8%). However, only W + B and W − B led to a significant loss in FRAP antioxidant capacity for blanched baby mustard. The greatest decrease in the FRAP level in blanched baby mustard was also observed under W + B (36.6%). Therefore, W + B was not conducive to the maintenance of the FRAP level in both unblanched and blanched baby mustard. The ABTS assay determines the scavenging ability of the antioxidant activity through reaction with a strong antioxidant agent (potassium permanganate or potassium persulfate) in the presence of ABTS salt.33 With exception of W + B and AIR, The ABTS levels of unblanched baby mustard after WNB, REF, and MIC were not significantly different from those of the control, and the lowest levels were observed in mustard under W + B. The ABTS levels of blanched baby mustard under W + B and W − B were significantly reduced compared with the control; by contrast, there were no significant differences in the ABTS levels between the control and the other three thawing methods. The lowest ABTS antioxidant capacity level was observed in blanched baby mustard under W + B. Similar to the FRAP results, W + B was not conducive to the maintenance of the ABTS level in both unblanched and blanched baby mustard. However, there were differences in the antioxidant capacity determined by the FRAP and ABTS assays, which may be related to differences in the assays, indicating that the antioxidant substances determined by the two assays differed. Therefore, multiple methods should be used to measure the antioxidant capacity to accurately reflect actual situations. The antioxidant activity is often found to be correlated with the total phenolics content in many studies.6,34 In our study, the antioxidant capacity of baby mustard was not consistent with the changes in total phenols. This may be because antioxidant capacity is a product of multiple antioxidants,25 and the content of total phenolics in baby mustard is generally low.3 Other antioxidants, such as ascorbic acid, may determine the antioxidant capacity in baby mustard rather than total phenolics.3 Overall, W + B was not conducive to the maintenance of the antioxidant capacity of baby mustard regardless of whether it was blanched.
 |
| Fig. 4 Effect of different thawing methods on the levels of FRAP (A) and ABTS+ (B) in frozen baby mustard. Each value is the mean ± standard error of four replicates (n = 4). The values were compared through the least significant difference (LSD) test within the unblanched and blanched groups, respectively. Values in each group not sharing a common letter are significantly different at p < 0.05. DW: dry weight. | |
PCA
Many previous studies have used PCA to comprehensively evaluate the quality of various horticultural crops.3,35,36 In this study, PCA was used to gain an overall understanding of the variation in the quality of frozen baby mustard stemming from the different thawing treatments. PCA results showed that the first two extracted components (PC1 and PC2) explained 71% of the variation (Fig. 5A). Loadings results indicated that PC1 was positively associated with all of the measured indicators, while PC2 was positively associated with pigments, antioxidants, and antioxidant capacities, and negatively associated with glucosinolates (Fig. 5B).
 |
| Fig. 5 PCA analysis of the responses of nutritional qualities in baby mustard under different thawing methods. (A) Score plot; (B) loading plot. | |
Frozen vegetables suffer inevitable quality deterioration along with the thawing process.12–14 In this study, the points in PCA score plot representing different thawing treatments and blanching status before freezing were clearly separated (Fig. 5A), and closer distances from the control corresponded to lower quality degradation of lateral buds compared with the control.2 Unblanched baby mustard samples under W − B and MIC were closer to their corresponding controls compared with samples of the other treatments, indicating that the W − B and MIC better retained the quality of unblanched baby mustard. By contrast, blanched baby mustard samples under REF and MIC were closer to their corresponding controls compared with samples of the other treatments, indicating that REF and MIC better retained the quality of blanched baby mustard. In addition, MIC greatly reduced the thawing time of frozen vegetables (Table 1). Therefore, MIC was the optimal thawing method for frozen baby mustard, as it maximized nutritional quality and reduced the thawing time.
Conclusions
The modifications in health-promoting compounds and antioxidant capacity of frozen baby mustard greatly varied among the various thawing methods. W − B and MIC better retained the overall nutritional quality of unblanched baby mustard compared with other treatments, whereas REF and MIC better retained the overall nutritional quality of blanched baby mustard compared with other treatments. In addition, the thawing time was greatly shortened by MIC. Furthermore, blanched treatment resulted in lower losses of glucosinolates, chlorophylls, carotenoids and ascorbic acid contents compared with the unblanched treatment during thawing process. Thus, MIC was the optimal thawing method for frozen baby mustard regardless of whether it was blanched.
Conflicts of interest
The authors declare no conflict of interest.
Acknowledgements
The authors thank Dr Li-Qiang Tan from College of Horticulture, Sichuan Agricultural University (Chengdu, China) for helpful suggestions on statistical analysis. This work was supported by National Natural Science Foundation of China (32072586, 31500247), Project of New Varieties Breeding of Sichuan Vegetable Innovation Team (sccxtd-2020-05), and Technology Project of Zhishi Supply Chain Technology Co., Ltd. (2020008).
References
- B. Sun, Y. X. Tian, M. Jiang, Q. Yuan, Q. Chen, Y. Zhang, Y. Luo, F. Zhang and H. R. Tang, RSC Adv., 2018, 8, 33845–33854 RSC.
- B. Sun, P. X. Lin, P. X. Xia, H. M. Di, J. Q. Zhang, C. L. Zhang and F. Zhang, RSC Adv., 2020, 10, 36495–36503 RSC.
- B. Sun, H. M. Di, J. Q. Zhang, P. X. Xia, W. L. Huang, Y. Jian, C. L. Zhang and F. Zhang, Food Chem., 2021, 339, 128057 CrossRef CAS PubMed.
- A. Rybarczyk-Plonska, S. F. Hagen, G. I. A. Borge, G. B. Bengtsson, M. K. Hansen and A. Wold, Postharvest Biol. Technol., 2016, 116, 16–25 CrossRef CAS.
- R. Klopsch, K. Witzel, A. Börner, M. Schreiner and F. S. Hanschen, Food Res. Int., 2017, 100, 392–403 CrossRef CAS PubMed.
- Z. L. Xiao, G. E. Lester, Y. G. Luo, Z. H. Xie, L. L. Yu and Q. Wang, Food Chem., 2014, 151, 472–479 CrossRef CAS PubMed.
- F. Galgano, F. Favati, M. C. Caruso, A. Pietrafesa and S. Natella, J. Food Sci., 2007, 72, 130–135 CrossRef PubMed.
- W. Kmiecik, Z. Lisiewska and A. Korus, Eur. Food Res. Technol., 2007, 224, 573–579 CrossRef CAS.
- E. M. Gonçalves, M. Abreu, T. R. S. Brandão and C. L. M. Silva, Int. J. Refrig., 2011, 34, 2136–2144 CrossRef.
- H. Y. Miao, J. Y. Lin, W. Zeng, M. Y. Wang, L. S. Yao and Q. M. Wang, Foods, 2019, 8, 375 CrossRef CAS PubMed.
- N. Pupan, P. Dhamvithee, A. Jangchud and S. Boonbumrung, J. Food Process. Preserv., 2018, 47, e13669 CrossRef.
- B. Li and D. W. Sun, J. Food Eng., 2002, 54, 175–182 CrossRef.
- D. Villarreal-García, P. A. Alanís-Garza, M. D. R. Cuéllar-Villarreal, M. Redondo-Gil, J. L. Mora-Nieves and D. A. Jacobo-Velázquez, CyTA--J. Food, 2015, 13, 312–320 CrossRef.
- X. Wen, R. Hu, J. H. Zhao, Y. Peng and Y. Y. Ni, Int. J. Food Sci. Technol., 2015, 50, 1116–1122 CrossRef CAS.
- L. Y. Cai, M. J. Cao, J. Regenstein and A. Cao, Compr. Rev. Food Sci. Food Saf., 2019, 18, 953–970 CrossRef PubMed.
- B. G. Xu, J. N. Chen, J. Yuan, S. M. R. Azam and M. Zhang, J. Sci. Food Agric., 2017, 219, 118–124 Search PubMed.
- R. L. Prior, E. Fan, H. Ji, A. Howell, C. Nio, M. J. Payne and J. Reed, J. Sci. Food Agric., 2010, 90, 1473–1478 CrossRef CAS PubMed.
- I. F. Benzie and J. J. Strain, Anal. Biochem., 1996, 239, 70–76 CrossRef CAS PubMed.
- T. Li, J. W. Lee, L. Luo, J. Kim and B. K. Moon, Appl. Biol. Chem., 2018, 61, 257–265 CrossRef.
- A. J. Virtanen, D. L. Goedeken and C. H. Tong, J. Food Sci., 1997, 62, 150–154 CrossRef CAS.
- S. Phothiset and S. Charoenrein, J. Sci. Food Agric., 2013, 94, 189–196 CrossRef PubMed.
- M. Francisco, R. Velasco, R. A. Moreno, R. Garcia-Viguera and R. E. Cartea, Food Res. Int., 2010, 43, 1455–1463 CrossRef CAS.
- X. Y. Tan, A. Misran, L. D. J. Daim, P. Ding and M. S. P. Dek, Sci. Hortic., 2020, 264, 109170 CrossRef CAS.
- H. Dong, Y. Jiang, Y. Wang, R. Liu and H. Guan, Food Technol. Biotechnol., 2004, 42, 135–139 Search PubMed.
- C. X. Cai, H. Y. Miao, H. M. Qian, L. S. Yao, B. L. Wang and Q. M. Wang, Food Chem., 2016, 210, 451–456 CrossRef CAS PubMed.
- W. J. Lessin and S. J. Schwartz, J. Agric. Food Chem., 1997, 45, 3728–3732 CrossRef CAS.
- L. Zhan, J. Hu, Y. Li and L. Pang, Postharvest Biol. Technol., 2012, 72, 76–81 CrossRef CAS.
- A. Hartmann, P. Claus-Dieter, W. Andlauer, H. Dietrich and M. Ludwig, J. Agric. Food Chem., 2008, 56, 9484–9489 CrossRef CAS PubMed.
- M. Holzwarth, S. Korhummel, R. Carle and D. R. Kammerer, Food Res. Int., 2012, 48, 241–248 CrossRef CAS.
- T. O. Idowu, A. O. Ogundaini, A. O. Salau, E. M. Obuotor, M. Bezabih and B. M. Abegaz, Phytochemistry, 2010, 71, 2092–2098 CrossRef CAS PubMed.
- N. Girgin and S. N. El, J. Food Compos. Anal., 2015, 37, 119–127 CrossRef CAS.
- A. C. Martins, L. Bukman, A. M. M. Vargas, E. O. Barizăo, J. C. G. Moraes, J. V. Visentainer and V. C. Almeida, Food Chem., 2013, 138, 574–580 CrossRef CAS PubMed.
- K. Sridhar and A. L. Charles, Food Chem., 2019, 275, 41–49 CrossRef CAS PubMed.
- M. Shiraishi, R. Shinomiya and H. Chijiwa, Sci. Hortic., 2018, 227, 272–277 CrossRef CAS.
- C. Chen, H. Zhang, X. Zhang, C. Dong and W. Xu, Postharvest Biol. Technol., 2020, 163, 111124 CrossRef CAS.
- P. D. C. Sanchez, N. Hashim, R. Shamsudin and M. Z. M. Nor, Postharvest Biol. Technol., 2020, 164, 111163 CrossRef CAS.
Footnotes |
† Electronic supplementary information (ESI) available. See DOI: 10.1039/d1ra00610j |
‡ These authors contributed equally to this work. |
|
This journal is © The Royal Society of Chemistry 2021 |
Click here to see how this site uses Cookies. View our privacy policy here.